Introduction
Kefir grain contains mainly lactic acid bacteria, acetic acid bacteria, and yeast held together in a polysaccharide matrix (kefiran) (Güzel-Seydim et al., 2011). Having either whitish or slightly yellowish appearance, the grains have irregular shape. Kefiran is present in the capsular material of some large, rod-like bacteria, especially Lactobacillus spp. In general, Lactobacillus kefir (Toba et al., 1987) or Lactobacillus kefiranofaciens (Yokoi et al., 1990) account for polysaccharide biosynthesis. Kefiran is a water-soluble exopolysaccharide (EPS) that contains approximately equal amounts of glucose and galactose monosaccharides (Micheli et al., 1999). It is commonly used as a stabiliser, an emulsifier, a thickener, and fat replacers exhibiting antibacterial, antifungal and antitumour activity (Maeda et al., 2004). There are many studies aiming to increase kefiran production by fermentation (Rimada and Abraham, 2003). However, a literature search revealed the lack of a validated HPLC method for the simultaneous detection of sucrose, glucose, galactose, xylose, arabinose and ribose in kefiran.
Lactobacillus kefir, Lactobacillus parakefir, Lactobacillus kefiranofaciens and Lactobacillus kefirgranum are the major exopolysaccharide -producing bacteria present in kefir grain microbiota (Cheirsilp et al., 2003a; Cheirsilp et al., 2003b; Wang et al., 2008). Currently, kefiran started to be commonly used as a food-grade gum and fortification agent in the food industry, and in the development of exopolysaccharides produced by microorganisms (Piermaria et al., 2009). In recent years, the development of exopolysaccharides produced by microorganisms has become more of an issue because the demand for natural polymers to be used in different industrial applications is gradually increasing. The use of lactic acid bacteria (LAB) in the fermented dairy industry is common owing to the fact that they can synthesize EPS, which will eventually contribute to the texturizing, viscosifying, and syneresis-lowering characteristics (organoleptic properties) of the product. The importance of these EPS comes from the fact that they provide the food with functional properties and health benefits (Laws et al., 2001). Kefiran might have therapeutic, immunostimulatory, antimutagenic, antiallergenic and antiulcer activities, and it might act as a prebiotic compound (Yoon et al., 1999; WonHo et al., 2003). EPS has a significant physical effects on the metabolic products of microorganisms, although they are not used as sources of carbon or energy. LAB, such as Lactobacillus, Streptococcus, Lactococcus and Leuconostoc produce EPS with different constituents. Bacterial synthesis of polysaccharides is affected by temperature, pH, the type of carbon source, the duration of fermentation and by the interactions between those parameters (De Vuyst and Degeest, 1999; Ruas-Madiedo et al., 2002). Streptococcus thermophilus produces 50 to 350 mg/L of EPS (Petry et al., 2003), and the highest EPS concentrations were achieved at 42 °C and a pH of 6.2 (De Vuyst et al., 1998). S. thermophilus was reported to prefer lactose rather than glucose in the medium (Degeest and De Vuyst, 2000). Looijesteijen et al. (1999) reported that the use of fructose rather than glucose as a substrate facilitated higher EPS production by Lactococcus lactis spp. cremoris B40. Mozzi et al. (2001) reported that biosynthetic enzyme activity was elevated during polymer production by Lactobacillus casei CRL 87 and that the utilization of different carbon sources (glucose and galactose) affected EPS production.
The EPS production by lactic acid cultures ranged from 50 to 350 mg/L for S. thermophilus, from 60 to 150 mg/L for Lactobacillus delbrueckii spp. bulgaricus, from 25 to 600 mg/L for L. lactis spp. cremoris and from 50 to 60 mg/L for L. casei (Pham et al., 2000). By modifying the medium composition and cultivation conditions, the EPS amounts were determined to be 3000 mg/L, 2100 mg/L, 490 mg/L, and 600 mg/L for S. thermophilus, L. delbrueckii spp. bulgaricus, L. casei, L. lactis spp. cremoris, respectively (Duboc and Mollet, 2001).
Exopolysaccharide ZW3 was reported to be produced from Lactobacillus kefiranofaciens ZW3 that was isolated from the Tibet kefir grain. After being compared with other commercially available hydrocolloids such as guar gum, locust gum, and xanthan gum, ZW3 was determined to have much better emulsifying capability (Wang et al., 2008).
Exopolysaccharides, kefiran, production in the medium containing various carbon sources (glucose, galactose or lactose), in which kefir grain was activated, was studied. The aim of this research was to perform a quantitative measurement and extraction of the bacterial polysaccharide in kefir grains by modifying an HPLC assay procedure for determining its sugar composition, and to quality of its structure using atomic force microscopy.
Materials and methods
Materials
The kefir grains were purchased from the Danem Co (Isparta, Turkey). Non-fat milk powder was obtained from Pınar Co., (Izmir, Turkey). All chemicals were of analytical reagent grade and all solvents were of HPLC grade. Sucrose, glucose, galactose, xylose, arabinose and ribose were obtained from Sigma-Aldrich (USA). Trifluoroacetic acid (TFA) was purchased from Merck (Darmstadt, Germany). Water, with a resistivity of 18.2 MΩ-cm, was obtained using a Milli-Q water purification system (Millipore, Bedford, MA, USA). All dilutions were performed in volumetric flasks, and liquids were filtered through 0.45 µm nylon filters prior to use.
Fermentation
Reconstituted milk (10 % total solids) was prepared using non-fat milk powder fortified with either glucose-GLKG (2 % w/v), galactose-GAKG (2 % w/v) or lactose-LKG (2 % w/v). The control treatment was prepared from non-fat milk powder (CKG) (12 % total solids). After pasteurization at 90 °C for 15 minutes, the reconstituted milks (100 mL) were inoculated with kefir grains (2 g) and fermented at 25°C. Fermentation was continued at 25 °C until a final pH of 4.6 was reached (after approximately 18 hours of incubation). After the kefir grains were separated under aseptic conditions, they were kept in sterilized water at 4 °C until the next inoculation. Kefir fermentation was carried out in 250 mL sterile jars on a rotary shaker (100 rpm) at 25 °C. Kefir grains need frequent fermentations over 9 days; three inoculations were carried out each week, and the weekly biomass increase was recorded.
Determination of the increase in kefir grain biomass
The biomass of kefir grains usually increases as they are subjected to fermentation. The kefir grains were aseptically separated from the fermented milk by using stainless steel sieves, and then washed with sterile distilled water and transferred into autoclaved aluminium weighing dishes containing sterilized paper towels to remove excess water. After the paper towels were removed, the kefir grains were weighed using an analytical balance.
Isolation and purification of the exopolysaccharides in the kefir grain
Kefiran was extracted from kefir grains activated in different carbon sources at 25 °C for 18 hours over 9 days. Kefir grains were added to boiling water in a ratio of 1:10 and stirred for 5 minutes. The mixture was centrifuged at 3.313 (× g) for 20 minutes at 4 °C (Sorvall RT7, Kendro Instruments Australia Pty Ltd., Lane Cove, NSW, Australia). Polysaccharides in the supernatant, or kefiran broth, were precipitated by adding an equal volume of cold absolute ethanol (-20 °C) and left at 4 °C overnight. The precipitate was then collected by centrifuging at 3.313 (× g) for 20 minutes and dissolved in distilled water. The ethanol precipitation and water dissolution treatment was repeated three times, and was followed by lyophilisation (or freeze-drying). The concentration of EPS in the suspension was quantified using the phenol-sulfuric method and expressed as lactose, glucose or galactose equivalents in mg/L (Cerning, 1995; Dubois et al., 1956).
Chromatographic procedure
A CARBOSep Coregel-87P analytical column of internal diameter 300 mm × 7.8 mm was used for the stationary phase (2008 Transgenomic® Inc., USA). The mobile phase consisted of deionized water at a flow rate of 0.4 mL/min. The column temperature was 85 °C and the injection volume was 20 μL. For the investigated compounds, the retention time values (tR) were determined from three separate injections into the mobile phase. Stock solutions (100 μg/mL) of sucrose, glucose, galactose, xylose, arabinose and ribose were prepared by dissolving them in the mobile phase. All working standards were diluted with the mobile phase. All solutions were kept away from light and were used in the first 24 hours. 10 mL of standard solutions of the studied compounds were prepared using the mobile phase over different concentration ranges. The linearity response of the sugars was initially studied using different concentrations levels of the working standards injected three times and covering the entire working range of 25 to 150 μg/mL for glucose, 20 to 120 μg/mL for galactose, 90 to 600 μg/mL for xylose, 10 to 50 μg/mL for arabinose, 30 to 100 μg/mL for ribose and 130 000 to 240 000 μg/mL for sucrose. The peak area counts were plotted against the concentration of the corresponding analyte, and the regression equation was adjusted. Kefiran was hydrolysed using 2 M trifluoroacetic acid (TFA) at 100 oC for 8 hours. High performance liquid chromatography was used to determine the sugar quantities in the hydrolysed kefiran.
The rheology of the exopolysaccharides of kefir grains
The viscosity was determined at 25 °C in a Brookfield Rotational Rheometer (model DV-II ProLV; Brookfield Engineering Laboratories, Middleboro, MA) according to Power Law methods (Kök-Taş et al., 2013). A small sample adapter was fixed with spindle SC4-18 to the Brookfield rheometer. The viscosity was measured at rotational speeds from 50 to 140 rpm, increasing by 10 rpm every 5 seconds. Duplicate analyses were carried out at 10 °C, and the data obtained was processed using Rheocalc Application Software (V3.2 Build 47-0; Brookfield Engineering Laboratories).
Atomic force microscopy (AFM)
AFM (Veeco Multimode V, USA) with a 2 µm × 2 µm horizontal and 50 µm vertical range was used to image exopolysaccharide films kept in equilibrium at 50 % relative humidity (RH), in order to study the surface morphology. Film samples cut into thin pieces were used to fit the AFM imaging head, and they were attached onto the sample holder using double-sided tape. Each film specimen was probed in tapping mode and a silicon cantilever having 20 to 80 N/m spring constant located over the samples was used for this purpose. The AFM material was n-type (1 to 10 Ohm-cm) silicon. 3D images of each sample were prepared (Khulbe et al., 1996). To keep the consistency, all images were received from each surface center. The roughness value of the films was determined by NanoScope SPM software (Version 7.20). AFM can be used to determine various roughness parameters, and in this study, we determined Sq (nm), Sa (nm), Smax (nm), and Sp parameters by AFM (Fabra et al., 2009).
Statistical analysis
Three repetitions were carried out during the experiment and all samples were analysed in triplicate. The significance of using different supplements for the increase in kefir biomass was analysed using ANOVA at the 5 % level. Mean values were compared using Duncan’s multiple range test with SPSS software (SPSS, 2006). Data was expressed as the mean of three measurements. Origin proVersion 8.0 statistical software (Origin Lab Inc., Northampton, MA) was used to analyze the HPLC data.
Results and discussıon
The observed biomass increments in kefir grains after different treatments are presented in Fig. 1. The highest biomass increase was observed with grains grown in reconstituted milk containing galactose. After 9 days, the percentage biomass increase in the control and in the samples supplemented with glucose, galactose, and lactose were 284 %, 306 % and 328 %, respectively (Fig. 1). The biomass concentration and exopolysaccharide concentration reached their highest levels during kefir grain growth on galactose (P<0.05). Although galactose and lactose enrichment significantly increased the kefir grain biomass after 9 days (P<0.05), glucose did not show a significant increase. Guzel-Seydim et al. (2011) reported that the largest biomass increase in kefir grains was obtained when whey protein was used in low fat milk. According to Gorsek and Tramsek (2007), the rotation time of the low speed shaker had the highest effect, whereas the concentration of glucose and the medium temperature had much less influence.
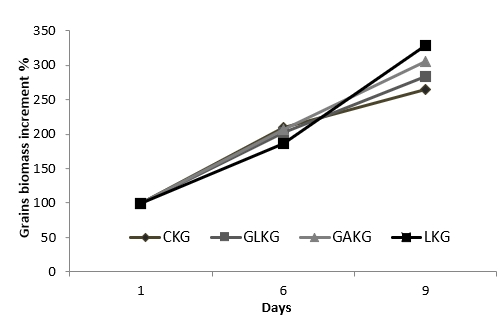
Figure 1. Biomass increment (%) of kefir grains over 9 d of incubation. Different carbon sources for growth of kefir grains included control (CKG; nonfat milkpowder) or nonfat milk powder fortified with 2 % glucose (GLKG), 2% galactose (GAKG), or 2 % lactose (LKG)
Table 1. Amount of kefiran produced by kefır grains using different carbon sources
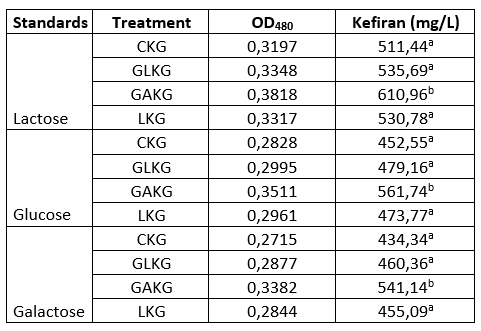
aValues with the same superscript letters within a column are not significant different (P<0.05)
bValues were given as mean±standard deviation
The EPS contents of the kefir grain samples were in accordance with each other with respect to the glucose, galactose and lactose standards (Table 1). Kefir grain using galactose had 610.96 mg/L EPS, kefir grain without a carbon source, kefir grains using glucose and lactose had 511.44, 535.69 and 530.78 mg/L EPS, respectively (Table 1). When galactose was used as a carbon source, dramatic increase was observed in the amount of EPS produced (P<0.05). Such results can be explained by the fact that galactose and glucose have influence on the enzymatic activities in sugar metabolism and on the sugar nucleotide formation. Mozzi et al. (2001) similarly reported that both PEP-gal-PTS and galactokinase activities were observed in galactose medium, confirming that these two systems mediated EPS production in L. casei CRL 87. The medium composition and growth conditions (i.e., medium, temperature and incubation time) of the LAB have a great influence on the total production of EPS (Cerning et al., 1994; Grobben et al., 1995). Successful growths of Lactobacillus helveticus ATCC 15807 and L. casei CG11 were observed in galactose, glucose or sucrose containing medium, but in that report, the EPS production in galactose medium was much less compared to that in others sugar media (Cerning et al., 1994). EPS biosynthesis in L. bulgaricus NCFB 2772 was reported, by Grobben et al. (1995), to be possibly dependent on the carbon media.
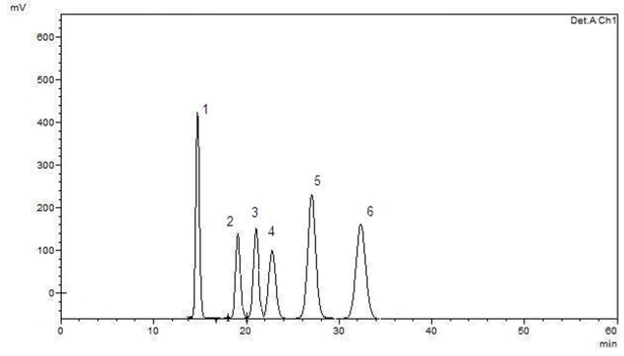
Figure 2. Typical chromatogram of studied compounds separation using the chromatographic conditions described in the text (1-sucrose, 2-glucose, 3-xylose, 4-galactose, 5-arabinose, 6-ribose)
Table 2. Statistical evaluation of the calibration data of investigated compounds by HPLC method
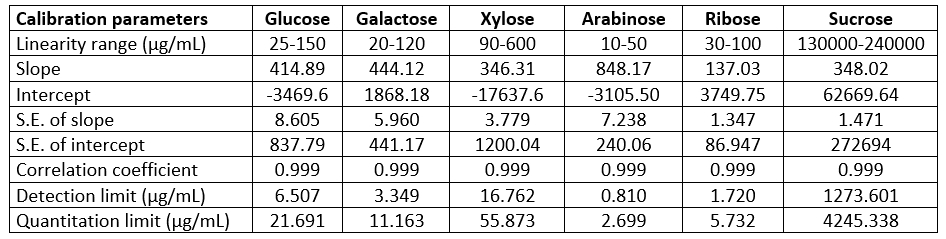
In this part of our study, the separation and retention times of sucrose, glucose, galactose, xylose, arabinose and ribose in kefiran were optimized by varying HPLC conditions. A CARBOSep Coregel-87P analytical column (of internal diameter 300 mm × 7.8 mm) yielded a greatly improved efficiencies for the compounds studied. The chromatographic separation was carried out in 34 minutes. The retention times were 16.24 minutes for sucrose, 19.40 minutes for glucose, 21.39 minutes for xylose, 23.68 minutes for galactose, 26.86 minutes for arabinose and 32.95 minutes for ribose. A standard chromatogram is demonstrated in Fig. 2. Linear regression analysis was performed for each of the six compounds using the external standard method. The calibration curves and equations for the compounds investigated were shown in Table 2. The limit of detection (LOD) and limit of quantification (LOQ) of the compounds were determined. These parameters were calculated at 3.0:1 and 10:1 signal/noise ratios, respectively. The HPLC conditions were optimized in this study to quantify the compounds in kefiran. The proposed method was applied for the direct detection of sucrose, glucose, galactose, xylose, arabinose and ribose in kefiran. In this method, only filtration and adequate dilution steps were performed and no sample extraction or evaporation was used. The obtained results are given in Table 3. Peaks belonging to the compounds estimated by the proposed HPLC method were observed in the chromatogram (as can be seen in Fig. 3). The proposed HPLC method was precise, accurate and reproducible, and it is useful for simultaneous determination of sucrose, glucose, galactose, xylose, arabinose and ribose in kefiran.
Table 3. Results of the assay of investigated compounds in kefiran

ND*: Not detected
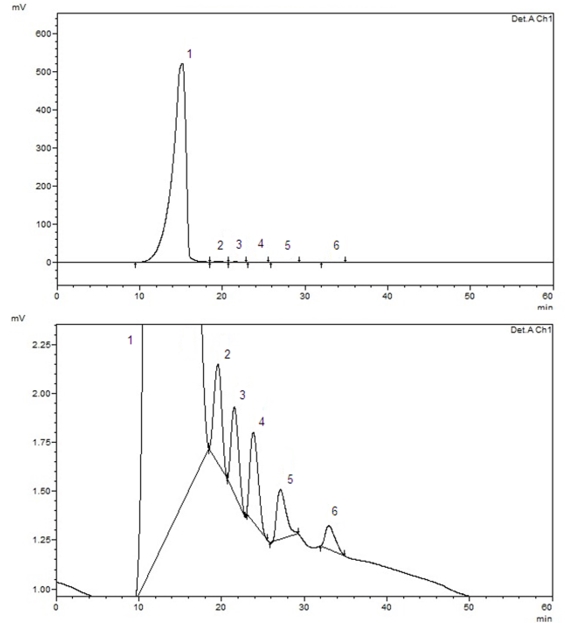
Figure 3. HPLC chromatograms of GAKG (1-sucrose, 2-glucose, 3-xylose, 4-galactose, 5-arabinose, 6-ribose)
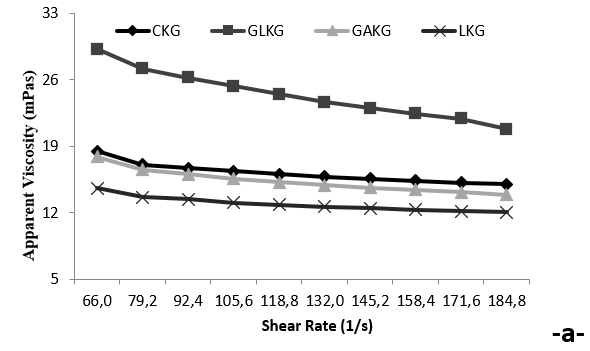
Figure 4a. Apparent viscosity-shear rate reograms of EPS produced by kefir grains using different carbon sources
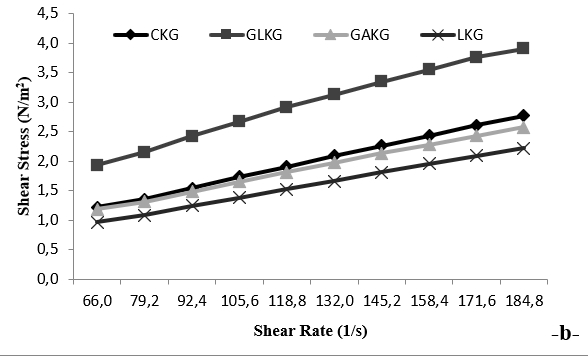
Figure 4b. Shear stress-shear rate reograms of EPS produced by kefir grains using different carbon sources
Hasheminya and Dehghannya (2020) reported that total sugars content in the samples treated with hot water was 86.55 %. According to the results of the structural evaluation of kefiran, it had a backbone composed of (1→6)-linkedGlc, (1→3)-linked Gal, (1→4)-linked Gal, (1→4)-linked Glc and (1→2, 6)-linked Gal, with branch attached to O-2 of Gal residues and Glc residues situated in the structure end (Ahmed et al., 2013; Ghasemlouet al., 2012).
The purified EPS from kefir grains using glucose had relatively higher viscosity than that without a carbon source. The apparent viscosity of kefirans from kefir grains using glucose, galactose or lactose was 29.2, 17.9 and 14.6 mPa.s at a shear rate of 66.0 s −1, respectively. The viscosity of EPS solution decreased from 29.2 to 12.2 mPas, at shear rate from 66.0 to 184.8 s −1, indicating the presence of the kefiran’s non-Newtonian behaviour (shear-thinning) (Fig. 4a). Shear stress values of different kefirans increased, while the shear rate increased in line with the Power Law model. Increasing the shear rate progressively reduced the viscosity, and the samples exhibited high viscosity. This behaviour was reversible, and the shear stress increased on expanding the shear rate (Fig. 4b). Ghasemlou et al. (2011) hydrolysed the purified EPS into individual monosaccharides using TFA. Abu-Jdayil and Mohammed, (2002) reported that EPS was composed of glucose (Glc) and galactose (Gal) with a relative molar ratio of 1.0:1.1. Loose physical contact between proteins, as a result of weak electrostatic and hydrophobic bonding, are expected to lead to shear-thinning behaviour in liquid-fermented milks. Furthermore, it showed pseudoplastic behaviour because of the strong interaction between water molecules and the hydroxyl groups (−OH) of different kefiran. A similar experiment was reported about the potential selection of Lactobacillus plantarum KF5 EPS as a biothickener and stabilizer agent. Kefiran gel could be formed at the presence of sugars (sucrose and fructose) and these sugars had different effects on the rheological at-tributes of kefiran gel. The sugars could hold water, modifying the water-polymer and polymer-polymer interactions. Sucrose and fructose actually acted like plasticizer, because they could easily make -inter-changeable linkages (Zavala et al., 2015). The use of exopolysaccharide-producing starter cultures is known to improve the rheological properties of fermented dairy products by improving the texture, reducing the separation of the serum and improving the water retention capabilities. In this study, the reduction in the EPS content during storage was thought to stem from the degradation of EPS by bacterial enzymes, and the viscosity values decreased correspondingly in the GAKG and LKG samples. A similar result was obtained by Wang et al. (2010) as to be 13 nm. These results were compatible with those of Ahmet et al. (2013) and Wang et al. (2010). In all studies, it was seen that the shape and the structure were different in each EPS.
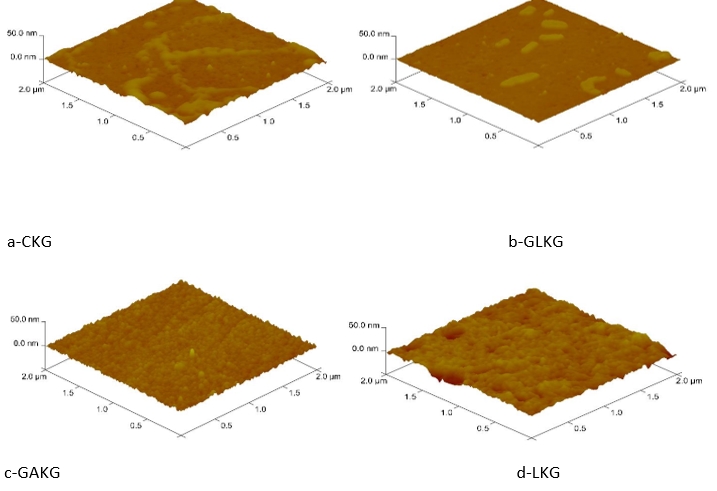
Figure 5. Atomic force microscopy (AFM) topographic images of molecular structures of different kefir grains
Table 4. Roughness parameters obtained from atomic force microscopy images; S q(nm) S a(nm), S max(nm) and S p
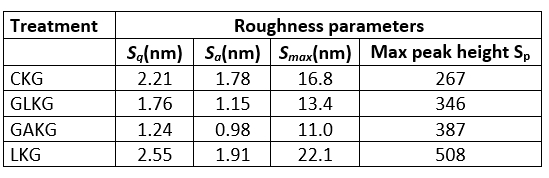
The topographical AFM images of different kefiran are shown in Fig. 5. Three-dimensional images of different kefirans in a 10 µg/mL aqueous solution showed spherical lumps and chains (Fig. 5). The roughness parameters are shown in Table 4. The maximum height of lumps is from 16.80 to 22.1 nm. The adhesion between polysaccharides and surfaces was quantitatively measured by AFM. Since it is possible to control their adhesion by biopolymer characteristics, the use of AFM is especially convenient in the investigation of polysaccharides on microbial and different kinds of cells (Motedayen et al., 2013).
Conclusions
The amount of exopolysaccharides produced by the kefir grain, comprising many different types of bacteria and yeast, was determined to be reasonably high. The biomass concentration and exopolysaccharide concentration reached their highest levels during kefir grain growth on galactose A literature search revealed the lack of a validated HPLC method for the simultaneous detection of sucrose, glucose, galactose, xylose, arabinose and ribose in kefiran. Kefiran production was more successful when galactose was used as carbon source.
Acknowledgements
This work was supported by the Danem Co., (Suleyman Demirel University, Technopark, Isparta, Turkey) for providing kefir The financial support for this work from Scientific and Technical Research Council of Turkey (TUBITAK) project ≠108O872 and Scientific Research Commission at Suleyman Demirel University Project ≠956 is gratefully acknowledged.
Sažetak
Kefiran je egzopolisaharid (EPS) kojeg proizvode specifični mikroorganizmi sadržani u kefirnom
U ovom je radu kefrno zrno aktivirano u medijima koji su sadržavali različite izvore ugljika (glukoza, galaktoza ili laktoza). Sastav monosaharida u kefiranu koji je tako nastao određivan je primjenom tekućinske kromatografije visoke djelotvornosti (HPLC). U svrhu kvantitativnog i kvalitativnog određivanja saharoze, glukoze, galaktoze, arabinoze, ksiloze i riboze u kefiranu korišten je HPLC s detektorom za indeks refrakcije (RI). Također su određivana i fizikalna svojstva različitih vrsta kefirana. Količina kefirana nastalog u kontrolnom uzorku te u uzroku medija s galaktozom bio je 511,00 odnosno 610,55 mg/L. Kefiran nastao u mediju s galaktozom pokazao je više statistički pozitivnih utjecaja u usporedbi s ostalim ispitivanim uzorcima. Prividna viskoznost kefirana iz medija s galaktozom određivana je pri brzini smicanja 66,0 s -1 i iznosila je 29,2 mPas. Od morfoloških svojstava određivana je hrapavasot površine različitih vrsta kefirana primjenom mikroskopije atomskom silom (AFC) pri čemu je maksimalna visina grumena iznosila između 16,80 i 22,10 nm. U ovom istraživanju je utvrđena prisutnost saharoze, glukoze, galaktoze, arabinosze, ksiloze i riboze u svim uzrocima kefirana.
Key words: kefiran; kefirno zrno; monosaharidi; tekućinska kromatografija visoke djelotvornosti (HPLC)