Introduction
Today, artificial sweeteners are produced and consumed for a wide variety of purposes (Li et al. 2021a) in the food, cosmetic, and pharmaceutical industries (Stolte et al. 2013). These sweeteners are substitutes for sugar in terms of flavor and serve as alternative food additives since they provide the desired taste in very small amounts and without calories (Chattopadhyay et al. 2014). Artificial sweeteners have been frequently observed in the environment in recent years because of their high consumption, persistence in the environment, and solubility in water (Lange et al. 2012, Li et al. 2020a). Despite their widespread use, the potential risks of artificial sweeteners to the environment and human health are still unclear (Li et al. 2021b). They are classified as anthropogenic trace pollutants with high concentrations in groundwater, surface waters, and drinking water (Lange et al. 2012). The main source of artificial sweeteners released into surface waters is wastewater treatment plants. Artificial sweeteners like many other chemicals cannot be effectively removed by conventional wastewater treatment and are often part of wastewater discharges to surface water (Li et al. 2021b).
Plants, stable organisms in ever-changing environmental conditions, are inevitably exposed to various biotic and abiotic stress factors. These stress factors negatively affect both quality and crop yield. The underlying reason is oxidative damage caused by increased accumulation of reactive oxygen species (ROS) (Miller et al. 2010). Under optimal physiological conditions, reactive oxygen species such as hydrogen peroxide (H 2O 2), singlet oxygen ( 1O 2), superoxide (O 2 -), and hydroxyl radical (OH -) are frequently produced as by-products of aerobic metabolism. The increased accumulation of ROS in stressed plants is due to the disturbed balance between ROS production and their removal (Mittler et al. 2004). Increased levels of ROS produced under stress can cause the oxidation of lipids, proteins, and nucleic acids; these life-threatening reactions activate plant stress responses and defence pathways. The environmental impacts depend on the delicate balance between ROS generation and ROS scavenging, which can be influenced by the intensity of stress and stress duration (Sharma and Dietz 2009). In plants, prevention or clearance of stress-induced ROS accumulation occurs with the active roles of molecules such as enzymatic and non-enzymatic soluble sugars (Miller et al. 2010). Abiotic stress is estimated to be the main cause of excessive crop loss, which is 50% worldwide (Mittler 2002, Gill and Tuteja 2010). Therefore, it is important to understand the responses to stress of ROS signaling pathways and their interaction(s). Previous studies on the impacts of pharmaceutical and personal care products on plants (Osma et al. 2018, Turkoglu et al. 2019, Elveren and Osma 2022) led to the hypothesis that artificial sweeteners might also negatively impact plants and induce changes in plant stress markers such as antioxidant enzymes.
The artificial sweeteners evaluated in this study (saccharin, sodium cyclamate, sucralose, aspartame) are some of the most widely consumed commercial products. Sucralose is a calorie-free high-intensity artificial sweetener that is widely used in a variety of foods and beverages worldwild (Li et al. 2020b). Saccharin is approximately 300 times sweeter than sucralose and is especially found in carbonated beverages in Turkey (Bayındır Gümüş et al. 2022). Aspartame has low calorie content; hence it is a non-nutritive sweetener (More et al. 2021). Today with an annual production of 3000–5000 metric tons, aspartame is one the world’s most widely used artificial sweeteners. It is an ingredient in more than 5000 food and beverage products including cereals, chewing gum, pharmaceuticals, and instant coffee (Landrigan and Straif 2021). Sodium cyclamate is utilised as non-nutritive sweetener. Its equivalent calcium salt is used particularly in low sodium diets. Cyclamate is 30 times sweeter than sucrose. Owing to its heat-stable properties, it is suitable for cooking and baking (More et al. 2021).
These sweeteners can reach the soil (including agricultural areas) in a variety of ways: spreading fertilizer and sewage sludge and using treated wastewater for irrigation (water recycling). Subsequently, they enter the food chain through vegetation consumption or impact plant physiology in a negative manner. In this study, the effects of artificial sweeteners applied to soil at different concentrations were evaluated by monitoring electrolyte leakage, antioxidant enzyme activities, and chlorophyll and carotenoid content in bread wheat plants. Bread wheat ( Triticum aestivum L.) is one of the most important plants representing more than half of the calories consumed in human nutrition and approximately 50% of the protein (Aydogan and Yagdı 2021). It is grown throughout the world making it an ideal plant for evaluating the potential impact of anthropogenic pollutants that may be present in treated wastewater.
Materials and methods
Plant material and treatments
Seeds of the licensed wheat variety “Esperia” were supplied from the Agricultural Credit Cooperatives of Turkey. The artificial sweeteners employed, sucralose (C 12H 22O 11), saccharin (C 7H 5NO 3S), aspartame (C 14H 18N 2O 5) and sodium cyclamate (C 6H 11NHSO 3Na) were purchased from Sigma Company.
Different concentrations (0, 25, 50, 100 mg kg -1) of artificial sweeteners were mixed as powder into the soil mixed with 650 g of animal manure and then 7 g of wheat seeds were sown
on it and covered with 100 g of soil. There were 3 pots with seeds for each of sweetener/concentration combination and 250 mL of water was added. Wheat grown without the addition of artificial sweetener served as the control group. After the seeds germinated, the wheat seedlings were irrigated at regular intervals to maintain field capacity moisture. Plants were grown in a laboratory at 5 - 10 oC temperature and under 60% humidity. Following germination, the ambient temperature was set at 10 - 15 oC. At the end of the 15 th day, the wheat leaves were harvested and some of them were used fresh to determine the amount of electrolyte leakage, while others were frozen for subsequent physiological and biochemical studies that served as markers of plant stress. For each parameter 10 replicates were prepared.
Determination of electrolyte leakage in leaf samples
The determination of electrolyte leakage was conducted on 0.1 g of fresh leaves in test tubes. 4 mL of distilled water was added to each test tube and incubated at 4 °C for 24 h. The amount of ions present in water was determined using an electrical conductivity meter (Elveren and Osma 2022).
Determination of antioxidant enzyme activity
Leaves (0.5 g) were weighed and placed in a porcelain mortar. 5 mL of cold homogenate buffer (0.1 M KH 2PO 4, pH 7.0 containing 1% polyvinylpyrrolidone (PVP) and 1 mM ethylenediaminetetraacetic acid (EDTA)) were added, and the mixture was transferred to a centrifuge tube; the mixture was centrifuged (15000 x g, 4 °C) for 15 minutes. The supernatant (“enzyme extract”) was used as a source for the activity measurements of antioxidant enzymes as described below. The chemicals and procedures used to measure the activities of each antioxidant enzyme followed those of Turkoglu et al. (2019).
Peroxidase (POX) activity determination was based on monitoring the absorbance increase at 470 nm caused by a colored product of the reaction in which guaiacol and H 2O 2 are substrates. 10 µL of enzyme extract was added to a spectrophotometer cuvette containing 3 mL of the substrate solution (100 mL of 0.1 M NaH 2PO 4, pH 5.5 with the addition of 5 mM guaiacol). The increase in absorbance was recorded at 1-minute intervals for 5 minutes. The amount of enzyme that increased the absorbance by 0.01 units in 1 minute at 25 °C was accepted as 1 enzyme unit, and the results were presented as enzyme unit per g of fresh tissue (EU g -1 FW) (Osma et al. 2018).
The method developed by Havir and McHale (1987) was used for the determination of catalase (CAT) activity. This method is based on monitoring the absorbance decrease at 240 nm following the addition of the plant extract to phosphate buffer containing H 2O 2. Absorbance against the blank was read at 1-minute intervals for 3 minutes. The decrease in absorbance per minute was calculated from the interval in which the absorbance decreased linearly. The amount of enzyme that reduced the absorbance of 1 mole H 2O 2 in 1 minute at 25 °C was accepted as 1 enzyme unit, and the results were presented as enzyme unit per g of fresh tissue (EU g -1 FW) (Osma et al. 2018).
Superoxide dismutase (SOD) activity was based on the spectrophotometric determination of the inhibition of the photochemical reduction of nitro blue tetrazolium (NBT). The reaction mixture (3 mL) contained 50 mM KH 2PO 4, pH 7.8 with the addition of 13 mM methionine, 75 M NBT, 2 M riboflavin, and 0.1 mM EDTA. For the activity measurement, 2.84 mL of the reaction mixture without riboflavin was added to a 3-mL spectrophotometer cuvette, then 100 μL of enzyme extract was added. The reaction was initiated by pipetting 60 μL of a 100 M riboflavin solution into the tube and placing it in front of a white light source immediately after mixing. The tube is kept in front of the light source for 15 minutes; the reaction was stopped by turning off the light source. The absorbance of NBT was read against the blank at 560 nm. The blank consisted of an enzyme-free sample from the same process as above. The amount of enzyme causing 50% inhibition of NBT reduction was accepted as 1 enzyme unit (EU), and the values were presented as EU g -1 FW (Turkoglu et al. 2019).
Determination of photosynthetic pigments
Leaves (0.5 g) were homogenized in 5 mL of 80% acetone. The homogenate was filtered using filter paper and then brought to 10 mL volume with acetone. The homogenate was centrifuged for 10 minutes, the supernatant was collected, and absorbance at 663, 646, and 440 nm was recorded. The following formulas were used to calculate chlorophyll and carotenoid (Porra et al. 1989):
Chlorophyll a (mg mL -1) = 12.25 × A 663 - 2.55 × A 646
Chlorophyll b (mg mL -1) = 20.31 × A 646 - 4.91 × A 663
Chlorophyll a + chlorophyll b (mg mL -1) = 17.76 × A 646 + 7.34 × A 663
Carotenoids (mg mL -1) = 4.69 × A 440 - 0.267 × (chlorophyll a + chlorophyll b)
Statistical analysis
Analysis of variance (ANOVA) in SPSS was used to determine the significance of treatment effects relative to control (untreated) plants for each individual artificial sweetener. Post hoc tests (Tukey-Kramer multiple comparisons) were performed when significant treatment effects were observed (P ≤ 0.05).
Results
In this study, four artificial sweeteners (at different concentrations) were applied to soil in which wheat seeds were germinated and grown. The presence of these artificial sweeteners was found to adversely impact the plant stress markers we evaluated in comparison to wheat plants grown in the absence of these artificial sweeteners. In general, the plant biomarkers did not follow a typical monotonic dose-response pattern i.e. incremental increases in concentration did not always produce only incremental increases or only incremental decreases in plant stress response.
Electrolyte leakage
Electrolyte leakage in plants significantly increased with increasing soil concentrations of saccharin and aspartame; the effect was especially true for plants exposed to saccharin (Fig. 1). This indicates that the presence of saccharin or aspartame in soil produces a general stress response by compromising cell membranes which could negatively impact plant growth.
Sucralose and sodium cyclamate at soil concentrations as high as 100 mg kg -1 had no impact on electrolyte leakage compared to control (untreated) plants, suggesting that these sweeteners did not compromise plant cell membranes during the 15 days study.
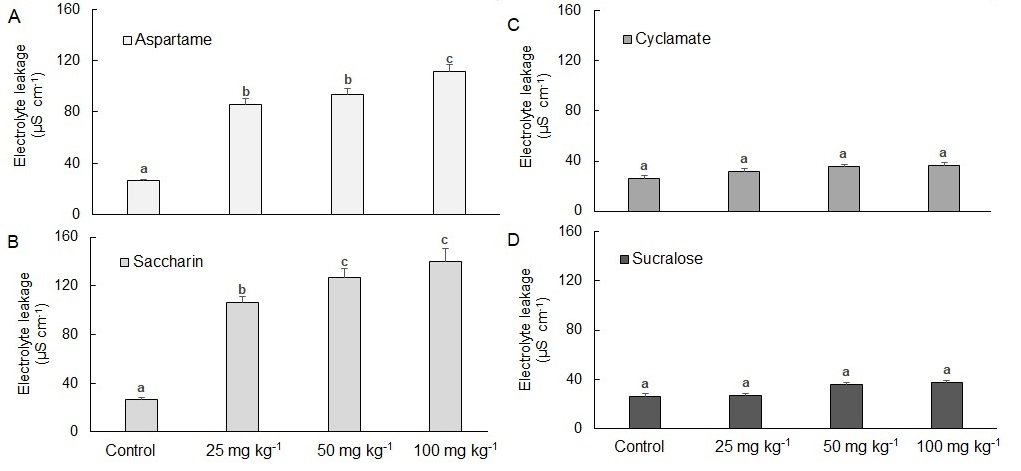
Fig. 1. Electrolyte leakage in wheat (Ttriticum aestivum L.) exposed to different concentrations of artificial sweeteners in soil for 15 days. A − sucralose, B − saccharin, C − cyclamate, D − aspartame. Results represent mean ± standard error, n =10. Bars with different letters are statistically different within a sweetener treatment.
Photosynthetic pigments
Significant changes in plant pigments in wheat following exposure to all artificial sweeteners were observed (Tab. 1).
The most dramatic changes in plant pigments occurred in the aspartame treatment (Tab. 1). Depending on the sweetener type and the pigment, changes mostly followed a monotonic pattern. In other words, as artificial sweetener concentration in soil increased, plant pigment concentrations increased compared to control plants. Chlorophyll a concentrations mostly increased after treatments with all artificial sweeteners.
For aspartame in soil, concentrations of chlorophyll a, chlorophyll b, and carotenoids increased already at the low soil concentration, but did not further increased at higher soil concentrations (Tab. 1).
For saccharin, the increase in concentrations of chlorophyll a and chlorophyll b was sighnificantly higher at lower than at higher concentrations (non-monotonic increase) (Tab. 1).
For sucralose, a non-monotonic increase of chlorophyll a content and a monotonic increase of carotenoids was noticed, while chlorophyll b content showed a monotonic decrease (Tab. 1).
For cyclamate, chlorophyll a content showed a monotonic increase, chlorophyll b a non-monotonic increase while carotenoids initially increased at the lowest concentration followed by no change at the higher concentrations (Tab. 1).
Tab. 1. Chlorophyll a, chlorophyll b, chlorophyll a+b, and carotenoids (mean ± standard error, n = 10) in wheat grown in soil containing artificial sweeteners for 15 days. For values with a different superscript letter within a treatment, the difference between the treatment means and control was statistically significant (ANOVA, P ≤ 0.05).
Antioxidant enzymes
Three of the four artificial sweeteners significantly increased CAT activity in wheat plants compared to control (untreated) plant. It was mostly a monotonic increase, although for aspartame the increase was significant already at the lowest concentration while for sucrose only at the highest concentration applied.
All four artificial sweeteners tested significantly increased POX activity in wheat plants compared to control (untreated) plants (Tab. 2). For aspartame a monotonic increase but for saccharine and cyclamate a non-monotonic increase in POX activity was observed. For sucralose, the increase in POX activity was observed only at the lowest (25 mg kg -1) and the highest (100 mg kg -1) concentrations.
Three of the four artificial sweeteners significantly changed SOD activity in wheat plants relative to control plants (Tab. 2). For cyclamate, a monotonic increase in SOD activity with increasing concentration of sweeteners was noticed. For aspartame, a significant increase was observed only at 50 mg kg -1 concentration while for sucralose only the highest concentration caused an increase. For saccharin, a decrease in SOD activity was observed at the lowest (25 mg kg -1) concentration; while 50 mg kg -1 and 100 mg kg -1 concentrations did not significantly affect SOD activity compared to control plants.
Tab. 2. Antioxidant enzyme (catalase - CAT, peroxidase - POX, superoxide dismutase - SOD) activity (mean ± standard error, n =10) in wheat grown in soil containing artificial sweeteners for 15 days. For values with a different superscript letter within a treatment, the difference between the treatment means and control was statistically significant (ANOVA, P ≤ 0.05).
Discussion
Only a few studies on the effects of artificial sweeteners on plants have been reported. Kobetičová et al. (2016) evaluated the effect of two artificial sweeteners (aspartame and saccharin) on aquatic (duckweed, Lemna minor; algae, Desmodesmus subspicatus) and terrestrial (white mustard, Sinapis alba; lettuce, Lactuca sativa) plants. Aspartame had a negative impact on duckweed growth. Unlike aspartame, saccharin had no negative effects on duckweed and the production of chlorophyll increased. It was suggested that it could be a stress response as enhanced photosynthesis could be related to the maintenance of the metabolism under stress (Kobetičová et al. 2016). In this study, both sweeteners (aspartame and saccharin) increased chlorophyll content in plants relative to controls, although saccharin treatment increased chlorophyll a and chlorophyll b content more dramatically at the lowest soil concentrations which could be consistent with plant response to stress.
Kobetičová et al. (2018) also evaluated potential growth effects of four artificial sweeteners (aspartame, saccharin, sucralose, and acesulfame K) and a natural sweetener (stevioside) on duckweed ( Lemna minor). They determined that aspartame and sucralose inhibited growth parameters (frond number and frond area), but did not affect chlorophyll concentrations. In this study, aspartame as well as saccharin increased the photosynthetic pigments in all concentrations investigated while sucralose increased chlorophyll a and carotenoids but decreased chlorophyll b at the highest soil concentration. Amy-Sagers et al. (2017) examined the effect of sucralose on Lemna minor and determined that the plant used sucralose instead of sugar to increase photosynthetic capacity and green leaf area. They determined that duckweed utilizes sucralose in metabolism as a carbon source.
Electrolyte leakage in plants significantly increased with increasing concentrations of saccharin and aspartame in the soil, indicating that these two sweeteners are more toxic to wheat than sucralose and cyclamate, causing oxidative damage of membranes. This could be also related to the observed increase in photosynthetic pigments especially carotenoids which could serve as antioxidants in an effort to protect the plant from stress (Mittler 2002, Gill and Tuteja 2010). It could be hypothesized that aspartame and saccharin may cause ion escape by increasing the permeability of the cell membrane more than other sweeteners. However, observed electrolyte leakage was not consistently related to antioxidant enzyme activities. Saccharin and aspartame caused increases in CAT activity and POX activity, probably as a result of increased H 2O 2 as the role of these enzymes is to scavenge H 2O 2. However, the activity of SOD, enzyme that catalyzes the dismutation of superoxide anion to H 2O 2 was not affected in a consistent manner. Sucralose and cyclamate at soil concentrations as high as 100 mg kg -1 had no impact on electrolyte leakage. However, sucralose increased CAT activity, while cyclamate caused an increase in SOD and POX activity indicating that they can induce some change in plant metabolism which is reflected in the activity of antioxidative enzymes.
Chlorophyll a content and POX activity proved to be sensitive parameters that could be used as stress markers in wheat. It is consistent with studies investigating the effect of active ingredients of drugs and personal care products on wheat (Turkoglu et al. 2019, Elveren and Osma 2022). SOD activity was the least sensitive plant stress marker in this study. It is possible that some sweeteners can react with superoxide radicals, causing a decrease in the amount of superoxide in the environment and a subsequent decrease in SOD activity.
Generally, the plant biomarkers did not follow a typical monotonic dose-response pattern. This pattern is consistent with previous observations on the impacts of pharmaceuticals and perosonal care products to wheat plants (Osma et al. 2018, Turkoglu et al. 2019, Elveren and Osma 2022).
Conclusions
The artificial sweeteners present in the soil could exert significant changes in plant metabolism. All four artificial sweeteners (saccharin, sodium cyclamate, sucralose, aspartame) inreased the concentration of chlorophyll a and POX activity, altough not always in a consistent monotonic manner. Saccharin and aspartame in the soil were more toxic to wheat plants inducing oxidative damage to membrane and activating antioxidative enzymes CAT and POX, thus suggesting that oxidative stress might be a mechanism which explains their toxicity.
Individual photosynthetic pigments or oxidative stress markers can be useful for evaluating the impact of different chemicals in the environment on plants provided they have adequate sensitivity to the stress. However, it is always more appropriate to use several markers, as different chemicals can impact plants differently.
The ecotoxicological effects of artificial sweeteners remain to be determined; the possible physiological, biochemical, and genetic changes that they may produce in plants should be further investigated.
Acknowledgments
This study was supported by the Erzincan Binali Yıldırım University of Turkey, Scientific Research Projects Coordination Unit (Project Number: FBA-2020-727).