Introduction
Patients with chronic heart failure (CHF) often have a poor prognosis as they have similar rates of first-time hospitalization and long-term survival as patients with common forms of cancer (1). In the treatment of CHF, angiotensin-converting enzyme (ACE) inhibitors are recommended for all patients with reduced ejection fraction to reduce the risk of hospitalisation and death (2). According to the guidelines, the dose of ACE inhibitors should be gradually titrated up to the maximum tolerated recommended dose to ensure adequate inhibition of the renin-angiotensin-aldosterone system and thus better outcomes (2–4).
Ramipril is one of the ACE inhibitors that can be used in patients with CHF. It is a rather complex drug in terms of its pharmacokinetics and pharmacodynamics. Due to its rapid absorption after oral administration, the maximum plasma concentration of ramipril is reached between 0.5 and 1 hour (5, 6). Ramipril is a prodrug that is metabolised by esterases in the liver and kidneys to the active ACE inhibitor ramiprilat. A number of other metabolites are formed in the liver, whereas in the kidneys, ramipril is metabolised to ramiprilat (7). ACE inhibitors have a very high affinity for the ACE enzyme, which is mainly found in tissue, but about 10 % is also present in plasma. The ACE enzyme has two independent binding sites with different binding constants. Due to the saturation of the binding sites, non-linear kinetics are to be expected (7). Ramipril is excreted in the urine to a much lesser extent than ramiprilat, so excretion is not influenced by renal function, which, however, has an important influence on the clearance of ramiprilat (5, 7–9). Ramiprilat is predominantly excreted in the kidneys by glomerular filtration and tubular secretion (8). Ramipril and ramiprilat are also partially excreted via the bile (10).
Previous pharmacokinetic studies showed that heart failure patients had higher plasma concentrations of ramipril and ramiprilat and a prolonged plasma half-life compared to healthy adults (11, 12). However, a population pharmacokinetic model has not yet been developed to further investigate and quantify these differences. No previous study has focused on the changes in pharmacokinetics that may occur in patients after prolonged drug therapy. Between 5 % and 15 % of CHF patients develop cachexia (13). Therefore, it is particularly important to know whether changes in body composition are associated with changes in pharmacokinetics in CHF patients (14, 15).
The aim of this study was to develop a population pharmacokinetic model of ramipril in patients with CHF to evaluate the influence of body composition on the pharmacokinetics of ramipril. In addition, longitudinal changes in the pharmacokinetic parameters of ramipril and ramiprilat in patients under chronic ramipril therapy were to be evaluated.
Experimental
Clinical studyThe clinical study was approved by the National Medical Ethics Committee of the Republic of Slovenia (approval number 114/10/11) and is registered at ClinicalTrials.gov (NCT01829880). The study was conducted in accordance with the ICH guidelines for good clinical practice, institutional and national ethical standards, and the Declaration of Helsinki and its revisions.
Patients with CHF class I–III according to the New York Heart Association functional classification were included in the study. They had to be on stable regular therapy with ramipril and their dosing regimen was not to be changed for at least four weeks prior to each study visit. The four-week therapy allows the determination of ramipril and ramiprilat plasma concentrations at steady state. Patients with known liver disease or acute worsening of heart failure requiring hospitalisation or a change in pharmacotherapy were excluded from the study. Patients were invited to participate in the study during their regular visits to the outpatient clinic of the University Clinic of Pulmonary and Allergic Diseases Golnik, Slovenia. After verbal and written information, consent was obtained from all patients included in the study.
The study comprised two visits to the outpatient clinic (one initial and one follow-up visit), at least 6 months apart. At both study visits, the ramipril dosing regimen and the exact time of the last ramipril dose were documented for each patient. Adherence to ramipril therapy was assessed by a physician and a pharmacist, and only patients with good adherence were included in the study. At each study visit, plasma concentrations of ramipril and ramiprilat were measured after ramipril dosing, and patients’ body composition was determined. Patients came to the study in the morning, fasted, and took their morning dose of ramipril in the clinic. They also fasted for at least 1 hour after the dose. According to the protocol, blood samples were to be taken shortly before the ramipril dose (trough sample) and 1, 2, 3, 4, and 5 hours after the dose. A total of 195 blood samples were taken from the patients, at a minimum of three time points per patient visit.
Biochemical and clinical parameters
Serum creatinine concentration was measured in blood samples to determine creatinine clearance using the Modification of Diet in Renal Disease (MDRD4) four-variable equation. The following parameters were determined to assess liver function: direct bilirubin, total bilirubin, serum aspartate transaminase (AST), and serum albumin. Left ventricular ejection fraction was determined by echocardiography using the biplane Simpson method.
Assessment of body composition
At both study visits, body composition was determined using dual-energy X-ray absorptiometry (DEXA) (Hologic Explorer, QDR Series, Hologic Inc., USA) and bioelectrical impedance analysis (BIA) (Bodystat 1500 analyser, Bodystat Ltd, UK). DEXA whole-body scans were used to determine fat mass, lean mass, and body mineral content. BIA was used to assess fat mass, lean mass, dry lean mass, and water content. The BIA was performed on fasting patients after a 10-minute rest period in a supine position. One patient with a pacemaker did not undergo BIA on either of the two study days.
Fat-free mass ( FFM) was measured directly using the DEXA method. However, in one patient at baseline and two patients at the follow-up DEXA scans were not recorded because the device was not available at the time of the examination. In these cases, the FFM value was calculated using the water content determined by BIA (16). In addition to actual body weight ( WT) and FFM, body mass can also be described by normal fat mass ( NFM), i.e. body mass at normal fat percentage calculated using Eq. 1, where Ffat is the estimated parameter to account for the contribution of fat mass (17).
( 1)
To evaluate the influence of body composition on the pharmacokinetics of ramipril, a fat-free mass index ( FFMI) and a skeletal muscle index ( SMI) were also calculated using Eqs. 2 and 3 (18) and skeletal muscle mass was calculated using the BIA equation of Janssen et al. (19).
( 2)
( 3)
Determination of ramipril and ramiprilat in plasma samples
Ramipril and ramiprilat were measured in plasma by liquid chromatography with tandem mass spectrometry. Plasma samples (500 µL) were mixed with stable D5-labeled internal standards for both analytes and extracted with mixed-mode solid phase extraction cartridges 60 mg/3 mL Strata X-C (Phenomenex, USA). The extracts were dried and reconstituted in a smaller volume of solvent containing 25 % methanol (200 µL) before being injected (1 µL) into Agilent 1290 Infinity ultra-high-performance liquid chromatograph coupled to the Agilent 6460 triple quadrupole mass spectrometer (Agilent Technologies, USA). Analytes were separated on a 50 mm × 2.1 mm C18 Kinetex column with 2.6 µm particles (Phenomenex, USA) using a mobile phase containing 0.1 % formic acid in water (A) and (B) acetonitrile at a flow rate of 0.5 mL min–1 and gradient elution (30–85 % B from 0.1–0.7 min). The mass spectrometer with electrospray ionization was operated in positive mode with multiple reaction monitoring at a collision energy of 15 eV using the following m/z transitions: 417.2 → 234.2 and 389.3→206.2 for ramipril and ramiprilat, respectively, and 422.3 → 239.3 and 394.3→211.2 for rampril-D5 and ramiprilat-D5, respectively. The total run time including the re-equilibration was 2.0 min. The linear range covered the concentrations 0.5–200 ng mL–1 with a limit of quantification of 0.5 ng mL–1 for both analytes. The average recovery was 99.7 % and 98.3 % for ramipril and ramiprilat, respectively. The matrix effect was tested by comparing the regression line slopes obtained by spiking plasma from 5 individual donors (20). The obtained RSD values of 4.6 % and 4.9 % RSD for ramipril and ramiprilat, respectively, show that there is no significant matrix interference (21). Short-term, freeze-thaw, and post-preparative stabilities were also tested and found to be adequate.
Pharmacokinetic modelling
The population pharmacokinetic model was developed using nonlinear mixed-effects modelling software (NONMEM®, version 7.5.1, Icon plc, USA). PsN (version 5.3.0, https://uupharmacometrics.github.io/PsN/) and Xpose (version 0.4.13, https://uupharmacometrics.github.io/xpose/) were used to support model development and evaluation.
Initial graphical exploration of the concentration-time data indicated two phases in the disposition of ramipril (parent drug). Therefore, the starting point for model development was a two-compartment model with first-order absorption and elimination for ramipril (ADVAN4, TRANS4). In the next step, we developed a parent-metabolite model assuming complete conversion of ramipril to ramiprilat. This way, we avoided the non-identifiability of metabolite pharmacokinetics. The parameter estimates from the first step were used as initial values. One- and two-compartment models for the disposition of ramiprilat (metabolite) were tested. The final analysis used a two-compartment model for ramipril and a one-compartment model for ramiprilat implementing ADVAN6 (Fig. 1). Assuming a log-normal distribution, between subject (BSV) and within the subject (WSV) variabilities were modelled using an exponential model. Additive, proportional, and combination models were tested for residual unexplained variability in plasma concentrations of ramipril and ramiprilat. The FOCE-I method was used to estimate the following pharmacokinetic parameters: absorption rate constant of ramipril ( Ka), volume of the central compartment for ramipril ( V1), volume of the peripheral compartment for ramipril ( V2), volume of distribution of ramiprilat ( Vm), distribution clearance of ramipril ( Q), clearance of ramipril ( CL) and clearance of ramiprilat ( CLm). Since the absolute bioavailability is not known, all clearances and distribution volumes are apparent, i.e. scaled by the bioavailability factor ( F). The parameters for the metabolite ( Vm and CLm) were additionally scaled by the fraction of ramipril that is metabolised to ramiprilat ( fm).
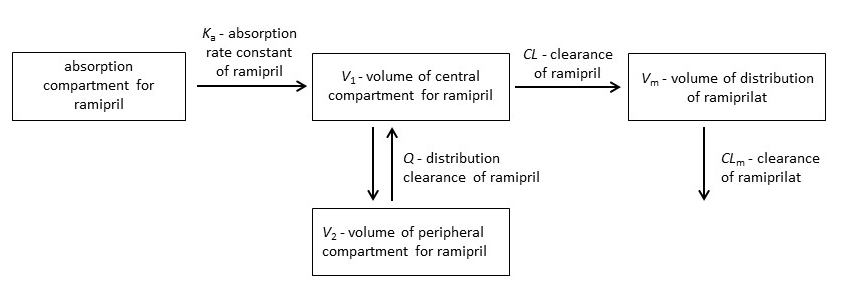
Fig. 1. The structural parent-metabolite pharmacokinetic model of ramipril and ramiprilat.
The covariate effects of patient age and sex, WT, FFM and NFM, creatinine clearance, liver markers (AST, albumin, conjugated and direct bilirubin), left ventricular ejection fraction, FFMI, SMI, and daily ramipril dose were first assessed by graphical exploration and then by a stepwise covariate modelling (SCM) procedure using PsN. In the first step, covariates associated with body size ( WT, FFM, and NFM) were tested using a power model. The exponents in the models adjusting for body size were both fixed values according to allometric theory (1 for volume terms, 0.75 for clearance terms) and estimated parameters. In the second step, the significance of the remaining covariates was tested within a model adjusted for body size using forward-backward SCM. Patient sex as a categorical covariate was coded to estimate the proportional difference. Linear and power models were investigated for continuous covariates. The significance of the covariate relationships was assessed using the likelihood ratio test ( p < 0.05 in the forward step, p < 0.01 in the backward step). Reduction of the unexplained between and within subject variabilities and biologically plausible parameter estimates were additional criteria for retaining a covariate in the model.
The models were evaluated using standard goodness-of-fit plots. Additional criteria included convergence of minimization, lack of substantial η- and ε-shrinkage, successful covariance steps, and gradients in the last iteration ranging from 10–3 to 102. Discrimination between models was based on the difference in the objective function value and the standard diagnostic plots. The final model was additionally evaluated by the bootstrap method with 1000 replicates of the original data set. To assess the simulation properties of the final model, a prediction-corrected visual predictive check was performed and the observed 5th, 50th, and 95th percentiles of ramipril and ramiprilat concentrations were compared with the 95 % confidence intervals of the simulations.
Experimental data were reported as median values with range. Wilcoxon signed-rank test was used to compare the demographic, biochemical, pharmacokinetic, and clinical parameters between the two study visits. IBM SPSS (version 28.0, IBM Corp., USA) was used for the analysis. p-values < 0.05 were considered significant.
Results and discussion
Patients characteristics
23 patients with CHF participated in the initial study visit and most of them (16/23; 70 %) were New York Heart Association class II. The median age was 77 years, and 65 % of the patients were male. The majority had mild chronic kidney disease, and the median estimated glomerular filtration rate determined by MDRD4 was 62 mL min–1. In some patients, AST, direct, and total bilirubin were elevated above the reference range, which can be present in patients with CHF (22, 23). However, none of the included patients showed clinical signs of severe liver disease. Patients were treated regularly with ramipril and the median daily dose was 5 mg (range 1.25–15 mg). The characteristics of the patients at the time of the first study visit are listed in Table I.
Table I. Characteristics of the patients at baseline (N = 23)
NYHA – New York Heart Association, LVEF – left ventricular ejection fraction, MDRD4 – estimation of creatinine clearance by four-variable Modification of Diet in Renal Disease equation, AST – aspartate transaminase
Of the 23 patients, 19 patients participated in the follow-up visit. Three patients died between the two study visits and one patient declined study-related procedures at the second visit. The median age of the remaining 19 patients was 78 years (range 56–87 years) and 74 % of them (14/19) were men. The median time between the two visits was 8 months (range 6–17 months). Table II compares the characteristics of the patients who participated in both study visits. The median body weight did not change significantly between the two visits. However, a significant increase in FFM and FFMI was observed. In addition, an increase in direct bilirubin and a decrease in direct bilirubin were noted. The values of the other parameters examined did not change significantly.
Table II. Patient characteristics at the initial and follow-up study visit (N = 19)
MDRD4 – estimation of creatinine clearance by four-variable Modification of Diet in Renal Disease equation, AST – aspartate transaminase, FFM – fat-free mass, FFMI – fat-free mass index, SMI – skeletal muscle index
The pharmacokinetics following oral administration of ramipril were adequately described using a two-compartment model for ramipril and a one-compartment model for ramiprilat. The parameters of the base pharmacokinetic model are reported in Supplementary Table S1. The available data allowed the estimation of the BSV for CL, V1, CLm, and Vm and the WSV for CL, CLm, and Vm.
We assumed a size dependence of clearances and volumes. Three descriptors of body size were tested (body weight, FFM, and NFM). NFM divides total body weight into FFM and fat mass, which is calculated from the difference between total body weight and FFM (17). The size dependence of clearances and volumes was better described by the theory-based allometric scaling using FFM and NFM than by body weight (Supplementary Table S2). In addition, the theory-based allometric models (exponent 0.75) of ramipril and ramiprilat clearances performed better than the corresponding linear models (exponent 1). In the allometric NFM models, the Ffat estimate, which accounts for the contribution of fat mass was 0.002 for V1, Vm, and CLm suggesting that our data could not support an estimate of Ffat different from 0. In the allometric NFM model for CL, the Ffat estimate was 0.310, however, the difference in objective function value (ΔOFV) compared to the FFM model was not significant when considering one additional parameter. When we tried to estimate the exponents for body size, the estimates were close to the theoretic allometric values and there was no improvement in OFV. The allometric FFM model was therefore selected as our final body size model and was included on all clearance and volume parameters (ΔOFV = –11.048).
Among the other covariates tested for inclusion in the model, the following resulted in a significant reduction in OFV: daily ramipril dose on CL (ΔOFV = 4.398) and CLm (ΔOFV = 15.174), age on CL (ΔOFV = 7.433) and estimated creatinine clearance by the MDRD4 equation on CLm (ΔOFV = 16.760). The relationships with daily ramipril dose was a power model, while the relationships with age and MDRD4 were linear models. Although the effect of daily ramipril dose on CL was insignificant by SCM criteria ( p = 0.036), its omission from the model resulted in decreased precision of the effect of daily ramipril dose on CLm, so we decided to retain this covariate effect in the model. Gender had no significant effect on pharmacokinetic parameters. Standard goodness-of-fit plots showed adequate model performance (Supplementary Fig. S1). Table III shows the parameters of the final ramipril pharmacokinetic model. Fixed effects of the model were estimated with reasonable precision, while BSV and WSV were less precise due to the limited number of patients available for analysis.
Table III. Parameters of the final ramipril pharmacokinetic model
Ka – absorption rate constant of ramipril, CL – clearance of ramipril, V1 – volume of central compartment for ramipril, V2 – volume of peripheral compartment for ramipril, Q – distribution clearance of ramipril, Vm – volume of distribution of ramiprilat, CLm – clearance of ramiprilat, CV – coefficient of variation, RSE – relative standard error, DD – daily dose, MDRD4 – estimation of creatinine clearance by four-variable Modification of Diet in Renal Disease equation
a
b
The performance of the final population pharmacokinetic model of ramipril was evaluated using a visual predictive check (Fig. 2). The median, 5th, and 95th percentiles of the observed data were in agreement with the simulations, indicating that the developed population pharmacokinetic model adequately describes the median ramipril and ramiprilat concentration profiles and their variability in patients with CHF.
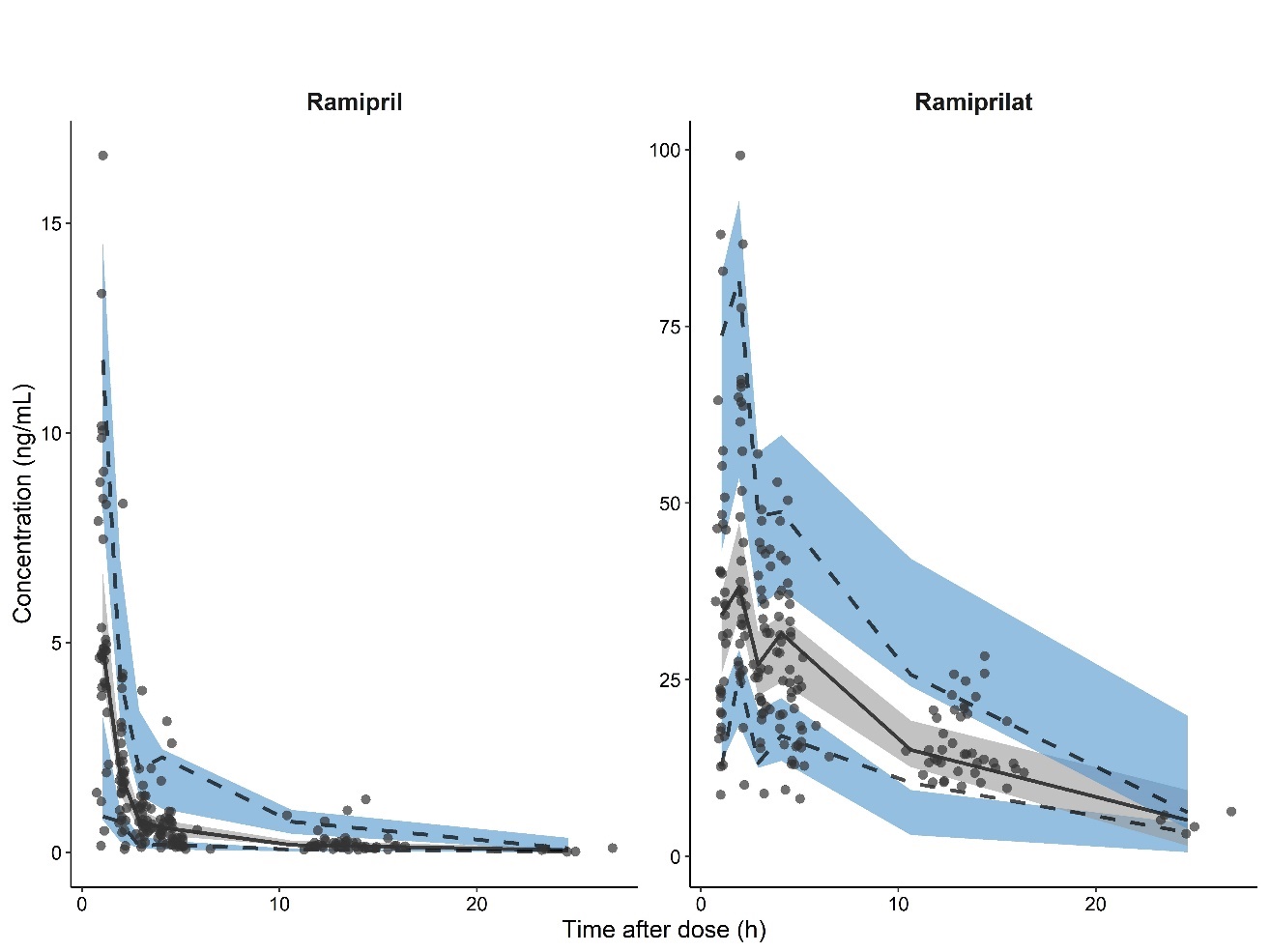
Fig. 2. Prediction-corrected visual predictive check of the final ramipril population pharmacokinetic model. Circles represent the concentration measurements and lines are the 5th, 50th (median), and 95th percentiles of the observed data. Shaded areas represent the 95 % confidence intervals of the 5th, 50th (median), and 95th percentiles predicted by the model.
Since a population pharmacokinetic model for ramipril in patients with CHF has not been developed previously, our estimates of pharmacokinetic parameters cannot be directly compared with those from previous studies. The most detailed description of ramipril and ramiprilat is a physiologically based pharmacokinetic model developed by Levitt et al. (7). Their analysis was based on their earlier three-way cross-over study in which plasma ramipril and ramiprilat concentrations were measured after intravenous administration of ramipril, intravenous administration of ramiprilat and oral administration of ramipril in 12 healthy male volunteers (6). Their estimates of absolute bioavailability based on plasma ramipril were 15 % and based on ramiprilat 44 %. The formation of ramiprilat from intravenous ramipril was 53 %. Their study yielded an estimated oral clearance of ramipril ( CL/ F) of 209 L h–1, which is in good agreement with our study. The estimated apparent oral clearance of ramiprilat ( CL/ F/fm) was 35.7 L h–1, which is significantly more than in our study (11.4 L h–1). However, this difference is to be expected as renal excretion is the major route of excretion for ramiprilat and our patients had impaired renal function.
The body weight of the patients included in our study did not change significantly between the two visits. However, we observed a mild, but significant increase in FFM and FFMI, which was not clinically relevant (Table II). This indicates that our patients were in good condition. Previous studies show that reduced FFM and FFMI are associated with an unfavourable prognosis in patients with CHF (24, 25). Our modelling analysis predicts that decreased FFM also leads to decreased ramipril and ramiprilat clearances and consequently increased drug exposure if the ramipril dose is not adjusted appropriately. This may be critical in CHF patients who develop cachexia.
The final pharmacokinetic model includes the influence of age and daily dose on CL and the influence of estimated creatinine clearance and daily dose on CLm. After absorption, ramipril enters the liver via portal circulation, where it is metabolised to ramiprilat. A part of the ramipril may escape the first-pass effect of the liver and enter peripheral tissues, and ramiprilat may also be formed locally in the kidney (26). In the liver, carboxylesterase 1, the most important hydrolase involved in the metabolism of ramipril, exhibits Michaelis-Menten kinetics (27). On the other hand, ramiprilat is only excreted via the kidneys, whereby its renal excretion is also influenced by the non-linear binding to the ACE enzyme. Consequently, the renal clearance of ramiprilat is nonlinear (7).
We found that increasing the daily dose of ramipril increased CL and CLm. As it was only possible to estimate the apparent clearance ( CL/ F) of ramipril and ramiprilat, the observed significant effect of daily dose could be a consequence of its effect on CL or on bioavailability ( F). We hypothesised that an increased daily dose would either increase CL or decrease F, and the effect on F should be the same for CL and CLm. ACE inhibitors are mainly absorbed by non-saturating passive diffusion, although active transport by jejunal peptide carrier-mediated transport may also be present (26). We found that the direct effect of the daily dose was rather due to CL. Indeed, we observed a greater decrease in OFV (ΔOFV = 15.174, p < 0.001) when the effect of daily dose on CLm was included in the model compared to the inclusion of daily dose on F (ΔOFV = 6.166, p < 0.013). This may be explained by the non-linear renal clearance of ramiprilat. At low concentrations, where most of the ramiprilat is bound to ACE, the CLm is much lower than at high concentrations, where most of the drug is free, and consequently, the CLm increases (7). The ESC Guidelines for the diagnosis and treatment of acute and chronic heart failure (2) recommend a daily target dose of 10 mg ramipril. However, because of the non-linearity of binding to ACE, Levitt et al. (7) suggested that the appropriate dose of ACE inhibitors should be based on quantitative inhibition of tissue ACE and that daily doses of ramipril above 5 mg should not significantly increase the clinical effect of the drug because the inhibited fraction of ACE is not significantly increased.
We also confirmed the association of CLm with renal function. On the other hand, renal function had no effect on CL. This is consistent with the fact that ramipril is mainly metabolised to ramiprilat in the liver, whereas the main route of excretion of ramiprilat is renal excretion (7). The relationship obtained is also consistent with the results of previous studies in which renal function significantly affected only the pharmacokinetics of ramiprilat, but not of ramipril (5, 9). Our model predicts that ramiprilat clearance ( CLm) decreases by about 1 % when creatinine clearance decreases by 1 mL min–1. A prolonged duration of ACE inhibition can therefore be expected in patients with impaired renal function. This shows that renal function must be carefully monitored in CHF patients.
Some studies have shown that the plasma concentrations of ramiprilat are increased in elderly patients. This has been explained mainly by the age-related decline in renal function, but a slower rate of absorption and metabolization than in younger patients may also contribute (28). In addition, prolonged hypoperfusion and hypoxia in patients with CHF may lead to structural changes in the liver and kidneys that could reduce the intrinsic clearance of these organs (29). In our model, age had a significant effect on CL but not CLm, which might be related to the decrease of esterases with age. On the other hand, markers of liver function (AST, direct and total bilirubin, serum albumin) had no significant effect on CL or CLm, nor did FFMI and SMI.
We also aimed to compare the pharmacokinetics of ramipril between the first and second study visits. A comparison of Bayesian estimates of the individual parameters revealed a significant difference between the two study visits for V2 and Q; however, the difference was very small and clinically irrelevant (Supplementary Table S3, Fig. S2). We found no significant difference in the clearances of ramipril and ramiprilat and therefore assume no changes in the average steady-state concentrations between the two study visits. We thus conclude that the pharmacokinetics of ramipril remain relatively stable during CHF and that regular outpatient visits at 6-month intervals seem appropriate.
Our study has some limitations. First, the number of patients participating in both study visits was relatively small, which prevented the estimation of between- and within-subject variability for some pharmacokinetic parameters. Second, although adherence to ramipril treatment was assessed prior to the study visits, there is a possibility that patients did not adhere to their drug treatment protocol. Also, with the median time of 8 months between the two study visits, we cannot extrapolate our results beyond this period. Validation in a larger cohort of patients with more than two study visits and a longer observation period is needed to confirm our findings. Finally, the inclusion of patients who develop cachexia during treatment with ramipril would be relevant for further evaluation of the effects of body composition on the pharmacokinetics of ramipril.
Conclusions
We developed a population pharmacokinetic model that adequately describes ramipril and ramiprilat pharmacokinetics in patients with CHF. Among the tested parameters of body composition, the inclusion of FFM into the model significantly improved its performance. The clearance of ramipril is also significantly influenced by age and daily dose, while the clearance of ramiprilat is affected by glomerular filtration rate and daily dose of ramipril. Therefore, renal function should be monitored in patients with CHF, and due to the relatively stable pharmacokinetics of ramipril, outpatient visits at 6-month intervals seem appropriate to evaluate ramipril therapy.
Supplementary data. – In the supplementary file, parameters of the base pharmacokinetic model are given as well as the influence of covariates associated with body size on the pharmacokinetics of ramipril and ramiprilat. Moreover, a comparison of pharmacokinetics at both study visits is available.
Conflicts of interest. – The authors declare no conflict of interest.
Funding. – The authors acknowledge the financial support from the Slovenian Research Agency research core funding No. P1-0189.
Authors contributions. – Conceptualization, K.Č.T., M.L. and M.K.K.; analysis, K.Č.T., I.G. and M.K.K.; investigation, K.Č.T., J.T. and M.L.; writing, original draft preparation, K.Č.T. and M.K.K.; writing, review and editing, I.G. and M.L. All authors have read and agreed to the published version of the manuscript.