Introduction
Milk is regarded as a valuable source of bioactive compounds. It contains many biologically active substances with antioxidant properties. Antioxidants largely limit or even prevent oxidative processes in the living organism. By scavenging and neutralizing reactive oxygen species, they play an important role in the support and enhancement of organism defence mechanisms, which is particularly important in the prophylaxis of some lifestyle diseases (Lisak Jakopović et al., 2019; Stobiecka et al., 2022). Such components are present in protein (β-lactoglobulin, lactoferrin), fat (vitamin E, A, β-carotene), and water (vitamin C, microelements: Sn, Zn, Fe, Mn) fractions (Puppel et al., 2015; Król et al., 2017; Vanitcharoen et al., 2018). It should be emphasized that whey proteins, especially β-lactoglobulin, have the highest antioxidant potential of all proteins in the human diet. This is related to the high content of sulphur containing amino acids, in particular cysteine, which is essential for glutathione synthesis (Ma et al., 2018). As reported by Kim et al. (2019), in addition to β-lactoglobulin, the antioxidant effect is also exhibited by α-casein. Due to their antioxidant properties, these proteins can inhibit cell aging and increase myoblast differentiation and maturation, thereby alleviating aging-related damage caused by oxidative stress. Antioxidant activity is also exhibited by lactoferrin, as it chelates iron and thus increases its bioavailability and inhibits the pro-oxidative effect of this element. However, the antioxidant capacity of LF declines proportionally to iron saturation (Cutone et al., 2020). Proteins and their fractions are valuable sources of bioactive peptides with an ability to scavenge free radicals, chelate metal ions, and inhibit lipid peroxidation (Bielecka et al., 2021). Fat-soluble vitamins, mainly vitamin E and especially α-tocopherol as well as vitamin A and β-carotene, are the main antioxidants in this group of compounds (Celi, 2011; Kaneai et al., 2012; Vanitcharoen et al., 2018). Their activity is based on scavenging organic free radicals and inhibiting lipid peroxidation (Mann et al., 2016). They also have the ability to absorb singlet oxygen and hydroxyl radicals, thus effectively preventing DNA oxidation (Cichosz et al., 2017). Since they are present in fat globule membranes, they prevent milk fat autoxidation. Similarly, vitamin D 3 is part of the non-enzymatic antioxidant system in milk, and 1,25-dihydroxycholecalciferol is the most active form. Its antioxidant effect is based on the inhibition of lipid peroxidation (Mutlu et al., 2013). Vitamin C (ascorbic acid) plays an important antioxidant role in the organism, by being a non-enzymatic water-soluble antioxidant, and as well as B group vitamins by inhibiting homocysteine production. Hence, the antioxidant potential of milk is directly associated with the content of compounds exhibiting antioxidant properties (Stobiecka et al., 2022).
The global dairy production, including Poland, is based primarily on Holstein-Friesian cows. The cows of this breed are characterized by high milk yields but also by the highest susceptibility to metabolic stress. As shown by some previous research findings, reactive oxygen species are produced during various physiological processes, e.g. during the lactation period. The animal organism utilizes antioxidants to reduce free radicals (Chang et al., 2007). An excess of free radicals results is damage to macromolecules, i.e. proteins, lipids, and DNA, leading to metabolic disorders, lower milk yields, or deterioration of milk quality with reduction in the content of antioxidant substances (Karasahin et al., 2021).
The aim of the study was to assess the content of components exhibiting antioxidant activity in the milk of Holstein-Friesian cows and changes in the total antioxidant status during subsequent lactations and their stages.
Material and methods
The study was carried out on a Holstein-Friesian cattle farm with an intensive milk production system. The cows were kept in a free-stall barn and their nutrition was based on the total mixed ration (TMR) system throughout the year. The feed ration was composed of roughage (maize silage, grass haylage) and concentrate fodder. The study involved 90 Holstein-Friesian cows, i.e. 30 in each lactation group (I - primiparous cows; II - multiparous cows in lactation II; III - multiparous cows in lactation III). Milk samples were collected from each cow in three terms: 1. before lactation day 100, 2. between lactation days 101 and 200, and 3. between lactation days 201 and 305. Chilled samples were transported to the laboratory where the somatic cell count (SCC) was determined using a Somacount150 device (Bentley Instruments, USA). Milk samples with the somatic cell count not exceeding 400 thousand/ml were taken for further analysis. In total, 262 milk samples were analysed. The following parameters were determined in each milk sample: the basic chemical composition, i.e. the content of total protein, fat, lactose, and dry matter (using the Infrared Milk Analyzer; Bentley Instruments, USA), the content of casein (according to AOAC (2000)), and the concentration of selected whey proteins, i.e. α-lactalbumin (α-LA), β-lactoglobulin (β-LG), lactoferrin, and bovine serum albumin (BSA), using reversed phase high-performance liquid chromatography. All samples for the whey protein determinations were prepared as in Romero et al. (1996) with modifications (Brodziak et al., 2012). Protein separation was performed using a ProStar 210 liquid chromatograph equipped with a UV-Vis ProStar 325 detector (Varian, USA). In all cases, the separations were carried out in the water-acetonitrile gradient mobile phase and NUCLEOSIL 300-5 C18 columns with 250-mm length and 4.6-mm diameter (Varian, USA). The mobile phase consisted of solvent A (90 % water, 10 % acetonitrile) and solvent B (90 % acetonitrile, 10 % water) (Sigma, Germany). A single sample was analysed for 35 min at a wavelength of λ = 205 nm and a column temperature of 37 °C. The standards were analysed in identical conditions. To this end, standard solutions of purified proteins were used, i.e. α-LA (≥85 %), β-LG (90 %), BSA (≥96 %), and lactoferrin (90 %); they all were obtained from milk proteins (Sigma, Germany). Based on the analysis of the retention times read from individual chromatograms, the qualitative identification of individual substances was performed with the use of the Star 6.2 Chromatography Workstation program (Varian, USA). The quantitative analysis was performed with the external standard method. The RP-HPLC method was used to determine the concentration of fat-soluble vitamins, i.e. A, D 3, and E. All samples were prepared with the Röse-Gottlieb fat extraction method modified by Hewavitharan et al. (1996). The separations were performed using a PursuitXRs 3-C18 column with 150-mm length and 4.6-mm diameter (Varian, USA). The phase was a mixture of acetonitrile, methanol, water, and dichloromethane (Sigma, Germany), and the flow rate was set to 1 mL/min. The standards were analysed in identical conditions. The following standard vitamin solutions were used: (±)-α-tocopherol (vitamin E) with ≥97 % purity (HPLC), cholecalciferol (vitamin D 3) - ≥98 % (HPLC), retinol (vitamin A) - ≥99 %, and β-carotene (provitamin A) - ≥98 % (HPLC) (Sigma, Germany). The qualitative identification of individual substances was based on the analysis of the retention times read from individual chromatograms using the Star 6.2 Chromatography Workstation program (Varian, USA). The quantitative analysis was carried out using the external standard method.
The total antioxidant status (TAS) was determined using the Randox tests (Tecan Austria GmbH, Grödig, Austria). The analysis consisted in spectrophotometric measurements of the degree of colour change of the resulting reactive radical ABTS® (2,2’-azino-di-[3-ethylbenzothiazoline sulfonate) using a UV-Vis spectrophotometer at a wavelength of 600 nm. ABTS® was incubated with peroxidase (metmyoglobin) and H 2O 2 to obtain the ABTS® + radical cation. It is characterized by a relatively stable blue-green colour, which was measured at the aforementioned wavelength. The antioxidants present in the added sample led to weakening of the blue-green color in proportion to their concentration.
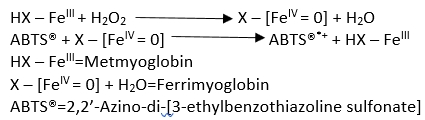
The results were analysed statistically using a one-way analysis of variance in StatSoft Inc. Statistica ver. 13. Significant differences between the means were determined using Tukey's test at a significance level p (alpha) = 0.05 and 0.01. Additionally, Pearson linear correlation coefficients for the milk yield and parameters were calculated.
Results and discussion
Table 1 shows the daily milk yield (on the sampling day), SCC, and the content of basic milk components relative to the subsequent lactations and their stage. A significant (p≤0.01) increase in the milk yield was recorded during the subsequent lactations. The daily amount of milk produced by cows in lactation III was by 5 kg higher in comparison to the group of cows in lactation II and by 10 kg higher than in the group of primiparous cows. An increase in the somatic cell count was also noted with the increasing age of the cows. Since the somatic cell count in the analysed milk did not exceed 400 thousand/mL, it can be assumed that the permeability of the mammary gland epithelium increased with the age of the cows. This was most probably associated with earlier inflammations, which damaged the epithelium and increased its permeability even after healing (Liu et al., 2009; Litwińczuk et al., 2011). Król et al. (2014) found significant interactions (p≤0.01) between the subsequent lactations and SCC. In turn, a downward tendency in the total protein content accompanied by an increase in the fat level was observed in the subsequent lactations. Similar relationships were confirmed by other authors as well (Kuczyńska et al., 2021). The amount of produced milk decreased significantly (p≤0.01) in the consecutive lactation stages. The highest yield was recorded in the first stage of lactation. However, the milk from this stage exhibited the lowest dry matter content, including the total protein and fat. During the subsequent lactation stages, the proportion of dry matter components (p≤0.05) in the milk, e.g., the content of protein (p≤0.05) and fat (p≤0.01), increased significantly. Studies conducted by other authors (Pecka et al., 2012; Król et al., 2013; Kuczyńska et al., 2021) reported a systematic decrease in the cow milk yield accompanied by an increase in dry matter content, including fat and protein, during consecutive lactation stages.
Table 1. Cow milk yield and the basic chemical composition of milk in the subsequent lactations and the stages of lactation
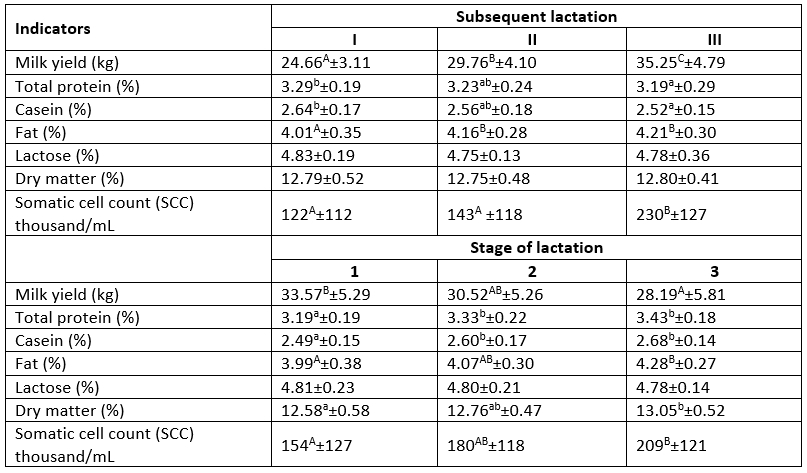
a, b - significant differences at p≤0.05; A, B, C - significant differences at p≤0.01
Table 2 shows the content of whey proteins in milk, which in most cases have antioxidant activity. The content of albumins, i.e. α-LA and β-LG, decreased and significant differences were observed between lactations I and III. The differences were 0.10 g/L (p≤0.05) in the case of α-LA and 0.18 g/L (p≤0.01) in the case of β-LG. Similar trends were noted along the lactation stages, with significant differences only in the α-LA content between the first and third stages (p≤0.05). The decline in the content of α-LA may have been caused by the decrease in the milk yield at the end of lactation, as this protein is one of the main components of the lactose synthase complex involved in the control of lactation and milk secretion (Liu et al., 2007). Similar findings were reported by other authors (Heck et al., 2009; Król et al., 2013). In the case of BSA, i.e. another milk albumin, there were different relationships, i.e. its amount increased significantly (p≤0.05) with the age of the cows. As suggested by Litwińczuk et al. (2011), the BSA concentration is an indicator of the permeability of the blood-milk barrier in the mammary gland. Mastitis is the main factor increasing the permeability. Since the SCC value in the present study did not exceed 400 000/mL, it can be assumed that the age of the cows had an impact on the permeability of the mammary gland epithelium as well. It was most probably associated with previous inflammation, which caused damage to the epithelium and increased its permeability even after healing. However, there was no effect of the lactation stage on the content of this protein in the milk. The subsequent lactations were accompanied by an increase in the concentration of the main antibacterial proteins, i.e. lactoferrin and lysozyme. There were significant (p≤0.05) differences (at a level of approximately 20 mgL) in the lactoferrin content between lactation I and III. Other authors reported significant differences in the content of lactoferrin in subsequent lactations as well (Hagiwara et al., 2003; Back and Thompson, 2005; Król et al., 2013). The content of lactoferrin and lysozyme increased with the progression of lactation, with significant (p≤0.05) differences in the lactoferrin level. Its content in the third lactation stage increased by over 25 mg/L, which corresponded to approximately 1/4 of its level in the first stage. A significant increase in the lactoferrin concentration in milk along lactation stages was demonstrated by Cheng et al. (2008), who reported a high correlation coefficient between the content of lactoferrin and the stage of lactation (r=0.557). The authors also found significant (p<0.01) negative correlations between the lactoferrin concentration and daily milk yield and significant (p<0.018) relationships between the lactation stage and milk yield. These correlations were confirmed in the present study. An increasing concentration of lactoferrin during the lactation stages was noted in the milk of cows characterised by a lower daily milk yield.
Table 2. Content of whey proteins in the milk in the subsequent lactations and the stages of lactation
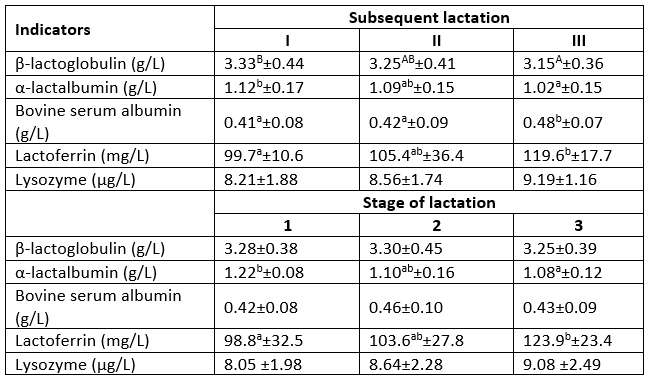
a, b - significant differences at p≤0.05; A, B - significant differences at p≤0.01
The results presented in Table 3 show a significant (p≤0.01) effect of the subsequent lactations on the content of lipophilic vitamins with antioxidant activity, i.e. A and E. The milk from the primiparous cows exhibited significantly higher contents of these vitamins, compared with the milk from lactation III of the multiparous cows. The milk produced by the cows in lactation I was characterized by on average 20 % and 15 % higher content of vitamins E and A, respectively, compared to the milk from the multiparous cows. With the progression of lactation, the content of the analysed vitamins (A, E, D 3) decreased, but statistically significant differences (p≤0.05) were found only in the case of vitamin A. In comparison with the first lactation stage, the content of this vitamin in the third stage decreased by almost 30 %. Kapusta et al. (2018) reported a higher level of vitamin E at the peak of the first lactation stage, i.e. between day 29 and 70. The results of a study conducted by Strusińska et al. (2010) indicated changes in the concentration of vitamins in milk analysed in different stages of lactation; however, as emphasized by the authors, the obtained differences were largely related to the nutrition provided to the cows. During the winter period (November-March), the highest concentrations of vitamin A and E in the milk were found between lactation day 121 and 180 and for between day 61 and 120 day, respectively, but the differences were not statistically significant. The pasture-derived feed in the ration resulted in a gradual increase in the vitamin E concentration with progression of lactation. During this period, the vitamin A content in milk was higher on the first 60 days after calving. In turn, in a study of organic milk conducted by Sakowski et al. (2012), the content of vitamins A and E in milk increased significantly during the subsequent stages of lactation. The authors found the highest level of these vitamins in the fourth stage of lactation (after 250 days post calving). On contrary, Navrátilová et al. (2019) did not find significant differences in the content of vitamins A and E in the milk of lactating mares. As reported by Calderón et al. (2007), the secretion of vitamins A and E to milk is variable and depends on the lactation stage only to a limited extent. The energy balance in cows has an impact on the concentration of vitamins A and E in milk (Noziére et al., 2006).
As demonstrated by Dolores-Perez and Calvo (1995), the vitamin A content in milk is positively correlated with the concentration of β-LG, as this protein is actively involved in the transport of vitamin A. Similarly, other authors (Kuczyńska et al., 2011; Brodziak et al., 2018) indicated relationships between the content of these compounds in milk. This was also confirmed by the results of the present study, which showed statistically significant (p≤0.01) positive correlations between the content of vitamin A and β-LG, with a high correlation coefficient – r=0.613 (Table 4).
Table 3. Content of lipophilic vitamins in the milk in the subsequent lactations and the stages of lactation
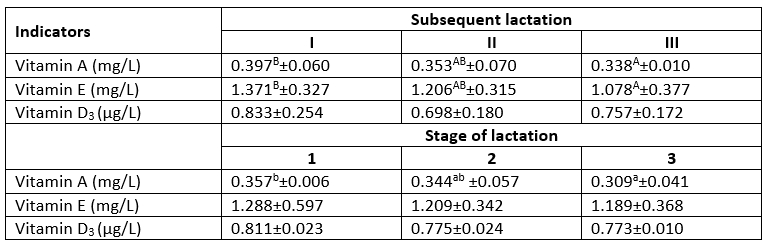
A, B - significant differences at p≤0.01
Significant (p≤0.01) positive correlation coefficients of the content of vitamin A, vitamin E, and β-LG with the total antioxidant status of milk were obtained (Table 4), which indicates that the content of these compounds largely determines the antioxidant potential of the milk. The highest values of the coefficients were recorded for the correlation between the total antioxidant status and the content of vitamin A (r=0.687) and E (r=0.664), and slightly lower values were noted for the correlation with β-LG (r=0.515). As reported by other authors (Puppel et al., 2017; Kapusta et al., 2018), milk with higher contents of vitamins A and E exhibited higher antioxidant potential.
Table 4. Pearson correlation coefficients for daily yield and milk parameters
*p≤0.05; **p≤0.01
The present results indicate that the total antioxidant status of the milk changed during the subsequent lactations (Fig. 1a) and their stages (Fig. 1b). The highest TAS was exhibited by the milk from primiparous cows (0.96 mmol/L), which was most probably associated with the higher content of components with antioxidant properties in the milk, i.e. vitamins A and E as well as β-LG. The total antioxidant status of the milk from cows in lactation II was slightly lower (0.92 mmol/L). In turn, a significant (p≤0.01) decrease (by approx. 20 %) in the value of the antioxidant potential of milk and in the content of components with antioxidant properties was noted in the lactation III period. These relationships are also indicated by the positive correlation coefficients between TAS of milk and the content of vitamins A, E, and β-LG (Table 4). Noteworthy, TAS of the milk was significantly (p≤0.05) negatively correlated with the milk yield (r=-0.317). The subsequent lactations were accompanied by a significant increase in the milk yield and a decrease in the antioxidant status of the milk. Similar relationships were reported by Kapusta et al. (2018), who obtained a significant (p≤0.01) correlation coefficient (r=-0.390) between the milk yield and total antioxidant status in a study of multiparous cows at the peak of lactation. As suggested by other authors (Sakowski et al., 2012; Kuczyńska et al., 2021), a higher milk yield can increase the risk of oxidative stress, which has an impact on the health of cows and the chemical composition and quality of milk. In a study conducted by Puppel et al. (2012), it was found that the antioxidant capacity of milk may be related to dietary supplementation and the age of cows. Addition of fish oil and linseed to cow rations significantly increased the total antioxidant status of milk, with a higher level in the milk from primiparous cows in both nutrition groups. Mann et al. (2016) highlighted the effect of the lactation stage on changes in the antioxidant status of milk. The authors determined the status of milk produced by different breeds of cows (Sahiwal, Karan Fries, and Holstein Frisian) and found higher values in the case of colostrum and milk collected in the early stage of lactation. In the later stages, the antioxidant potential values declined significantly. The authors suggest that elevated levels of antioxidants in colostrum may be crucial for the protection of the health of new-born calves against oxidative stress. The present study demonstrated a decrease in the total antioxidant status of the analysed milk in the consecutive stages of lactation, with significant differences (p≤0.05) between the first and third stages (Fig. 1). Significant changes in the antioxidant potential of milk during lactation were also reported by Annie et al. (2019), who showed the highest antioxidant potential of milk obtained in the early stage of lactation. The investigations conducted by Kapusta et al. (2018) also revealed a clear relationship between the lactation stage and the level of antioxidants in milk. The highest total antioxidant status was determined by the authors on the first days of lactation, but its level gradually decreased on the following days.
a)
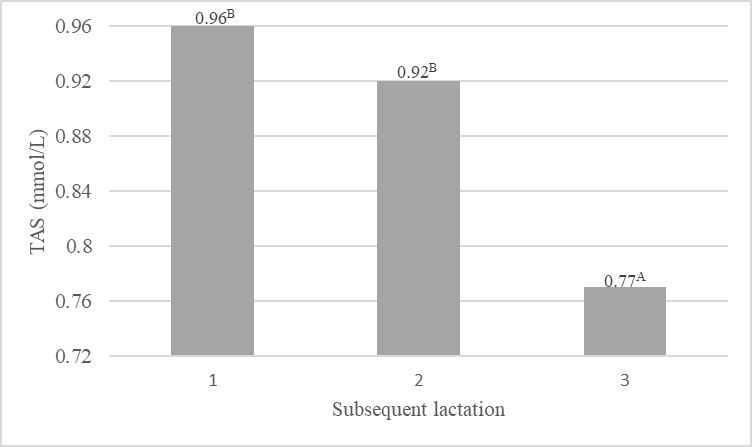
b)
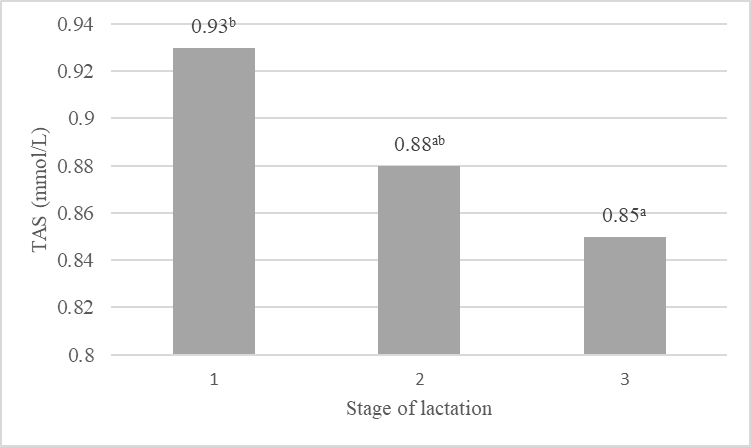
A, B - significant differences p≤0.01; a, b - significant differences p≤0.05
Figure 1. Value of the total antioxidant status (TAS, mmol/L) in the subsequent lactations and their stages
Conclusions
The study showed that the content of antioxidant components, i.e. vitamins A and E and albumin (β-LG and α-LA), decreased significantly during the subsequent lactations. At the same time, a significant decrease in the value of the total antioxidant status of milk was noted. The lactation stage had a lesser effect on the antioxidant potential of milk. The values of the correlation coefficients between the total antioxidant status level and the content of vitamins A and E as well as β-LG indicate that the presence of these compounds largely determines the antioxidant potential of milk. Additionally, there were negative correlations between the level of total antioxidant status and the daily milk yield, which suggests that high yields of milk have a negative impact on its antioxidant potential.
Funding
This paper was funded through the “Regional Initiative of Excellence” in 2019-2022, project
number 029/RID/2018/19, funding amount: PLN 11,927,330.00.
Antioksidacijski potencijal mlijeka holštajn-frizijskih krava s obzirom na stadij i redoslijed laktacije
Sažetak
Cilj istraživanja bio je utvrditi koncentraciju sastojaka koji pokazuju antioksidativno djelovanje u mlijeku holštajn-frizisjkih krava i promjene ukupnog antioksidacijskog statusa tijekom stadija i redoslijeda laktacije. U svrhu istraživanja korišteni su uzorci mlijeka prikupljeni od 90 krava (po 30 za svaki analizirani laktacijski broj: I - prvotelke; II - višetelke u drugoj laktaciji; III - višetelke u trećoj laktaciji) u tri razdoblja: 1 - do 100 dana laktacije, 2 - 101-200 dana laktacije, a 3 - 201-305 dana laktacije. U mlijeku je određen osnovni kemijski sastav, broj somatskih stanica, udio kazeina, odabranih proteina sirutke i vitamina topljivi u mastima. Također je izmjeren i ukupni antioksidacijski status (TAS) mlijeka. S obzirom na redoslijed laktacije značajno se smanjivala (p≤0,01) koncentracija sastojaka s antioksidacijskim svojstvima, tj. vitamina A i E, te albumina (α-laktalbumin i β-laktoglobulin). Istovremeno je zabilježen i pad vrijednosti TAS u mlijeku. Stadij laktacije imao je manji učinak na antioksidacijski potencijal mlijeka. Visoki koeficijenti korelacije između vrijednosti TAS i koncentracije vitamina A, E i β-laktoglobulina ukazuju da udio ovih spojeva uvelike određuje antioksidacijski potencijal mlijeka. S druge strane, negativne korelacije dobivene između vrijednosti antioksidacijskog potencijala i dnevnog prinosa mlijeka (r=-0,347, p≤0,05) upućuju na to da visoka proizvodnja mlijeka negativno utječe na antioksidacijsku vrijednost mlijeka.
Ključne riječi: mlijeko; vitamini; bjelančevine; antioksidacijski potencijal; laktacija