INTRODUCTION
Elderberry ( Sambucus nigra L.) is a seed-propagated perennial deciduous shrub or small tree with weak roots. It blooms from May to June (1). All plant parts of black elderberry (leaves, berries, inflorescences, roots, shoots, bark) have a long history of usage in herbal medicines or a variety of culinary applications and are found on practically every continent (2).
Elderberries and elderflowers are most commonly utilized in the food sector as food colorants, juices, and flavoring agents (3). Dried elder blossoms ( Sambuci flos) are most commonly used in tea preparations (4), but they are also utilized in a variety of cosmetics and therapeutic items (5). S. nigra leaves are utilized for cutaneous therapy (3). Elderberry flowers and fruits are high in bioactive compounds with antioxidant capabilities, but they also have vital minerals like calcium and magnesium (6) and exhibit antioxidant (6), antidiabetic, anti-inflammatory, immunostimulating, chemopreventive (3), and antiviral properties (7), according to pharmacological studies.
The components found in medicinal plants may contribute to the documented therapeutic effects of these plants, therefore, it is crucial to determine their levels in herbal plants (8). The presence of heavy metals in the soil or changes in the availability and distribution of metalloids serving as plant nutrients cause numerous adaptation mechanisms in plants (9). Plants are the primary conduits for heavy metals from contaminated soil to humans. Heavy metals tend to accumulate in the food chain (10).
While the chemical composition of elderberries has been comprehensively studied with a lot of applications, there is still not enough information on leaves and flowers from the Kosovo region concerning the mineral content. In this regard, the purpose of this study was to use inductively coupled plasma-atomic emission spectrometry (ICP-AES) and inductively coupled plasma-mass spectrometry (ICP-MS) methods to assess the mineral content of S. nigra leaves and flowers in different locations.
EXPERIMENTAL
Plant material
Plant material (leaves and flowers of S. nigra) was collected in a flowering stage in the period of May–June 2021 from eleven different locations in Kosovo. The symbol, location and collection data for the plant material: SN-1 (Klinë), SN-2 (Istog), SN-3 (Vitomiricë), SN-4 (Pejë), SN-5 (Skivjan), SN-6 (Deçan), SN-7 (Gjakovë), SN-8 (Suharekë), SN-9 (Ferizaj), SN-10 (Graçanicë), and SN-11 (Podujevë). Plant materials were air-dried and stored in a paper box until analysis.
Chemicals
For this work, the following reagents were used: conc. nitric acid (69 %, m/ V), trace pure (Merck, Germany), and hydrogen peroxide (30 %, m/ V), p.a. (Merck). All solutions were prepared using redistilled water.
Diluting 1000 mg L–1 solutions (11355-ICP multi Element Standard, Merck) yielded metal standard solutions.
Digestion and chemical analysis
After cleaning the plant samples (leaves and flowers), they were ground by hand using gloves and dried at 40 °C for 48 h. Samples were digested in a microwave system by the wet digestion method. Sample (0.5 g) was put in the Teflon tube to which 7 mL of nitric acid and 2 mL of hydrogen peroxide were added. The mixture was left to react for 10 minutes to allow time for reactions to occur and the gases to be released, resulting in less pressure in the Teflon tube during microwave digestion. Then Teflon tubes were put in the Mars microwave digestion system (CEM, USA) with the following program: ( i) temperature 180 °C, 20 min ramp time, with power of 800 W; ( ii) temperature 180 °C, 25 min on hold time, with a power of 800 W. After digestion the obtained solutions were filtered, poured in 25-mL plastic flasks and the rest of the vessel was filled with redistilled water and sent for elements chemical analysis.
The application of inductively coupled plasma-atomic emission spectrometry (ICP-AES) and inductively coupled plasma-mass spectrometry (ICP-MS) was used to determine the following 31 elements: Ag, Al, As, B, Ba, Be, Bi, Ca, Cd, Co, Cr, Cs, Cu, Fe, Ga, Hg, In, K, Li, Mg, Mn, Na, Ni, P, Pb, Rb, Sr, Tl, U, V, and Zn.
ICP-AES (Varian, model 715ES, USA) was used for the analysis of Al, B, Ba, Ca, Cr, Cu, Fe, K, Mg, Mn, Na, P, Sr, V, and Zn. The QC/QA of the applied technique was performed by the standard addition method, and it was found that the recovery for the investigated elements ranges for ICP-AES between 98.5–101.2 %. Quality control was further assured using standard moss reference materials M2 and M3, which were generated for the European Moss Survey (11). The measured concentrations were in good agreement with the recommended values. The difference between the measured and certified values was within 10 %.
Sixteen isotopes7Li,9Be,59Co,60Ni,79Ga,755As,855Rb,107Ag,114Cd,115In,133Cs,202Hg,205Tl,209Bi,238U, and Pb including the isotopes206Pb,207Pb,208Pb were determined using inductively coupled plasma mass spectrometry (Plasma Quant ICP-MS, Analytic Jena, Germany) for the analysis of the content of following trace elements: Ag, As, Be, Bi, Cd, Co, Cs, Ga, Hg, In, Li, Ni, Pb, Rb, Tl, and U.
External calibration was accomplished by measuring standard solutions containing these elements at the concentrations of 0.5, 1, 2, 5, 10 and 100 μg L–1 for the following isotopes:7Li,49Ti,59Co,65Cu,75As,98Mo,107Ag,114Cd,118Sn,121Sb,205Tl and Pb (206Pb,207Pb,208Pb). External calibration for202Hg was accomplished by measuring standard solution with the concentration of 0.5, 1, 2, 5, and 10 μg L–1. No internal standards were used.
Detector attenuation mode was used to cover a wide range of linearity without the collision gas. In another step, He was utilized as a collision gas for troublesome isotopes such as60Ni and78Se. External calibration was used to determine the concentration of60Ni in the range of 1, 5, 10, 50, 100, and 500 g L–1.78Se was determined in the range of 1–50 µg L–1.
Detection and quantification limits were calculated automatically by the instrument software (Aspect MS 4.3, 2017). Standard solutions were measured in the sample sequence after every 20 samples to ensure sensitivity and repeatability. Recoveries of the analyzed elements ranged from 82.1–105.1 %.
RESULTS AND DISCUSSION
The content of macroelements and microelements
In this study, a total of 31 elements were determined (15 elements by the ICP-AES method and 16 elements by the ICP-MS method). Concentration levels of all measured elements are shown in Table I for leaves and in Table II for flowers of Sambucus nigra.
Table I. The mineral content of Sambucus nigra leaves (SN-LE)
ICP-AES – inductively coupled plasma-atomic emission spectrometry), ICP-MS – inductively coupled plasma-mass spectrometry), SN-LE – Sambucus nigra leaf
a Dry mass basis.
Table II. The mineral content of Sambucus nigra flowers (SN-FL)
ICP-AES (Inductively coupled plasma – atomic emission spectrometry), ICP-MS (Inductively coupled plasma – mass spectrometry), SN-FL – Sambucus nigra flower)
a Dry mass basis.
The macroelements K, Ca, Mg, and P determined by the ICP-AES method were dominant elements in all analyzed samples of S. nigra (Tables I and Table II). A higher content of K, Ca, and Mg was found in the leaves compared to the flowers, whereas the flowers were characterized by a higher content of phosphorus. The content of macroelements in the flowers was according to this sequence K > Ca > P > Mg, while in leaves it was K > Ca > Mg > P. These are commonly generated from soil (12). Potassium was the dominant element in all analyzed samples. Our findings agreed well with those of Imenšek et al. (13).
Metals such as Zn, Cu, Fe, Mn, and Cr are vital elements that are required for the human body's physiological and biological activities (13). Zn and Cu are critical minerals for human, animal, and plant growth and play important roles in a variety of metabolic processes (14). The content of microelements in Sambucus nigra varied, depending on the plant part and locality. Microelements B, Cu, Fe, Mn, Na, Sr, and Zn were determined by the ICP-AES method. Their content in the flowers of S. nigra followed this order: Fe > Mn > Na > Zn > B > Cu > Sr, while in the leaves it was: Fe > Mn > Na > Zn > B > Sr > Cu. When comparing flowers and leaves, higher contents of Cu and Na were found in flowers, while higher amounts of B, Mn, Sr, and Zn were found in leaves.
Specific was the presence of Fe, where in most of the leaf samples analyzed it was in a higher content (111–205 mg kg–1) and the lowest in the flowers (57–165 mg kg–1), with the exception of sample SN10-FL flower where Fe content was much higher (422 mg kg–1). Our results for Fe are higher compared to those reported by Imenšek et al. (13). The discrepancy in Fe content may mainly be due to the geological formations that predominate in that area (15). Microelements Bi, Co, Ga, Li, Ni, and Rb were determined using ICP-MS. When comparing the leaves and flowers of S. nigra, higher contents of Ni, Co, and Bi were found in the leaves, while higher content of Rb, Li, and Ga was found in the flowers.
The content of potentially toxic elements
From potentially toxic elements (PTE), Al, Ba, Cr, and V were determined by ICP-AES and Ag, As, Cs, In, and Pb by ICP-MS. In most of the leaf samples, the content of Al was higher than in flowers (75–145 mg kg–1 vs. 28.6–114 mg kg–1), except in the flower of sample SN10-FL; here Al content was much higher (320 mg kg–1), similarly to the case of Fe. High concentration of Al is usually found in acid soils, which are characterized by a lack of nutrients and toxicity from metals such as Mn, Fe, and Al; Al toxicity is the main factor that limits plant growth in these soils (16).
The content of As and Pb in the leaves was higher (0.05–0.2 mg kg–1 and 0.38–0.94 mg kg–1, resp.) compared to the flowers (0.01–0.06 mg kg–1 and 0.11–0.47 mg kg–1, resp.) of S. nigra, except sample of flower SN-10 from Graçanica where the content of As and Pb was tens of times higher in the flowers (0.64 mg kg–1 and 3.58 mg kg–1, resp.). In all the analyzed samples, the content of Pb was below the limit allowed according to Ph. Eur. (5.0 mg kg–1 for Pb) (17).
Be, Cd, Hg, Tl, and U were determined by the ICP-MS method. Their concentration was higher in leaves than in flowers, except for sample SN10-FL where the content of Be, Tl, and U was several times higher in flower. Cd is a highly toxic metal that has a detrimental effect on plant development and productivity (18). Cd content was below permissible limits according to Ph. Eur. (1.0 mg kg–1) (17).
When considering the morphological features of the plants, the leaves contained the largest amount of Hg (19). The content of Hg was higher in the analyzed leaves compared to the flowers of S. nigra (11.2–41.4 µg kg–1 and 5.83–13.25 µg kg–1, resp.). Due to the significant toxicity of Hg, it should not exceed 0.1 mg kg–1 according to Ph. Eur. (17). The content of Hg found in the present study was below permissible limits.
If sufficient amounts of U and other radionuclides accumulate, they can be hazardous to plants, animals, and humans (20). Uranium accumulation has an impact on plant germination, early seedling growth, and photosynthetic, metabolic, and physiological activities (21). Given that As, Pb, Tl, and U were found in high concentrations in sample SN10-FL, it can be considered that their origin was mainly influenced by the anthropogenic factor, usually by mining activities in this region where the mine of Hajvali is located, heavy road traffic, waste dumps, industrial areas, etc. (15).
Statistical analysis
Considering the significant difference in the contents of elements in leaf and flower samples presented in the results above, it was logical to assume a class-based PCA approach, where the data from the flower and leaf samples would be treated in separate PCA models. The initial PCA model of the leaf samples was built using four main components that explained 69.1 % of the data variability (R2X = 0.691). The loading plot of the model (Fig. 1) revealed the prominent correlation patterns in the dataset. Thus, when comparing p1 and p2, one can observe how the X-variables are interrelated and correlated. The plot illustrates the variations among the X-variables, indicating which ones share similar information, and which ones exhibit negative correlations or lack correlation, and also identifies those that are poorly explained by the model (p1 and p2 being close to 0). Tl, Cu, and V could be considered as elements (X-variables) that had the lowest variance in the dataset and/or were not related to the variations in other elements, while Fe, Al, K, Li, As, B, Bi, Ca, Mn, Mg, In and Hg, presented highest variability in the dataset and could be considered as important for the model calculations. Further on, using HCA, an attempt was made to classify the samples according to their scores in the main components and try to examine the similarities and patterns in the specific contents of elements in different leaf samples. The dendrogram revealed three distinctive groups of samples (group 1 – SN-11, group 2 – SN-6 and SN-10, and group 3 – SN-1, SN-2, SN-3, SN-4, SN-5, SN-7, SN-8 and SN-9), which were the basis of the PLS-DA model. The PLS-DA model was built using three main components (R2X = 0.551, R2Y = 0.897) and its VIP plot (Fig. 2a) highlighted the variables Fe, Al, B, Zn, Ca, Hg, Mn, As, U, Na, Mg, and In as elements whose content presented the main source of variation among the examined group of samples. As SN-11 was already reported as an outlier due to the extreme quantitative differences (outside of the 3SD range from the average) in the content of Cd, Co, Hg, Na, and In, the focus was set on the samples from group 2 which exhibited a distinct pattern of heavy and essential element contents compared to the rest of the dataset (group 3) (Fig. 2b), as indicated by the VIP plot mentioned above.
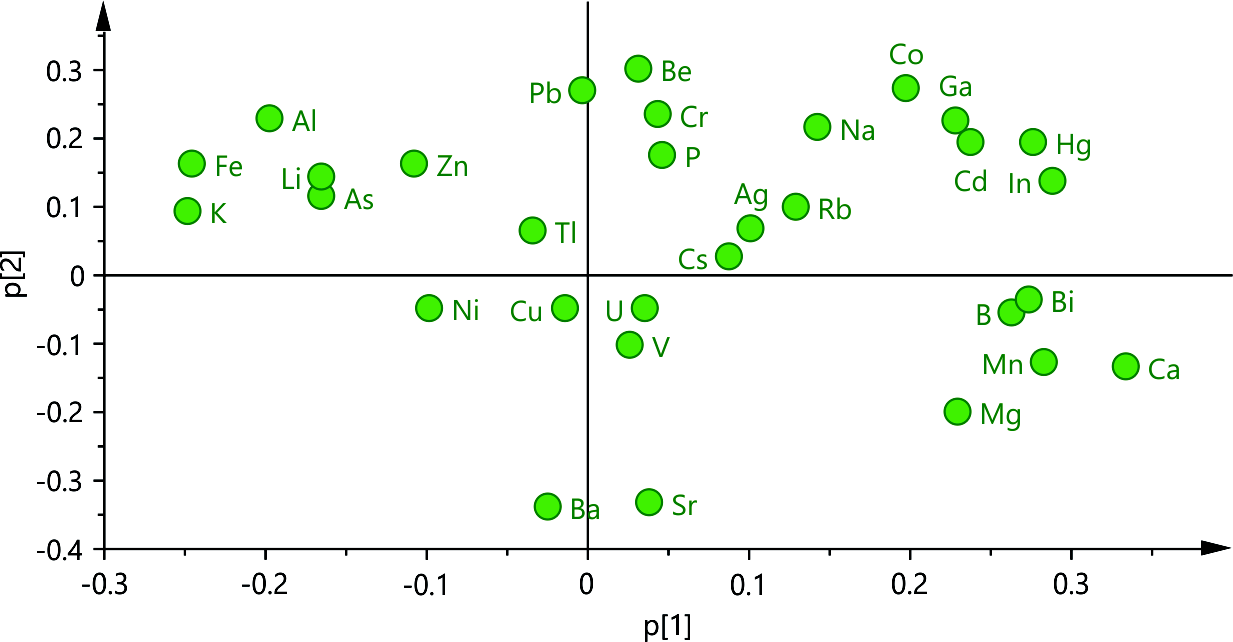
Fig. 1. Loading plot p1 vs. p2 from the PCA model of the leaf samples element content data.
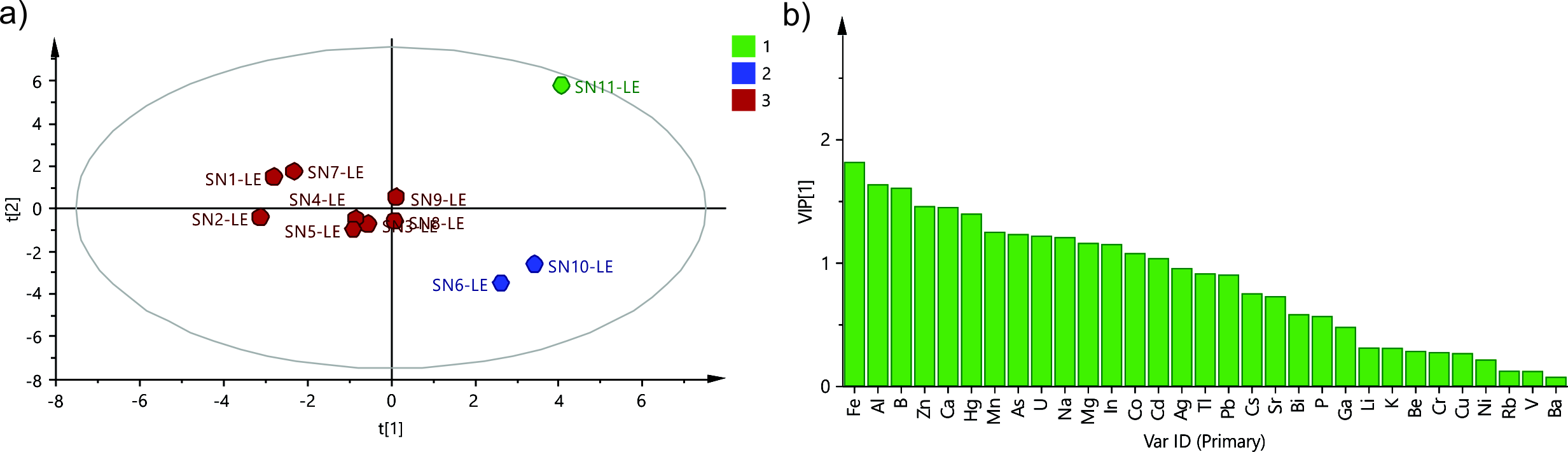
Fig. 2. PLS-DA model for the element content of the S. nigra leaf samples: a) score scatter plot t1 vs. t2, each sample is colored according to the class assigned by the model, b) VIP plot.
The PCA model for the flower samples was created using three main components, explaining 71.6 % of the variability in element content within the dataset. The loading plot (Fig. 3) showcases unique patterns and correlations among variables when compared to the leaf samples. Notably, the model cannot fully explain the variations in Rb content.
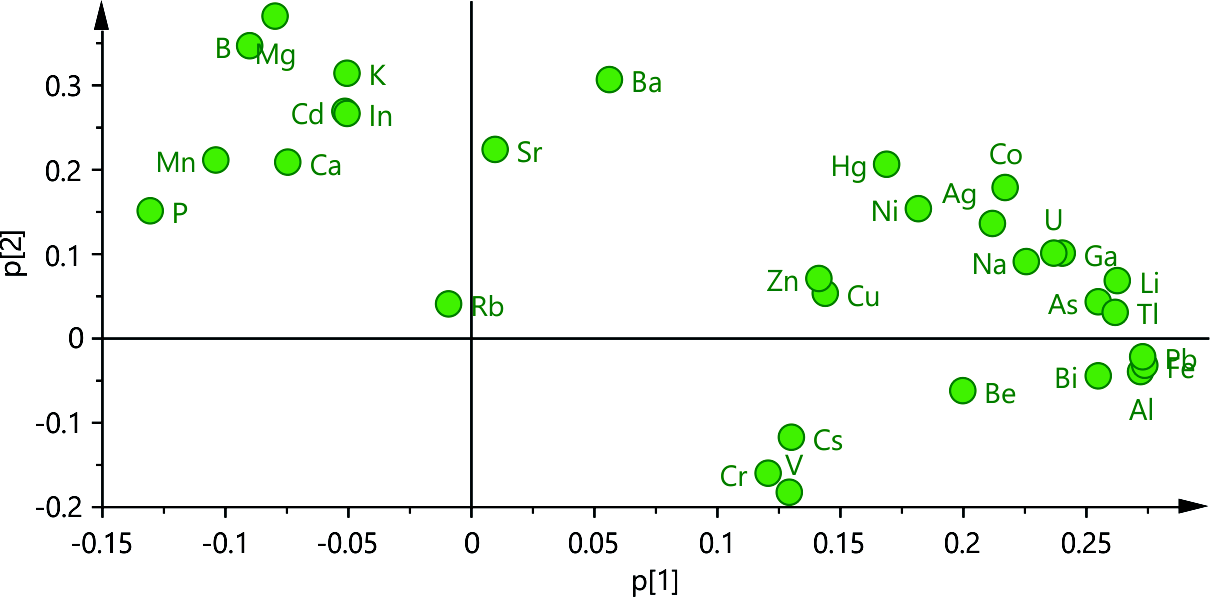
Fig. 3. Loading plot p1 vs. p2 from the PCA model of the flower samples element content data.
Additionally, the dendrogram successfully divided the samples into five distinct groups based on their scores in the main components (group 1 – SN-10, group 2 – SN-7 and SN-11, group 3 – SN-2, group 4 – SN-3, SN-6 and SN-9, and group 5 – SN-1, SN-4, SN-5 and SN-8). The PLS-DA model was further constructed on 4 main components (R2X = 0.819, R2Y = 0.827), and as presented in the score scatter plot (Fig. 4) both the first and second components were employed for successful grouping of the samples. According to the VIP plot the contents of Al, Fe, Pb, As, Tl, Bi, U, Li, Na, Co, Ga, Ag, as well as Sr, Mg, Ba, B, and K for the first and second component, resp., are considered important for the implementation of the discriminative variable selection.
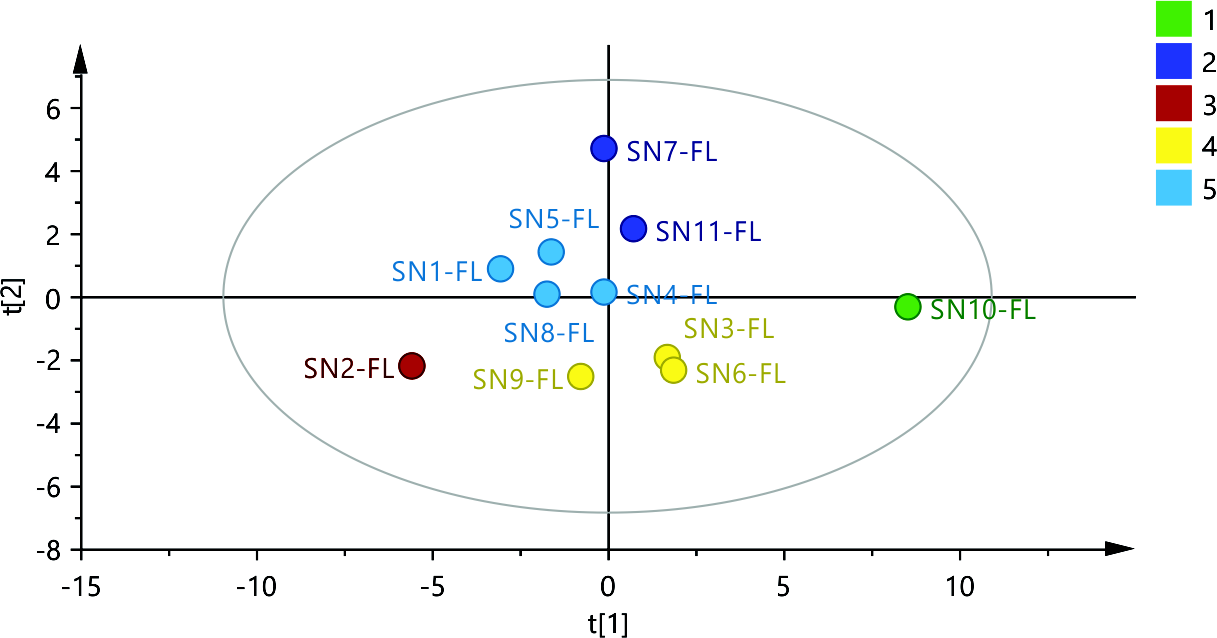
Fig. 4. Score scatter plot t1 vs. t2 from the PLS-DA model for the element content of the flower samples, each sample is colored according to the class assigned by the model.
CONCLUSIONS
Variations in mineral concentration were observed between leaves and flowers of S. nigra concerning geographic locations. The multivariate analysis revealed distinctive patterns of element contents in leaf and flower samples, highlighting the importance of Fe, Al, B, Zn, Ca, Hg, Mn, As, U, Na, Mg and In for the leaf samples, and Fe, Pb, As, Tl, Bi, U, Li, Na, Co, Ga, Ag, Sr, Mg, Ba, B, and K for discriminative selection of the samples. The content of heavy metals and toxic elements was higher in leaves than in flowers but sill within the limits recommended by Ph. Eur.