Introduction
In recent years, a lot of attention has been paid to public health and prevention of diseases caused by modern lifestyles and nutrition. For this reason, researchers have increasingly focused on studying traditional products, often unjustly forgotten, such as donkey’ milk ( DM), whose beneficial therapeutic and cosmetic properties have been known since ancient times (Coppola et al., 2002). The DM is currently referred to as a novel dietary food and a good alternative for infant nutrition in the case of cow’s milk protein allergy ( CMPA), due to its specific chemical composition, including low casein content and protein profile similar to that of human milk (Criscione et al., 2009; Gubić et al., 2015; Ivanković et al., 2009; Monti et al., 2007; Polidori et al., 2015). According to the available data, DM has an anti-inflammatory, anti-viral, anti-proliferative, anti-tumor and antimicrobial activity (Altomonte et al., 2019; Brumini et al., 2013; Jirillo et al., 2010; Koutb et al., 2016; Mao et al., 2009; Martini et al., 2021; Massouras et al., 2020; Šarić et al., 2012; Tidona et al., 2011). Furthermore, it has beneficial effects on atherosclerosis prevention and could also improve the human immune system (Jirillo et al., 2010). Besides that, DM can be used in mixture with goat milk to produce high-quality extra-hard cheese (Šarić et al., 2016).
DM has been a part of traditional medicine in different world regions, especially in Africa, Asia, Eastern and Southern Europe (Blench, 1999; Kugler et al., 2008; Zhang et al., 2008). This type of milk has been used as a natural remedy for the treatment of different respiratory diseases, including pneumonia. It has been reported that the Maasai people in Africa give warm, freshly milked DM to their children with pneumonia (Farnaud and Evans, 2003). The use of DM for this purpose has also been observed in England, Italy and the Balkan countries (Fernando and Starkey, 1997; Ivanković et al., 2000; Tidona et al., 2011). Although most fatalities occur in developing countries, pneumonia is equally present in developed and developing countries. Worldwide, more children die from pneumonia than from any other infectious disease. According to the United Nations Children's Fund ( UNICEF) over 800,000 children under the age of five were estimated to have died from pneumonia in 2018 (UNICEF,2018). Different bacteria can cause bacterial pneumonia. One of the most important is Klebsiella pneumonia, which causes a wide range of infections, from mild urinary infections to severe bacteremia and pneumonia with a high mortality rate, usually affecting immunocompromised patients (Cortes et al., 2002). Pulmonary infections caused Klebsiella pneumonia are often associated with a rapidly progressive clinical manifestation, which leaves little time to establish adequate antibiotic treatment (Cortes et al., 2002).
Although DM has been traditionally used to treat different respiratory diseases, there is no scientific proof of its beneficial activity in the prevention and cure of respiratory diseases. Even though the amount of research concerning DM functionality has increased, the studies on its antimicrobial activity are still limited, and are mainly related to foodborne pathogens (Fratini et al., 2016; Massouras et al., 2020; Šarić et al., 2014a, Šarić et al., 2014b; Šarić et al., 2017; Tidona et al., 2011; Zhang et al., 2008). There is no available data on antibacterial activity of DM toward clinical isolates of K. pneumoniae. Lysozyme ( LYZ) and lactoferrin ( LF) are described as the main antimicrobial agents in DM (Bhardwaj et al., 2020; Brumini et al., 2016; Coppola et al., 2002; Martini et al., 2019; Tidona et al., 2011; Vincenzetti et al., 2008; Zhang et al., 2008) while the calcium (Ca) content may also affect the antimicrobial activity of this milk (Šarić et al., 2014a; Šarić et al., 2017).
The specific objective of this study was to investigate the effect of the level of contamination ( LC), incubation temperature ( T), the incubation period ( t), LYZ, LF and Ca content on the number of K. pneumoniae in intentionally contaminated DM.
Materials and methods
Collection of milk samples
Individual milk samples were collected from the Special Nature Reserve "Zasavica" located in the northwest of Serbia from seventeen clinically healthy Domestic Balkan donkeys. The donkeys belonging to different stages of lactation were hand milked. After milking, milk samples were immediately chilled to 4 °C and transported to the laboratory where the samples were frozen at -20 °C. Nine of the seventeen samples marked with numbers 1 to 9 were used for tests performed at 9 and 15 °C, whereas the remaining eight samples marked with numbers 10 to 17 were used for the test at 38 °C.
Determination of protein profile
A modified method described by Tidona et al. (2011) was used for the preparation of samples. Milk was diluted with buffer (0.125 M Tris–HCl, 4 % SDS, 2 % glycerol, 2 % β-mercaptoethanol, pH 6.8), in 1:1.5 (v/v) ratio of milk to buffer, and afterwards heated at 100 °C for 5 minutes. Agilent 2100 Bioanalyzer (Agilent Technologies, Santa Clara, CA, USA) was connected to the Protein 80 Plus LabChip pack. The dedicated Protein 80 software assay on 2100 expert software was utilized for the chip-based separations. The Protein 80 LabChip pack was used as a protocol for setting up the chips. The fractioning is size-based, and the observed profiles show the smallest proteins, which emerge at the bottom of the gel patterns, according to the convention for SDS-PAGE (Torbica et al., 2010). Bovine serum albumin was utilized as the standard for the quantification of the milk proteins. All samples were analysed in triplicate.
Determination of calcium (Ca) content
The concentration of Ca in milk was determined by the atomic absorption spectrometer (Varian, SpectrAA 10, Australia), after processing with Milestone Ethos-10 microwave digestor. All samples were analysed in triplicate.
Antibacterial assay
Preparation of inoculum
The antibacterial properties of the DM were tested against clinical isolate of K. pneumoniae obtained from patient with pneumonia. The bacterial strain was provided by Institute for Pulmonary Diseases of Vojvodina, Sremska Kamenica, Serbia. The clinical isolate of K. pneumoniae was pre-cultured on nutrient agar (Himedia, India) overnight at 37 °C. Afterward, the density of the bacterial suspension was adjusted to turbidity equal to the 0.5 McFarland standard using a DEN-1 densitometer (Biosan, Riga, Latvia). A ten-fold sequential dilutions of the bacterial suspension were prepared in 0.1% peptone saline (Šarić et al., 2012).
Test at 9 °C and 15 °C
Nine of the seventeen DM samples (25 mL each) were intentionally contaminated with K. pneumoniae at 10 2, 10 3 and 10 4 cfu/mL LCs using appropriate dilutions of the bacterial suspension. Tested DM samples were dispensed into a sterile vessels and incubated at 9 °C and 15 °C for 96 h. Changes in the number of K. pneumoniae were monitored every 24 h.
Test at 38 °C
The remaining eight DM samples (25 mL each) were intentionally contaminated with K. pneumoniae at the LC of 10 2 cfu/mL. Tested DM samples were dispensed into a sterile vessels and incubated at 38 °C for 8 h. Changes in the number of K. pneumoniae were monitored every hour.
The Violet Red Bile Glucose Agar (VRBGA) (Himedia, India) was used for the enumeration of K. pneumoniae in intentionally contaminated DM samples in all tests (9, 15 and 38 °C). Inoculated plates of VRBGA were incubated at 37 °C for 24±3 h. The number of viable K. pneumoniae was expressed as log cfu/mL. Intentionally contaminated nutrient broth (Himedia, India) was used as a positive control, while non-inoculated DM was used as a negative control. All samples were analysed in triplicate.
Statistical analysis
The obtained data were statistically processed using the software package STATISTICA 10.0 (StatSoft Inc., Tulsa, OK, USA). All determinations were done in triplicate; all data were averaged, and expressed as mean values ± standard deviation (SD). Analysis of variance (ANOVA) and Tukey's HSD test for comparison of means were used to analyse the variations of the number of K. pneumoniae in DM and nutrient broth (NB), with the variations in the LC, T, and t. The principal component analysis (PCA) was used to discover the possible correlations between measured parameters, and to classify the objects.
The second order polynomial (SOP) models in the following equation were developed to relate responses (Y) and two process variables (X):

where: β k0, β ki, β kii, β kij were constant regression coefficients; Y k the number of K. pneumoniae in DM ( Y 1 ) or NB ( Y 2 ), while X 1 is the LC, X 2 is T, X 3 is the t, X 4 is the LYZ content, X 5 is the LF content and X 6 is the Ca content. In this article, ANOVA was conducted to show the significant effects of independent variables on the responses and to show which of responses were significantly affected by the varying treatment combinations.
The multi-layer perceptron model (MLP), with three layers (input, hidden and output) was applied to ANN model calculation (Arsenović et al., 2013). This form of the ANN model is widely recognized and chosen due to its high capability of approximating nonlinear functions (Turanyi and Tomlin, 2014). The MLP neural network learns using an algorithm called „back propagation“. Levenberg–Marquardt algorithm is proven to be the fastest and also particularly adapted for networks of moderate size. The experimental database is randomly divided into three groups for ANN model development: training data (60 %), cross-validation (used to test the performance of the network while training) (20 %) and testing data (used to examine the network generalization capability) (20 %). Before the ANN calculation, input and output data were normalized (Min-Max normalization method was used in this paper) in order to improve the prediction of the ANN (Pezo et al. 2013). During the calculation of the ANN, input data are repeatedly introduced to the network (Grieu et al., 2011). The training process of the network was repeated 100,000 times, testing the different topologies of ANN, with a different number of neurons in the hidden and the output layers (5-20), different activation functions (such as logarithmic, logistic, tangent hyperbolic, or identity), and with random initial values of weight coefficients and biases. The optimization of the ANN structure was performed by minimizing the validation error. Broyden-Fletcher-Goldfarb-Shanno (BFGS) algorithm was utilized, for finding the solution of the unconstrained nonlinear optimization during the ANN modelling (Pezo et al. 2013).
Results and discussion
The results of the antibacterial assays performed at 9 and 15 °C are presented in Tables 1 and 2.
Тable 1. The count of K. pneumoniae (log cfu/mL) in intentionally contaminated DM samples during 96 h of incubation at 9 °C
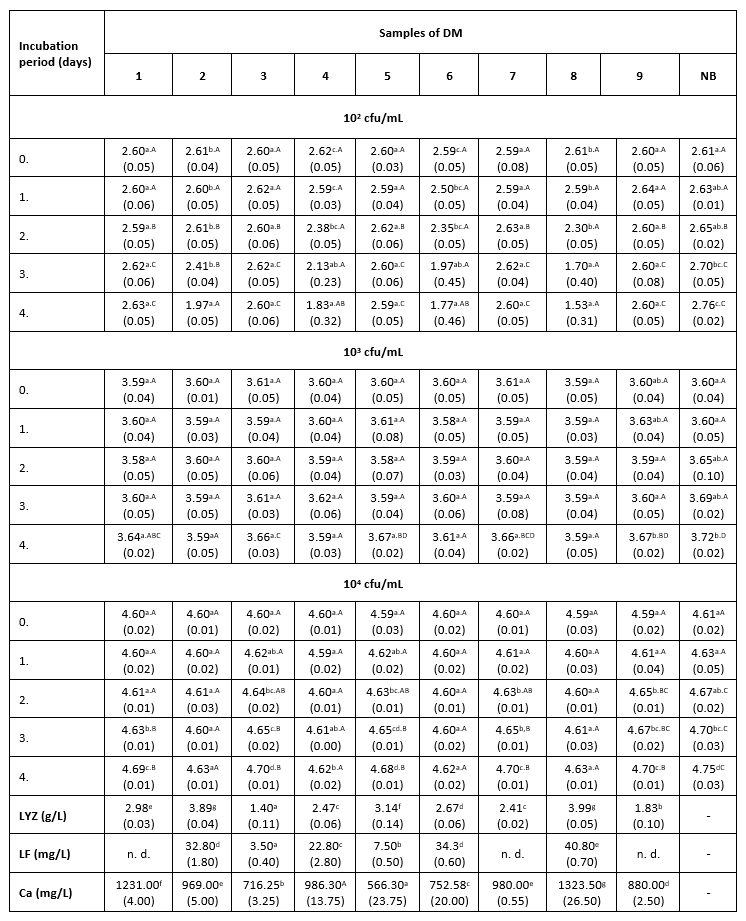
*Mean values of the observed parameters are written as the result of three measurements (n=3). Standard deviation values are given in parentheses. Statistical significance is considered by the column but separately by the number of bacteria (10 2 cfu / mL, 10 3 cfu / mL, 10 4 cfu / mL). Different letters written in the lowercase show statistically significant different means in columns of the observed data (p<0.05), according to post hoc Tukey’s HSD test. Different letters written in the uppercase show statistically significant different means in rows of the observed data (p<0.05), according to post hoc Tukey’s HSD test. NB-nutrient broth; n.d.-not detected.
The results of the antibacterial assay performed at 9 °C show low antibacterial activity of the examined DM samples against the tested clinical isolate of K. pneumoniae (Table 1). The antibacterial activity of DM caused a slightly slower growth of K. pneumoniae in comparison with the growth in the positive control. A more distinct antibacterial activity in all three LCs was observed in samples 2, 4, 6 and 8, which had a higher LF content (22.8 to 40.8 mg/L) and a relatively high concentration of LYZ (2.47 to 3.99 g/L). The presence of Enterobacteriaceae was not detected in any of the negative control samples.
The results of the antibacterial assay performed at 15 °C are presented in Table 2. The bactericidal activity of DM was noticed only at the lowest LC and only in samples with the highest LF concentration (Table 2). The antibacterial activity of DM samples with an LC of 10 2 cfu/mL in which LF was not detected or was present at low concentrations (3.5-7.5 mg/L), consisted of deceleration of K. pneumoniae growth to the end of incubation.
In samples with the highest content of the LF, at the intermediate LC (10 3 cfu/mL), a decrease in the number of tested bacteria was observed to the end of the incubation. In samples 7 and 9, the lag phase was extended for 24 hours compared to growth in nutrient broth. After that, a decline of the antibacterial activity in these milk samples was observed, but not until its complete cessation, considering that the number of K. pneumoniae to the end of incubation increased much faster in nutrient broth (Table 2).
Тable 2. The count of K. pneumoniae (log cfu/mL) in intentionally contaminated DM samples during 96 h of incubation at 15 °C
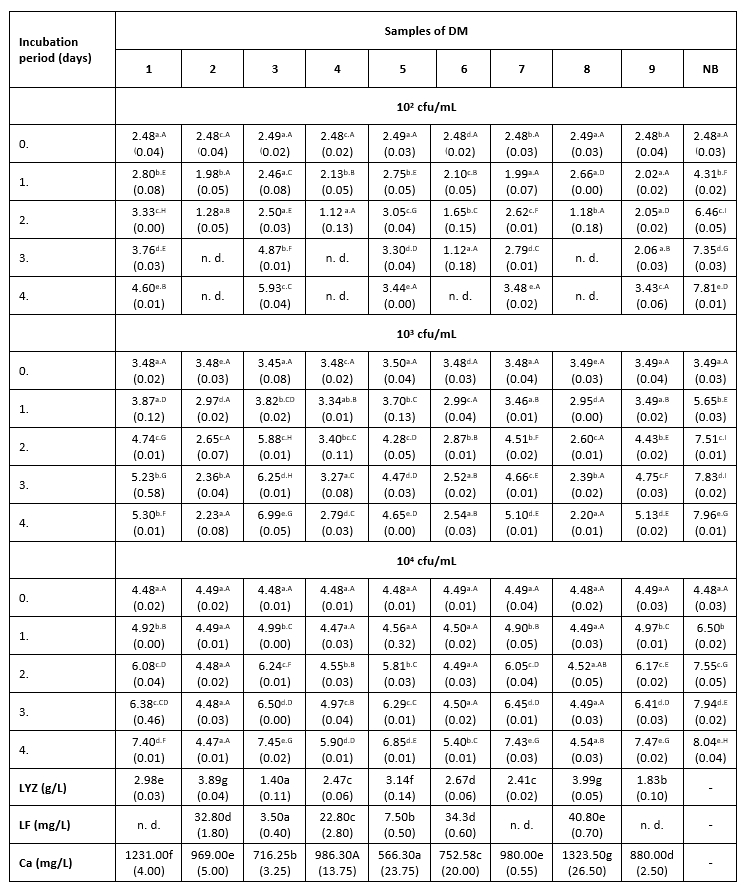
*Mean values of the observed parameters are written as the result of three measurements (n=3). Standard deviation values are given in parentheses. Statistical significance is considered by the column but separately by the number of bacteria (10 2 cfu / mL, 10 3 cfu / mL, 10 4 cfu / mL). Different letters written in the lowercase show statistically significant different means in columns of the observed data (p<0.05), according to post hoc Tukey’s HSD test. Different letters written in the uppercase show statistically significant different means in rows of the observed data (p<0.05), according to post hoc Tukey’s HSD test. NB-nutrient broth; n.d.-not detected.
At the intermediate LC, the correlation between the LYZ content and the antibacterial activity of DM samples was very pronounced. Thus, sample 3 with the lowest content of this protein (1.4 g/L) showed the weakest antibacterial activity against K. pneumoniae. The strongest antibacterial activity (at LCs of 10 3 and 10 4 cfu/mL) was measured in samples 2 and 8 (Table 2), in which the high concentrations of LF and the highest content of LYZ were observed (Table 2). In samples with lower content of LF (3.5-7.5 mg/L), the strongest antibacterial activity was recorded during the first 24 hours of incubation, during a slight increase in the number of test microorganisms. After 24 hours, the number of K. pneumoniae begun to increase, suggesting that these milk samples no longer exhibited their antibacterial activity. The presence of Enterobacteriaceae was not detected in the negative controls.
The dependence between the antibacterial activity of milk samples to K. pneumoniae and Ca content in these samples during the experiment at 9 and 15 °C was not determined. The results of the antibacterial assay performed at 38 °C are presented in Table 3.
Table 3. Changes in K. pneumoniae count (log cfu/mL) in DM during 8 h at 38 °C
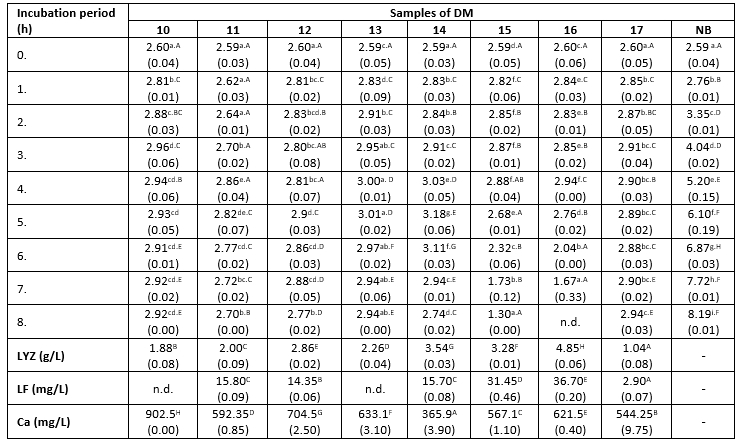
*Mean values of the observed parameters are written as the result of three measurements (n=3). Standard deviation values are given in parentheses. Statistical significance is considered by the column but separately by the number of bacteria (10 2 cfu / mL, 10 3 cfu / mL, 10 4 cfu / mL). Different letters written in the lowercase show statistically significant different means in columns of the observed data (p<0.05), according to post hoc Tukey’s HSD test. Different letters written in the uppercase show statistically significant different means in rows of the observed data (p<0.05), according to post hoc Tukey’s HSD test. NB-nutrient broth; n.d.-not detected.
The count of K. pneumoniae during 8 hours of incubation at 38 °C changed following the similar trend in all tested samples (Table 3). This count increased from the start of the incubation period until the fourth or fifth hour (significantly lower growth rate compared to the nutrient broth), and subsequently began to decline. However, the differences in antibacterial activity between the tested samples were obvious, indicated by differences in the recorded counts of tested bacteria after 8 hours of incubation. The relationship between the degree of reduction in the count of K. pneumoniae and the Ca content in milk samples has been not established in this assay. For example, samples 15 and 16 exhibited a stronger antibacterial effect compared to sample 10, even though it has nearly twice higher Ca content (Table 3). The strongest antibacterial effect was observed in the sample with the highest content of LYZ (Table 3). In contrast to that, the reduction degree of the tested strain of K. pneumoniae was directly proportional to the content of LF in the DM samples. At the end of the experiment, the highest count of tested pathogens was detected in samples with LF content below the limit of detection, or present in low concentrations (2.9 mg/L). The lowest count of K. pneumoniae was observed in samples with the highest concentration of this LF (Table 3). The presence of Enterobacteriaceae was not detected in the negative controls.
ANOVA shows significantly independent variables as well as interactions of these variables. The ANOVA test shows the significant effects of the independent variables on the responses and which of responses were significantly affected by the varying treatment combinations (Table 4).
Table 4. ANOVA table of the K. pneumoniae count in donkey milk (DM) and nutrient broth (NB evaluation (sum of squares)
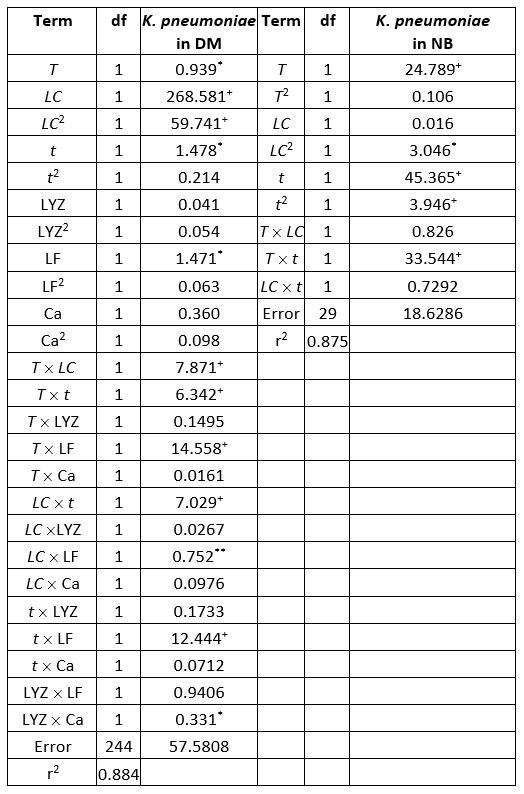
+Significant at p<0.01 level, *Significant at p<0.05, level 95 % confidence limit, error terms have been found statistically insignificant, df - degrees of freedom
Evaluation of the number of K. pneumoniae in DM, in the SOP model, was mostly affected by the linear term of the LC, and also the quadratic term of the LC, statistically significant at p<0.01 level. The influence of the linear terms of T, t, and LF content was also observed, but the impact was much lesser compared to the LC, statistically significant at p<0.05 level. The interchange terms T × LC, T × t, T × LF, LC × t, and t × LF also showed the influence on determination of K. pneumoniae in DM, statistically significant at p<0.01 level. The linear term of the experiment duration was the most influential variable in the SOP model for the prediction of the number of K. pneumoniae in NB. At the same time, the linear term of T and the interchange term T × t and the quadratic term of t were also important and statistically significant at p<0.01 level. The quadratic term of the LC was found influential in the SOP model to evaluate the number of K. pneumoniae in NB, statistically significant at p<0.05 level. During experiments, SOP models representing the number of K. pneumoniae in DM and NB during the experiments had an insignificant lack of fit tests. T, the LC, the t, and LF content were found statistically significant, while the predicted and observed responses corresponded well with coefficients of determination in the range of 0.875-0.885. The developed models were used for graphical representation of DM and NB in the three-dimensional coordinate system (Figure 1).
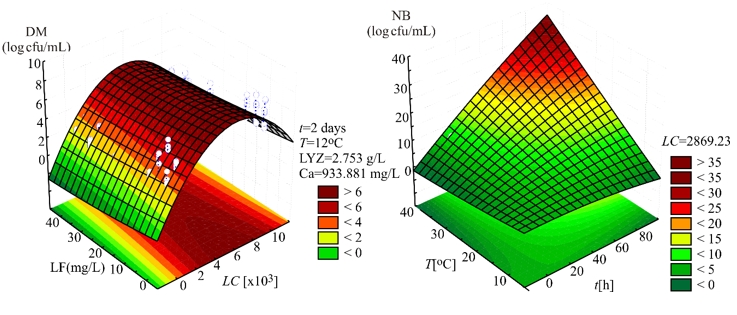
Figure 1. Three-dimensional surface plots of donkey milk (DM) and nutrient broth (NB)
Broyden-Fletcher-Goldfarb-Shanno (BFGS) algorithm, implemented in StatSoft Statistica’s evaluation routine, was used for the ANN modeling. The optimization procedures to minimize the error function between the network and experimental outputs were used during the ANN training cycle (Pezo et al., 2013; Taylor et al., 2006), and the sum of squares (SOS) was evaluated according to the BFGS algorithm, to speed up and stabilize the convergence of the results (Basheer et al., 2000). The training process was repeated several times to get the best performance of the ANN, due to a high degree of variability of parameters. It was accepted that successful training was achieved when the learning and cross-validation curves (SOS vs. training cycles) approached zero. The coefficient of determination (r 2) and SOS were used as parameters to check the performance (i.e. the accuracy) of the obtained ANN. The optimum number of hidden neurons was chosen upon minimizing the difference between predicted ANN values and desired outputs, using SOS during testing as a performance indicator. According to ANN performance (sum of r 2 and SOSs for all variables in one ANN), it was noticed that the optimal number of neurons in the hidden layer for DM calculation is 11 (network MLP 6-11-1), with observed training performance 0.986 and training error 4.67∙10 -4 (the optimal network for NB calculation was MPL 3-10-1, training performance was 0.982 and training error was 0.002). It can be seen that these r 2 values for SOP models (Table 4) are very much similar to those associated with the ANN model. These findings agree with other authors (Basheer et al., 2000; Pezo et al., 2013). Although ANN models are more complex (51-89 weights-biases for DM and NB calculation) than SOP models, ANN models performed somewhat better because of the high nonlinearity of the developed system (Pezo et al., 2013).
In order to assess the effect of changes in the outputs due to the changes in the inputs, sensitivity analysis was performed. The greater effect observed in the output implies a greater sensitivity concerning the input (Pezo et al., 2013). Sensitivity analysis has been performed to test an infinitesimal change in an input value in 10 equally spaced individual points, in the range between the minimum and maximum of the observed assay to explore the observed outputs’ changes. It is also used to test the model sensitivity and measurement errors. The influence of the input over the output variables, i.e., calculated changes of output variables for infinitesimal changes in input variables, is shown in Figure 2.
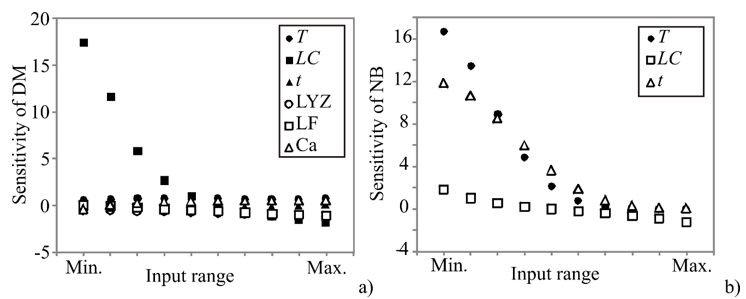
Figure 2. Sensitivity analysis - the influence of the input over the output variables: a) DM and b) NB
The obtained values corresponded to the level of experimental errors, and have also shown the T, LC, t, LYZ, and LF influence on the count of K. pneumoniae in DM, as well as the influence of T, LC, and t on the count of K. pneumoniae in NB. According to Figure 2, the count of K. pneumoniae in DM was mostly influenced by LC, which was also confirmed by ANOVA analysis of the SOP model. The count of K. pneumoniae in NB was mostly influenced by T and t variables. Sensitivity analysis is used to show the influence of the inputs, but it also shows the importance of an input variable at a given point in the input space (Saltelli and Annoni, 2010). As presented in Figure 2, the count of K. pneumoniae in DM samples was more strongly affected by the infinitesimal changes of LC, at the minimum of the input range, while the count of K. pneumoniae in NB was mostly affected by T and t at the minimum of the input range. These findings are in accordance with ANOVA analysis, as well as with the experimental measurements.
There are many different factors that affect milk composition such as genetic factors, nutrition and stage of lactation (Lika et al., 2021; Ugarković et al., 2020). The measured differences in the content of LYZ, LF and Ca (Tables 1-3) might be caused by the effects of the lactation period of milking animals (Gubić et al., 2014; Gubić et al., 2016; Salimei and Fantuz, 2012) since the donkeys included in this study were in different lactation stages. In donkey milk, the LYZ content seems to decrease during lactation (Gubić et al., 2016; Polidori and Vincenzetti, 2010; Vincenzetti et al., 2017). In general, the obtained values of LYZ, LF and Ca were in the range of previously reported concentrations in DM (Coppola et al., 2002; Salimei and Fantuz, 2012; Vincenzetti et al., 2008).
As reported in the literature, the major antimicrobial agent in DM is lysozyme ( LYZ), followed by lactoferrin ( LF) (Coppola et al., 2002; Martini et al., 2019; Tidona et al., 2011; Vincenzetti et al., 2008; Zhang et al., 2008). LYZ likely plays a major role in the antibacterial activity of DM against the clinical isolate of K. pneumoniae tested at 9, 15 and 38 °C, with regard to much higher concentrations of LYZ compared to LF (Tables 1-3). According to results obtained at 9 and 15 °C, antibacterial activity of DM toward K. pneumoniae depended on the content of LYZ and LF as well as on the LC, T, and t. A lower level of tested K. pneumoniae obtained after 96 hours of incubation at 9 °C compared to 15 °C does not mean a stronger antibacterial activity of DM at 9 °C, and it may be explained by a higher growth rate of K. pneumoniae at 15 °C. This is evident when the growth of these bacteria is compared in nutrient broth at 9 °C and 15 °C. Actually, the temperature of 15 °C was proven to be more favourable for the antibacterial activity of DM compared to 9 °C. This finding was most evident in tests with the lowest LC (10 2 cfu/mL), where K. pneumoniae in four DM samples was not detected after 72 hours of incubation at 15 °C (Table 2). The increase in the LC led to a faster consumption of the antibacterial capacity of DM. Stronger antibacterial activity of DM at 15 °C could be caused by an increased activity of LYZ and LF at a higher T. According to literature data, the minimum temperature at which LYZ in DM exhibited antibacterial (enzymatic) action was 15 °C (Mariani et al., 2001). However, due to a certain drop in growth intensity of K. pneumoniae in DM which was demonstrated at 9 °C, it is evident that the antibacterial components of donkey milk were also active at this temperature. The decrease in K. pneumoniae count at 9 °C can as well be attributed to the smaller size of bacterial cells at lower temperatures. As Johansen et al. (1994) already concluded, . the smaller size of bacterial cells causes a lower amount of biomass for the same number of cells, making them more sensitive to LYZ.
Despite all that, a clear conclusion cannot be made on a direct correlation between the content of LYZ and the degree of reduction of pathogens tested under these experimental conditions. According to the available literature sources, there is no data on the antibacterial activity of DM or its LYZ or LF toward K. pneumoniae. On the other hand, there are few reports of the bactericidal effect of other LYZs against K. pneumoniae, including equine LYZ (Brumini et al., 2013; Martini et al., 2019). The antibacterial activity of LYZ is primarily directed against Gram-positive bacteria and is related to its ability to cause bacterial lysis by catalysing hydrolysis of the 1,4-b-linkages between N-acetylmuramic acid and N-acetylglucosamine in peptidoglycan, which is the major component of the Gram-positive bacterial cell wall (Alhazmi et al., 2014). Due to its activity, LYZ contributes to the low bacterial count of raw donkey milk. On the other hand, Gram-negative bacteria appear to be less sensitive to LYZ owing to the protective role of the outer membrane, and the mechanism of its antibacterial activity against Gram-negative bacteria has not been completely elucidated. Taking into account that the samples of DM with higher LF content showed a stronger antibacterial effect against K. pneumoniae (Tables 2 and 3), the antibacterial synergism of LYZ and LF was most likely expressed to this clinical strain. According to Martini et al. (2019) LYZ can act synergistically with LF against Gram-negative bacteria. (More precisely, LF can interact with the lipopolysaccharide LPS layer of the outer membrane of Gram-negative bacterial cell causing its disruption and enhance susceptibility of Gram-negative bacteria to LYZ (Farnaud and Evans, 2003). According to previous investigations, human LF possesses the ability to directly bind to the lipid A LPS in the outer layer membrane of K. pneumoniae through its cationic zone (Appelmelk et al., 1994). These actions result in an inhibition or a complete cessation of bacterial growth. The DM samples with low LF content as well as those samples in which this protein was below the limit of detection of the method still showed antibacterial activity (Tables 2 and 3). It could be due to the fact that the saturation of the lipid in A LPS layer of K. pneumoniae required only low concentrations of LF. Depending on the strain of K. pneumoniae, these concentrations were in the range of 0.125 to 1.0 mg/L (Appelmelk et al., 1994). The absence of dependence between the content of Ca in DM samples and their antibacterial activity established in this study can indicate that the determined inhibition of K. pneumoniae growth in DM is not a consequence of the LYZ transformation to the linear protofilaments as was probably the case in previous investigations with E. coli, S. enteritidis and S. typhimurium in DM (Šarić et al., 2014a; Šarić et al., 2017). The emphasis of this in situ study was on previously assigned main DM antimicrobial agents ( LYZ and LF) and Ca content due to its reported contribution to antibacterial activity of DM against some members of Enterobacteriaceae family (Šarić et al., 2014a; Šarić et al., 2017). However, DM represents a very complex medium and the method used for antibacterial testing does not allow determining the contribution of other compounds in DM to its total antibacterial activity. Previous investigations have shown that the fatty acids with reported antibacterial activity might play an important role in the overall antibacterial activity of DM due to their high share in its overall fatty acids profile (Derdak et al., 2020; Massouras et al., 2017; Šarić et al., 2014b). Besides that, LYZ can acts in synergy with some other milk components such as lactoperoxidase, N-acetyl-b-D-glucoaminidase, immunoglobulins and some fatty acids (Derdak et al., 2020; Martini et al., 2019). It has been reported that LYZ can form complex with oleic acid with bactericidal action called ELOA (Vincenzetti et al., 2017). Furthermore, other studies have shown that e LF as a minor component in DM can be easily digested by gastric and duodenal juice. Thus, it has been suggested that LF can play its biological role in vivo mainly through its bioactive peptides derived from digestion (Martini et al., 2021). Recent studies also showed that antimicrobial activity of LF was further increased after in vitro simulated gastrointestinal digestion (Martini et al., 2021). Similarly, it was suggested that α-lactalbumin possesses bactericidal activity only after its partial digestion by endopeptidases (Vincenzetti et al., 2017).
Although the results obtained in this study showed that DM could be considered as a valuable potential natural agent in the treatment of bacterial pneumonia, additional in vivo investigation on DM antibacterial activity against K. pneumoniae is required in order to elucidate its health benefits.
Conclusion
The antibacterial activity of donkey’s milk against K. pneumonia observed in this study was found to be incubation temperature and level of contamination dependent. Thereby a stronger antibacterial activity of donkey milk at higher incubation temperature was obtained in the initial phase of the incubation period. However, a faster weakening of this antibacterial activity after this period was probably caused by the intensive multiplication of tested bacteria at higher incubation temperature. The higher initial level of contamination caused a rapid consumption of the antibacterial capacity of donkey’s milk. Although lysozyme is marked as the major antibacterial agent in donkey’s milk due to its high concentration, a statistically significant correlation of antibacterial activity of donkey’s milk and lactoferrin content indicated that synergistic activity of lysozyme and lactoferrin is the mechanism of antibacterial activity of donkey milk towards the tested clinical isolate of K. pneumoniae. The results obtained in this study indicate the need for clinical trials which could lead to new therapeutic applications of donkey milk in the prevention and cure of bacterial pneumonia.
Acknowledgments
Authors are grateful to Slobodan Simić and Nikola Nilić (Special Nature Reserve "Zasavica", Serbia) for providing the milk samples.
Funding
This work was financially supported by the Provincial Secretariat for Higher Education and Scientific Research, Autonomous Province of Vojvodina, Republic of Serbia [grant number 142-451-2637/2021-01/2] as a part of the project entitled "Examination of the impact of goat, mare and donkey milk on the recovery of patients with pneumonia".
Antibakterijsko djelovanje mlijeka magarice na klinički izolat Klebsiella pneumoniae
Sažetak
Svrha ovog istraživanja bila je utvrditi antibakterijsko djelovanje sirovog mlijeka magarice prema kliničkom izolatu Klebsiella pneumoniae pri 9, 15 i 38 °C, te razjasniti ulogu lizozima, laktoferina i kalcija u antibakterijskom djelovanju mlijeka magarice. Također su ispitani učinci razine kontaminacije i razdoblja inkubacije na navedenu antibakterijsku aktivnost. Antibakterijski testovi provedeni su određivanjem broja bakterija u ciljano kontaminiranim (10 2, 10 3, 10 4 cfu/mL) uzorcima mlijeka magarice tijekom 8 i 96 sati. Lab-on-a-chip elektroforeza i atomska apsorpcijska spektrometrija korištene su za određivanje sadržaja lizozima, laktoferina i kalcija u mlijeku magarice. U ispitivanim uzorcima mlijeka magarice utvrđeni su različiti stupnjevi antibakterijske aktivnosti prema ispitivanom soju K. pneumoniae. Za ovaj klinički soj dokazan je antibakterijski sinergizam lizozima i laktoferina, jer su uzorci s većom količinom laktoferina pokazali jače antibakterijsko djelovanje. Korelacija između sadržaja kalcija i antibakterijske aktivnosti uzoraka mlijeka magarice nije utvrđena. Mlijeko magarice pokazalo je jači antibakterijski potencijal na 15 °C u usporedbi s antibakterijskim potencijalom na 9 °C, ali je on bio ograničen većom stopom rasta K. pneumoniae na 15 °C. Viša razina kontaminacije uzrokovala je bržu potrošnju antibakterijskog kapaciteta mlijeka magarice. Model umjetne neuronske mreže za predviđanje broja K. pneumoniae dobro se slagao s eksperimentalnim podacima (ukupni r 2 za mlijeko magarice bio je 0,986, s pogreškom učenja od 4,67 10 -4, dok je r 2 za hranjivi medij bio 0,982, a pogreška učenja bila je 0,002).
Ključne riječi: mlijeko magarice; antibakterijsko djelovanje; Klebsiella pneumoniae
References
https://doi.org/10.3390/foods9091302.
https://doi.org/10.15567/mljekarstvo.2015.0303
Šarić, Ć.L., Šarić, M.B., Mandić, I.A., Kevrešan, S.Ž., Ikonić, B.B., Kravić, Ž.S., Jambrec, J.D. (2014a): Role of calcium content in antibacterial activity of donkeys’ milk toward E. coli. European Food Research and Technology 239, 1031-1039. https://doi.org/10.1007/s00217-014-2299-4