Introduction
Sweet potato [Ipomoea batatas (L) Lam.] is one of the most widely consumed roots in many cuisines. It is rich in dietary fibers, minerals, vitamins, polyphenols and carotenoids, of which almost all are trans-β-carotene (provitamine A) (Dincer et al., 2011;U.S. Department of Agriculture and Agricultural Research Service 2011). Although the sweet potato could be eaten fresh, baking is the most popular method of cooking sweet potato (Collins and Pangloli, 1997). The other ways of cooking sweet potato are boiling, microwaving, frying and steaming. Also it has been demonstrated that sweet potatoes can be processed into liquid and semi-solid food products such as beverages, soups, baby foods, ice cream, baked products, restructured fries, breakfast cereals, and various snack and dessert items. Sweet potato puree and powders could be the main ingredients that provide the functionality required in these processed products (Truong and Ramesh, 2010). There are numerous studies on influence of thermal processing of sweet potato on changes in the chemical composition, physical and thermophysical characteristics (Ahmed et al., 2010 ;Lai et al., 2013;Padda and Picha, 2008; Picha, 1986;Takenaka et al., 2006;Wang and Kays, 2000). These changes occur as a result of heating during processing and lead to a change in the functional behavior of product. The biggest change in chemical composition, besides decrease of vitamin C and β-carotene, is decrease of starch content and yielding maltose (Lai et al., 2013;Melorose et al., 2005;Picha, 1986). Baking breaks down starch granules to form disaccharides and monosaccharides and at the same time degrading the sucrose causing maltose to become the major sugar component (Chan et al., 2014;Claude and Ubbink, 2006;Šimkovic et al., 2003;Tao et al., 2010;Zhang et al., 2002;. Depending on the method of preparation these chemical changes can occur in greater or minor extent. Thermal behavior such as the phase transition of foods is affected by these changes since it is strongly depended on the temperature and chemical composition of foods. These transitions may be either endothermic, such as melting, gelatinization, denaturation, and evaporation or exothermic, such as crystallization, oxidation, and fermentation. The phenomenon of gelatinization is very complex, and several models, which are summarized byJenkis and Donald (1993), have been proposed to explain this behavior (Jenkins et al., 1993). Starch gelatinization is a combined process consisting of the hydration of an amorphous region and subsequent melting of crystalline arrays (Randzio and Orlowska, 2005). Another phase transition of great importance is glass transition. Glass transitions are observed as a shift in the base line and it is associated with water content and water activity determinations. Glasses are not crystalline with regular structure, but retain the disorder of the liquid or amorphous state. In kinetic terms glass is as any liquid or supercooled liquid whose viscosity is between 1012 and 1013 Pa s-1, thus effectively behaving like a solid that is able to support its own weight against flow due to gravity. The glass transition temperature is of particular interest in relation to storage of food powders (Raemy, 2003).physical state of food material is changed when energy is added or removed by heating or cooling which leads to removing the water from foods or retarding the growth of microorganisms and slowing down the chemical changes that affect quality or cause food to spoil (George, 1993). Freezing is the process which involves lowering the product temperature generally to -18 °C or below causing the removing of water in form of ice (Fennema, 1966). As a method of long-term preservation for fruits and vegetables, freezing is generally regarded as superior to canning and dehydration, with respect to retention in sensory attributes and nutritive properties (Fennema, 1993). Foods and beverages do not freeze completely at a single temperature, but rather over a range of temperatures. In fact, foods high in sugar content or packed in high syrup concentrations may never be completely frozen, even at typical frozen food storage temperatures. Thus, there is not a distinct freezing point for foods and beverages, but an initial freezing point at which crystallization begins (Lozano, 2006). On the other hand, the aim of drying process is to remove water from foods and it is generally referred to heating. In most cases it is consist of heat and mass transfer which manifest on inferior product quality such as degradation of color, nutritional values, and antioxidant activity (Liaotrakoon et al., 2012). To prevent loosing such valuable compounds milder processing methods should be considered. Freeze-drying is considered as one of the best method of water removal with final products of highest quality compared to other methods of food drying such as conventional, convective air, vacuum oven drying, microconvection, solar drying, etc. (Marques et al., 2006). Biological and sensory qualities of the freeze-dried material are mainly retained in the product (Fennema, 1993). Therefore, the aim of this study was to examine a physicochemical and thermal behavior of sweet potato processed (raw, steamed and baked) and preserved by freezing and freeze-drying.
Materials and methods
Sweet potato purees (SPP) preparation
Actual sweet potato, “Beauregard” cultivar (the most producing cultivar in Croatia), was obtained from the Višnjica (Višnjica, Slatina, Croatia). Roots were stored under regular atmosphere (RA) at room temperature and before they were used for study they were washed with tap water. Raw SPP was prepared after hand-peeling. Baked SPP was prepared after baking in oven in tightly sealed foil pouch for 60 min after which the roots were hand-peeled. Steamed SPP was prepared from the peeled roots after which they were cut into slices (2 cm thick) and steam-cooked for 20 min. The raw, baked and steamed roots were pureed using a Braun Multiquick Professional 600 W Turbo mixer. After preparation, purees were preserved by freezing and freeze-drying.
Freezing and Freeze-drying
Raw, baked and steamed SPP were frozen and freeze-dried. SPP used for freezing were packaged into polyethylene bags and frozen in a laboratory freezer. Samples were maintained at -18 °C for a one week. SPP used for freeze-drying were placed onto plates diameter of 19 cm and frozen at -18 °C for 12 h before freeze-drying in a laboratory freeze-dryer (Christ Freeze Dryer, Gamma 2-20, Germany). Drying conditions were as follows: freezing temperature, -55 °C; temperature of sublimation, -35 to 0 °C; vacuum level, 0.220 mbar. The temperature of isothermal desorption varied from 0 to 22 °C under the vacuum of 0.060 mbar. Freeze-drying lasted until the total solids content was 96-99%, which was about 48 h.
Color measurement
The color of the SPP was determined by using a colorimeter (Minolta CR-300) prior to the chemical analysis. Color values, for each sample, were calculated using the averaging mode with five replications. The color measurements were made using the L*a*b* system. L* measures lightness and varies from 100 for perfect white to zero for black, approximately as the eye would evaluate it. The a* parameter measures redness when positive and greenness when negative, and b* measures yellowness when positive and blueness when negative. The color change was calculated according to equation (1), and color intensity according to equation (2).
Chemical and Physical Analysis
The content of soluble solids of SPP was measured with a table top Carl Zeiss refractometer (Deutsche Demokratische Republik - DDR) and given in Brix (°Brix). Total solids were determined in vacuum dryer; drying temperature was 65 °C and pressure was 20 mbar. Drying last until reaching a constant weight, which was about 4 h. Reducing and total sugars were determined by Luff Schoorl’s method. The starch was determined by Ewers method, an official European Commision method (directive: 71/250/EEC). The pH was determined at ambient temperature by pH meter (S20 SevenEasy™ pH-Mettler Toledo, Switzerland).
Texture analyses
The texture analysis was performed on fresh, baked, and steamed roots (before puree preparation) at the ambient temperature with a Texture analyzer TA.XTplus (Stable Mycro System, United Kingdom). The texture analyzer was equipped with 2 mm cylinder probe and heavy duty platform. On the basis of the preliminary work, the instrument working parameters were determined with the test mode compression, pretest speed at 1.5 mm/s, test speed at 1.5 mm/s, post-test speed at 10.0 mm/s, distance 10.0 mm, trigger force at 25.0 g and data acquisition rate at 200 pp. The data were analyzed using Texture expert Version 1.22 Software (Stable Micro System, United Kingdom) to measure the bioyield point and flesh firmness. The measurement was performed in 10 parallels.
Differential scanning calorimetry (DSC)
The melting point and glass transition of different processed and preserved SPP were measured by means of a differential scanning calorimetry (DSC 822e, Mettler Toledo, Switzerland). The DSC was calibrated with indium before use. Sweet potato puree (SPP) samples, from the fresh (raw), steamed and baked SPP, as well as frozen and freeze-dried raw, steamed and baked SPP were weight (20-25 mg) in the manufacturer’s stainless steel pans and cooled to -100 °C and equilibrated for 3 min, heated up to 100 °C, cooled down to -100 °C, and reheated up to 100 °C with constant heating and cooling rates at 20 °C/min, using an empty pan as the reference. The DSC thermograms of fresh and frozen samples were typical of food materials with large endothermic peak of ice melting. The initial freezing point is considered as the intersection of the tangent and the base line to the left side of the melting peak. The glass transition temperature (Tg) was identified in the freeze-dried (powdered) sweet potato samples as a vertical shift in the heat flow curve of thermogram (Liaotrakoon et al., 2012). Phase properties as the onset (Tgo), midpoint (Tgm), and endpoint (Tge) of Tg and specific heat changes (ΔH), were analyzed by DSC thermogram using a STARe Evaluation V6_V12 Conversation software. The average values of triplicate measurements were obtained. Standard deviation was used to identify the variability or error in the experimental measurements in case of freezing point as well as glass transition temperature.
Statistical analyses
All measurements were done in triplicate and data were expressed as mean ± standard deviation. The experimental data were subjected to a one-way analysis of variance (ANOVA) and Fisher’s LSD was calculated to detect significant difference (p ≤ 0.05) between the mean values. MS Excel (Microsoft Office 2007 Professional) statistical program was used for statistical analysis. Pearson’s correlation coefficient was calculated using Microsoft Excel 2007.
Results and discussion
Chemical composition and color analysis of SPP
In order to obtain the standard SPP the time of thermal treatment and texture of baked and steamed roots during processing were monitored. The roots were baked and steamed until reaching a certain flesh firmness needed for production of smooth puree. The baked (60 min) and steamed (15 min) roots picked as a good raw material for puree production had a flash firmness of 26.64 and 26.18 g, respectively (Table 1,Fig. 1). Chemical composition and color analysis of SPP made from raw, baked, and steamed roots are presented inTable 2. The results showed that SPP made from baked roots had higher total and soluble solids (20.32 and 18.95%, respectively) than SPP made from raw and steamed roots. It can be also noticed that starch content was reduced by steaming and baking which reflected on amount of total and reducing s ugars. The increase of reducing sugars level in baked SPP for 3.78% and steamed for 0.86% SPP was the result of yielding the maltose. This was confirmation of theory that higher temperatures during processing of sweet potato causing starch granules to break down forming disaccharides and later on monosaccharides (Chan et al., 2014). The Beauregard variety of sweet potato is known for its orange flesh. The intensity of orange color of SPP (Fig. 1) decreased in order; raw, baked and steamed 37.55, 31.34, and 31.24, respectively (Table 2). The highest change in color (ΔE) compared to raw SPP was observed in SPP made from baked roots (Table 2). However, after freeze-drying the lowest change in color was observed for processed SPP compared to unpreserved samples, 2.67, 2.81, 22.35 for steamed, baked and raw SPP, respectively. After freezing, the chemical composition and physical properties of SPP made from raw, baked, and steamed sweet potato roots (data not shown) were not significantly different from chemical composition and physical properties of fresh SPP made from raw, baked, and steamed sweet potato roots. The only difference was in content of soluble solids; the frozen SPP samples had slightly higher content of soluble solids than fresh SPP samples. This can be explained by the fact that ice formation causes rupture of cell walls which results in leakage of the cell content (Barbosa-Cánova et al., 2005). Another preservation technique used in this study was freeze-drying. Pictures of powdered and rehydrated freeze-dried SPP are presented inFig. 2 and3, respectively. The results of physicochemical properties of freeze-dried SPP made from raw, baked, and steamed sweet potato roots (Table 3) showed that all samples had a high level of total solids (98.19, 96.78, 97.73%, respectively) as well as a low water activity (0.15, 0.21, 0.19, respectively). A small change in the proportion of sugar in favor of the freeze-dried samples was also observed. The highest change in color (22.35) compared to raw SPP before preservation was observed in SPP made from raw sweet potato where the intensity of color dropped from 37.55 to 21.23. The lowest change in color (2.67) was observed in SPP made from steamed sweet potato and the highest intensity of color (26.16) was measured in SPP made from baked sweet potato.
Superscripts with the different letters in a same column are significantly different at a probability, p<0.05.
Superscripts with the different letters in a same row are significantly different at a probability, p<0.05.
*The results are given on dry weight basis; Superscripts with the different letters in a same row are significantly different at a probability, p<0.05.
Thermal behavior of SPP
The results of thermal properties of fresh and frozen SPP made from raw, baked, and steamed sweet potato roots are given in Table 4. The transition temperatures for initial freezing point (Fig. 4) were determined as the intersection of the tangent and base line to the left side of the melting peak (Bai et al., 2001). The results showed that SPP made from baked sweet potato roots had the lowest initial freezing point (-2.80 °C) followed by SPP made from steamed (-2.63 °C) and raw (-0.71 °C) sweet potato roots. This result is in negative correlation (r = -0.845) with solid content of SPP made from baked, steamed and raw sweet potato roots, respectively. The lower initial freezing temperature is result of increase of solid content, mostly sugars which act as plasticizer and increase the amount of water which was unavailable for freezing and reduced the amount of energy required to freeze the processed SPP (Roos, 1995. Analysis of experimental data showed that up to 87 J/g of heat need to be removed to freeze SP puree. Consequently SPP made from raw sweet potato roots had the lowest melting temperature (Tm), while Tm of SPP made from baked and steamed sweet potato roots had higher melting temperature and were not significantly different (p < 0.05). The enthalpy of transition (ΔH) is the peak temperature, which corresponds to complete melting in organics and the energy that the melting transition needs in order to occur. The highest energy for melting transition (ΔH) is needed for SPP made from raw sweet potato roots followed by steamed and baked sweet potato roots -103.79, -103.63, and -102.90 J/g, respectively. The ΔH is also in correlation to moisture content of samples. In samples with higher moisture content the energy needed for transition is higher (Roos, 1987). Frozen SPP samples followed the same trend. InTable 5 the heat flow properties at glass transition state of the freeze-dried SPP were shown. Considering thermal behavior of fresh and frozen SPP, there was no evidence of glass transition in temperature range studied. The lacking of glass transition in studied temperature range indicates that samples preserved at -18 °C were unstable since it was suggested that deteriorative changes in frozen food increase by orders of magnitude with small increase in temperature above the glass transition temperature of the maximally freeze-concentrated solute matrix (Roos, 1995). A glass can be considered as a solid liquid with an extremely high viscosity through which unstable state can achieve apparent structural and chemical stability (Schebor et al., 1999A. The increase of liquid viscosity due to low temperature and low water content could result in a brittle glass. This state leads to a significant arrest of translational molecular motion so that translational mobility and diffusion are considered to be virtually non-existent. Thus chemical reactions that are dependent upon the diffusion of molecules would slow down or would not occur at all, achieving stability of the biological compound for a long period of time (Koster, 1991;Schebor et al., 1999B;Sun and Leopold, 1994). The most serious problem in dehydration of foods is caused by the difficulty of water removal from sugar containing foods and product stickiness at late stages of dehydration process and storage. Heat flow properties at glass transition state of the freeze-dried SPP are presented inTable 5. The glass transition in freeze-dried SPP made from raw sweet potato roots was not detected. The lacking of glass transition in the freeze-dried SPP made from raw sweet potato roots was result of high content of total solids (98.19%) and low water activity (0.15) of sample. However, in the freeze-dried SPP made from baked and steamed sweet potato roots, the glass transition was detected in the range of 39 and 42 °C, but with no significant difference (p > 0.05) (Fig 5). The presence of Tg in freeze-dried SPP made from baked and steamed sweet potato roots is the result of the higher water content due to higher amount of sugars compared to SPP made from raw sweet potato roots. Due to very low time availability of freeze-drying, the solutes like sugars and acids present in the food become amorphous. These solutes are soluble in water, which acts as a plasticizer and decreases the Tg of the system with increase in moisture content and water activity (Jaya and Das, 2009;Roos and Karel, 1991;Roos, 1993 ;Roos, 1987). This is evident from the content of total solid of SPP made from baked (96.78%) and steamed (97.73%) sweet potato roots as well as water activity 0.21; 0.19, respectively. Even though the higher content of sugars decrease Tg of SPP made from baked and steamed sweet potato roots, the temperatures at which the glass transition occurs are still high enough for storage of such product at room temperature.
Superscripts with the different letters in a same row within the same sample (fresh or frozen) are significantly different at a probability, p<0.05.
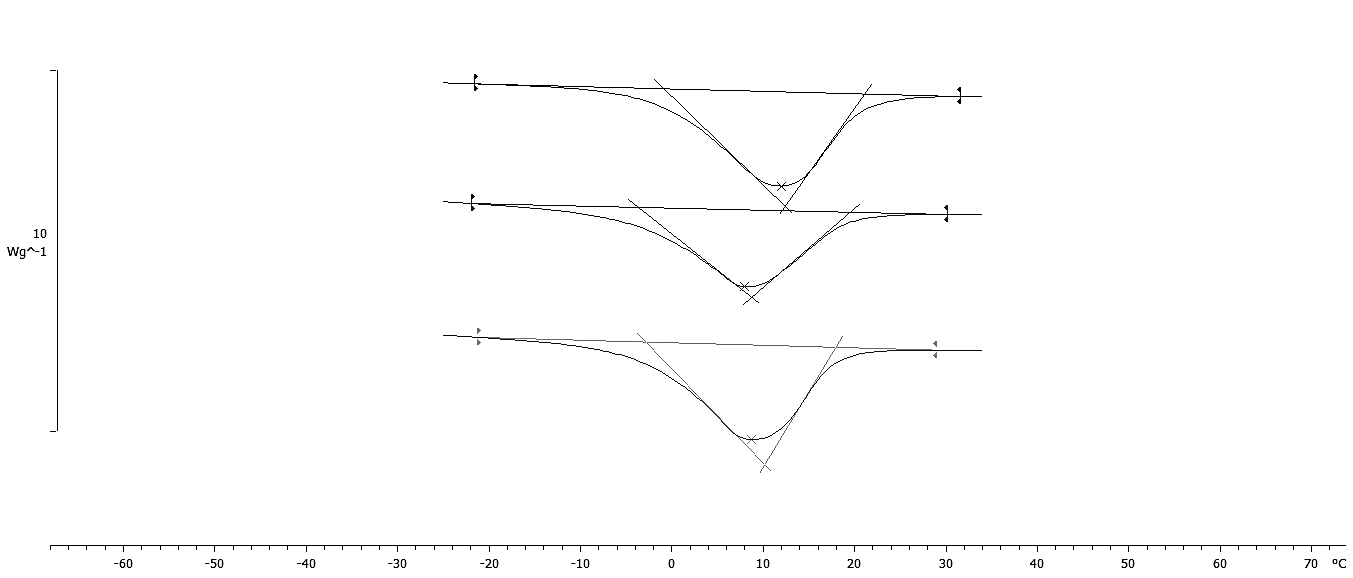
Heat flow properties | Raw | Baked | Steamed |
Tgo (°C) | n.d.* | 34.45 ± 1.966a | 33.31 ± 1.442a |
Tgm (°C) | n.d. | 41.41 ± 2.432a | 39.93 ± 0.693a |
Tge (°C) | n.d. | 49.85 ± 3.543a | 49.92 ± 2.029a |
∆Cp (J/gK) | n.d. | 0.324 ± 0.011b | 0.423 ± 0.003a |
*n.d. not detected; Superscripts with the different letters in a same row are significantly different at a probability, p<0.05.
Conclusions
From the results presented it could be concluded that thermal treatment caused change of the physicochemical properties of sweet potato. These changes are mostly related to the sugar and starch content of sweet potatoes, resulting in a sweet flavor and a soft texture. Changes of physicochemical properties of sweet potato also had an impact on frozen and freeze-dried SPP. SPP made from baked and steamed sweet potato roots had a lower freezing and melting points compared to raw SPP. In freeze-dried samples, there were also differences between raw and thermal treated SPP. SPP made from baked and steamed sweet potato roots had lower amount of total solid and a higher water activity which contributed to a lower temperature of the glass transition in these samples. Nevertheless, these temperatures were much higher than a room temperature.