Introduction
Yoghurt is a widely consumed dairy product that has gained popularity due to its specific taste, nutritional value, and health benefits. However, the moderate protein content of traditional yoghurts limits their ability to provide adequate protein supplementation for individuals who require higher protein intake, such as athletes, elderly people, and those recovering from injuries or illnesses. To address this limitation, researchers have explored various methods for processing high-protein yoghurts. The market for organic and functional foods is rapidly growing, with a projected global size of $275.77 billion by 2025, driven by increasing consumer awareness of the link between diet and health (Tomić Maksan et al. 2021; Grand View Research, 2019).
Among the common methods of producing high-protein yoghurt is the concentration of yoghurt after fermentation (Ozer et al., 1998; Ozer et al., 1999) or prior to fermentation by methods such as ultrafiltration (Rattray and Jelen, 1996; Gésan-Guiziou et al., 1999) or microfiltration of milk (Chen et al., 2018). Another often-used approach for high-protein production is the direct fortification of yoghurt with various protein concentrates and isolates before the fermentation step. Protein sources can be of both animal and plant origin. While animal protein sources are mainly limited to the use of whey protein powders, the situation in the realm of plant proteins is significantly more diverse. In addition to conventionally employed soy and wheat proteins, proteins derived from various crops, including pea, fava bean, mung bean, rice, oat, chickpea, chia seed and potato, are also utilized (de Paiva Gouvea et al., 2010).
A particularly promising plant protein candidate for integration into dairy products is wheat bran protein isolate (WBPI). Wheat bran is a by-product of wheat milling and is a rich source of protein, dietary fiber, and antioxidants (Elleuch et al., 2011). A notable advantage of WBPI lies in its procurement from the readily available wheat bran, the outer layer of the wheat grain, which is an economically viable and environmentally sustainable by-product abundantly generated during the milling process. The protein isolation process involves sequential procedural stages, including defatting, solubilisation, and precipitation, with derivation from the peripheries of the wheat grain (Pořízka at al., 2023). The resulting protein isolate is characterized by a high protein content (up to 90 %), with essential amino acids in quantities that meet or exceed the recommended daily intake (Uttam et al., 2023). WBPI is notably rich in asparagine, glutamic acid, leucine, arginine, and proline. Its Protein Digestibility Corrected Amino Acid Score (PDCAAS) closely approximates that of pea and soy protein isolates. In addition to its protein related constituents, WBPI incorporates bioactive elements, including polyphenols, phytosterols, and lignans. These constituents have been empirically associated to health benefits, including the improvement of cardiovascular health, antioxidative efficacy, and potential anticancer properties (Pořízka et al., 2023; Alzuwaid et al., 2020; Uttam et al., 2023; Nongonierma and Fitzgerald, 2017; Arte et al., 2019; Jørgensen et al., 2019).
The potential application of WBPI in yoghurt extends beyond its nutritional benefits. The use of plant-based proteins, such as WBPI, can contribute to sustainable food production practices by reducing reliance on animal-based protein sources. Additionally, fortifying yoghurt with WBPI can offer a new product for consumers seeking alternative protein sources and expand the yoghurt product market. However, high-protein products, in general, exhibit fundamentally different sensory properties compared to standard yoghurt. Differences are particularly evident in texture, taste, and aroma. For example, the incorporation of whey protein isolate (WPI) in reduced-fat and non-fat yoghurts played a crucial role in replacing fat and improving the texturing properties of these yoghurts. The addition of WPI improved the structure and quality of the yoghurts, particularly in terms of texture and physical characteristics. Set-type yoghurts fortified with WPIs exhibited a softer texture and experienced less syneresis (liquid separation) compared to the control yoghurts (Hashim et al., 2021). Another study demonstrated that fortification with 5 % soy protein led to an increased consistency and stability of the yoghurts. On the other hand, fortification with 5 % whey protein resulted in the formation of aggregates due to excessive coagulation during fermentation. The moisture content and total solid content of the yoghurts were affected by the type as well as by the concentration of proteins used for fortification. The higher the protein fortification level, the lower the moisture content, and the higher the total solid content of the yoghurts. The addition of whey protein led to yoghurts with higher hardness, gumminess, and chewiness compared to the control (without protein fortification) and soy protein-fortified yoghurts. However, whey protein and soy protein did not significantly affect the springiness and cohesiveness of the yoghurts. In terms of rheological properties, soy protein-fortified yoghurts exhibited dynamic viscosity and apparent viscosity values similar to the control yoghurt. The shear stress-shear rate relationship, which determines the apparent viscosity of the yoghurts, could be accurately predicted using the power law model, with an accuracy ranging from 80 % to 99 %, depending on the level of protein fortification and protein type (Mitra et al., 2022).
The novelty of the present study lies in the use of a new type of protein isolate (WBPI) in the production of high-protein yoghurt. This application has not been studied at a scientific level yet. The objectives of this study were to determine the optimal levels of WBPI for yoghurt fortification and to evaluate the impact of its inclusion on sensory and nutritional properties. Additionally, the study investigated the effect on the rheological properties and viability of starter culture bacteria.
Materials and methods
Isolation and characterization of protein isolate from wheat bran
Protein isolate from wheat bran was obtained using the pH-shift method with modifications. Wheat bran was milled (<0.2 mm). The protein fraction was extracted from wheat bran using distilled water with a pH exceeding 10.5 (1 M NaOH) in a ratio of 1:20 (w/v) with continuous mixing for 2 hours. The pH of the suspension was monitored every 30 minutes. After 2 hours, the suspension was centrifuged (8000 rcf, 10 minutes), and the supernatant was collected. The pH of the supernatant was adjusted to 4 (1 M citric acid), mixed thoroughly, and cooled to 4 °C to facilitate protein precipitation from the aqueous phase. After another centrifugation, the sediment was collected and lyophilized. The protein content of the isolated WBPI was determined using the Euroconvertor EA 3100 elemental analyser, where the N content was multiplied by the protein conversion factor 6.31 for wheat bran.
Manufacturing of high protein yoghurt
Fresh, whole-fat milk (3.6 %), pasteurized (Moravia Lacto, Czech Republic), was used for manufacturing all yoghurt samples. A freeze-dried starter culture (YF-L812, Chr. Hansen, Denmark) containing Streptococcus thermophilus and Lactobacillus delbrueckii subsp. bulgaricus was used to create a 1 % w/w inoculum. For the reference sample, the milk was first heated to 70 °C for 10 minutes. After cooling to room temperature, the inoculum was added to the milk in a ratio of 1:100. The mixture was thoroughly mixed and placed in a yoghurt maker (43 °C) for 8 hours. Finally, the reference sample was refrigerated and cooled to 4 °C for further analysis.
To manufacture high-protein yoghurt, WBPI was added to the milk after heating, prior to fermentation. The protein content of the products was increased by adding 2 % and 5 % of WBPI. The suspensions were homogenized before and after inoculation. The conditions for producing high-protein yoghurts were the same as for the reference. All samples were analysed in duplicates. Before every analysis, the samples were mixed and homogenized again.
Dry matter and water holding capacity
To assess the impact of the addition of the protein concentrate on the physical properties of fermented milk products, the dry matter (DM) and water holding capacity (WHC) of individual samples were determined in duplicate. DM was measured by drying the samples in an aluminium dish in a dryer (105 °C) until a constant weight was achieved (approximately 3.5 hours). For WHC determination, approximately 10 mL of each sample was weighed in a 50-mL tube and centrifuged (10,000 rpm, 10 minutes, 25 °C). The supernatants were removed, and the sediment of the individual samples was then weighed. WHC was expressed as grams of sediment per 100 grams of the sample.
Rheological characteristics
The flow properties of the prepared samples were determined using a rotational rheometer (DHR-2, TA Instruments, Inc.). A flat steel plate-plate geometry with a 40 mm diameter was employed as a suitable sensor for these types of samples. Experiments were conducted at least in duplicates under a controlled temperature of 10 °C (maintained throughout the entire experiment using a Peltier plate system).
Before each individual measurement, a conditioning step was included (180 s; 10 °C). During this step, the samples were relaxed and tempered. The axial force during the squeezing of the sample into the geometry gap (1,000 µm) did not exceed 5 N. The steady-state flow step was applied to each individual sample in logarithmic scale mode. The shear rate was increased logarithmically from 0.1 s⁻¹ to 10 s⁻¹ with 6 measuring points per decade. After reaching the final shear rate (10 s⁻¹), the apparent viscosity was measured during the application of shear rates in descending mode (10 - 0.1 s⁻¹; 6 points per decade).
Analysis of cell number and cells viability by flow cytometry
The determination of the cell number and viability of bacteria was performed using flow cytometry with the fluorescent viability probe propidium iodide (Thermo Fisher Scientific). Initially, 1 gram of both reference and fortified yoghurts (8 days old) was resuspended in 10 mL of citrate buffer (100 mM, pH 4.4) and diluted with the buffer to a cell density of approximately 106 cells per mL. Subsequently, 2 μL of propidium iodide (2 mg/mL) was added to the suspensions (resulting in a final concentration of the stain of 4 mg/mL in 1 mL of the sample). The bacterial suspensions were then incubated in the dark at room temperature for 5 minutes. After incubation, the stained samples were immediately measured at the single-cell level using the Cytek Aurora flow cytometer (Cytek Biosciences Inc.) in triplicates, and the signal was observed in collecting channel B6 (617±35 nm).
Sensory analysis of yoghurt
The quality of the final fermented milk products was assessed through sensory analysis after 7 days of storage. Sensory descriptors such as texture, appearance, and flavour were evaluated by a panel consisting of 20 participants from the Faculty of Chemistry, all with relevant experience and knowledge of dairy products. The panel compared individual samples, including the reference, and assigned scores ranging from 1 to 5. The scores were given based on yoghurt descriptors, including homogeneity, viscosity, flavour, lumpiness, sweetness, sourness, bitterness, and overall impression. The median of each descriptor for individual samples was used for evaluation by Statistica (Tibco, USA).
Results and discussion
Properties of WBPI
The protein isolate used to produce protein yoghurts was obtained from wheat bran using the pH shift method as described in the experimental section. The composition of WBPI is presented in Table 1 and compared to soy and whey protein isolates.
Table 1. Composition of WBPI (Tamaru et al. 2014; Mohsen et al., 2009; Onwulata et al., 2004)
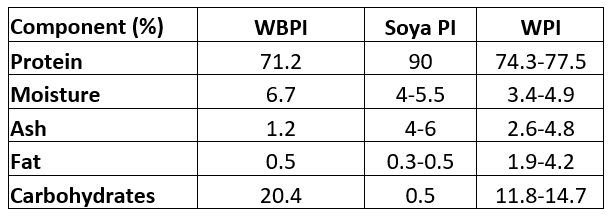
The composition of WBPI is comparable in many parameters to standard whey and soy protein isolates, typically ranging between 70-90 % protein content. WBPI contains 71.2 % proteins, primarily glutelins, albumins, prolamins, and globulins (Idris et al., 2003). The percentage composition of individual protein fractions depends on the degree of wheat bran grinding (Balandrán-Quintana et al., 2015).
Carbohydrates constitute the second most abundant component of WBPI. In plant protein isolates, they mainly consist of nutritionally significant dietary fiber, while in animal protein isolates, mono- and oligosaccharides dominate. The amount of carbohydrates in protein isolates is influenced by the isolation process or the structure of the protein source. Conversely, plant protein isolates typically have a very low fat content compared to animal proteins, making them nutritionally suitable for various types of diets.
A crucial nutritional indicator for protein isolates is their amino acid profile (AAP). The AAP of WBPI is presented in Table 2. WBPI contains nutritionally essential amino acids (EAA) as well as nonessential amino acids (NEAA). While EAA are generally considered more desirable, NEAA also provide several health benefits.
WBPI contains all essential amino acids, with their concentrations comparable to standard soy isolates. The total amount of EAA in WBPI is slightly lower than in whey protein isolate, as EAA are naturally more abundant in animal proteins. Nevertheless, this doesn't imply that WBPI is a poor source of EAA. Methionine is the limiting amino acid in WBPI, but a single dose of WBPI (typically 30 g of protein isolate) covers more than 40 % of the recommended daily intake of methionine.
The most abundant amino acid in WBPI is glutamic acid, and it also contains a relatively high amount of proline and semi-essential amino acids arginine and histidine (SEAA). Considering the AAP, WBPI could be considered for use in sports nutrition. Proline, along with hydroxyproline, is crucial for body protein synthesis, being the second most abundant amino acid in collagen and playing an important role in wound healing (Wu et al., 2011). Arginine supplementation is significant for sport performance, as it is related to NO synthesis, leading to increased blood flow, improved muscle contraction, and enhanced oxygen kinetics (Viri et al., 2020). The intake of histidine, up to about 8 g per day, could also provide several health benefits, including the reduction of inflammation in obese individuals, support for physical concentration, and a decrease in fatigue (Moro et al., 2020).
Table 2. Amino acid profiles of different protein isolates [g/100 g] (Mohsen et al., 2009; Tamaru et al., 2014; Banaszek et al., 2019; Chungchunlam et al., 2016).
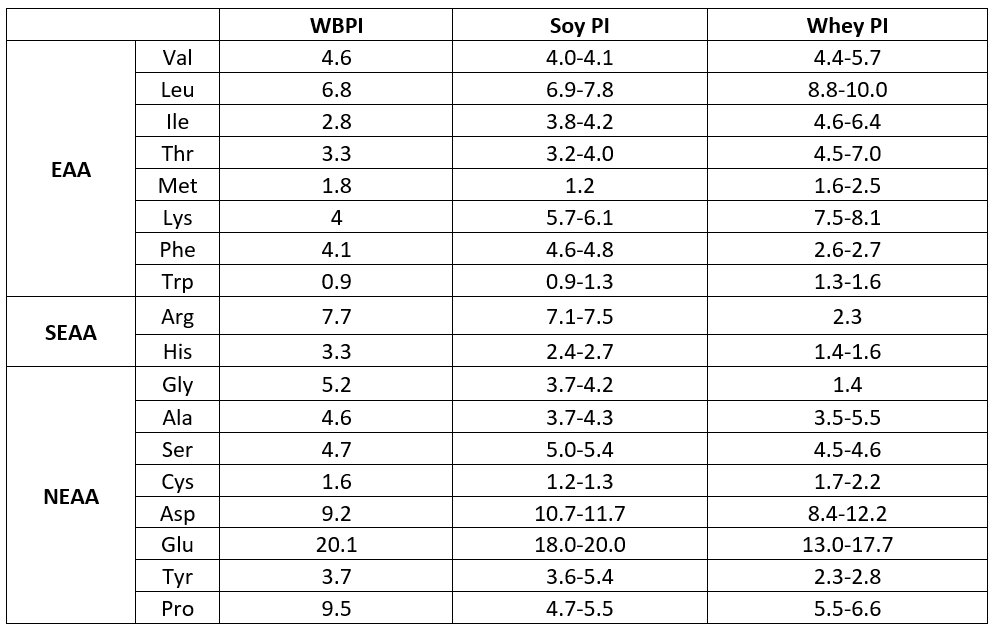
Influence of yoghurt fortification by WBPI on functional properties
Reference and fortified yoghurts were prepared following the procedures described in the experimental section. The fortification aimed to increase the protein content through direct addition of WBPI into the milk prior to fermentation. The milk used contained 3.2 % proteins, primarily represented by caseins and serum proteins. The addition of WBPI to the milk altered the nutritional parameters of the products, as shown in Table 3.
Table 3. Nutritional parameters of yoghurt samples
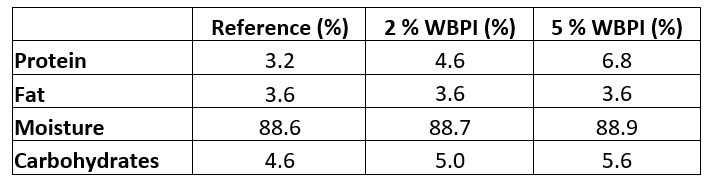
WBPI effectively increased the protein content of yoghurt. With the addition of 2 % and 5 % WBPI, the protein content of yoghurts increased to 4.6 % and 6.8 %, respectively. The increase in protein intake is essential not only for athletes but also for elderly people. While the protein Recommended Dietary Allowance (RDA) is 0.8 g/kg/day, experts recommend an intake of up to 2.0 g/kg/day, depending on an individual's physical condition (Wolfe, 2012; Baum et al., 2016; Volpi et al., 2013). This nutritional strategy helps older individuals avoid muscle loss, maintain strength, and can even reduce or prevent obesity (Volpi et al., 2013; Wolfe et al., 2008).
For the elderly, plant proteins may be preferred over animal proteins due to their lack of cholesterol (Qin et al., 2022) and their potential to reduce existing levels (Maki et al., 2010; Tong et al., 2021).
In addition to protein content, WBPI slightly enhanced yoghurts with dietary fiber, offering additional dietary benefits. Meanwhile, the fat content remained almost identical to that of the reference yoghurt.
Water holding capacity and dry matter
Protein-fortified yoghurts are significant not only due to their nutritional benefits but also because their improved consistency makes them more palatable, especially for the elderly. Texture is a crucial factor influencing consumers' perception of food. Texture defects can result from ingredients, the manufacturing process, or even during storage. While sensory analysis provides a basic description of texture quality, several instrumental methods can be employed for texture characterization.
In the case of yoghurts, one undesirable phenomenon is the syneresis of whey. Generally, higher dry matter content in yoghurts decreases the risk of syneresis. According to Tamine and Deeth (1979), it is recommended for commercial yoghurt production to use milk containing about 15 % dry matter. Dry matter content is often increased by adding milk or whey powder, leading to the production of thick and smooth yoghurts. However, the reduction of syneresis was not observed after adding maca powder to yoghurts, mainly composed of carbohydrates (Korkmaz et al., 2021).
Texture defects and changes in yoghurt are commonly evaluated by determining water holding capacity (WHC) or rheological properties of the products. WHC is quantified as the drainage of the sample under specific conditions, describing the structural homogeneity. The percentage of drained yoghurt after dynamic drainage (centrifugation) most often defines WHC, although it does not necessarily correspond to syneresis under normal storage conditions.
Figure 1 illustrates the percentage increase in WHC of yoghurt samples after the addition of WBPI. Enriching yoghurts with 2 % and 5 % WBPI increased dry matter to 14.5 % and 17.5 %, respectively, whereas the reference yoghurt contains 12.5 % dry matter. Fortification also caused an increase in WHC of the yoghurts by 1.6 % and 3.7 %, respectively, compared to the reference. The addition of WBPI to the yoghurts clearly shows a similar trend to the addition of milk or whey powder. Under the testing conditions, syneresis in both fortified samples was reduced.
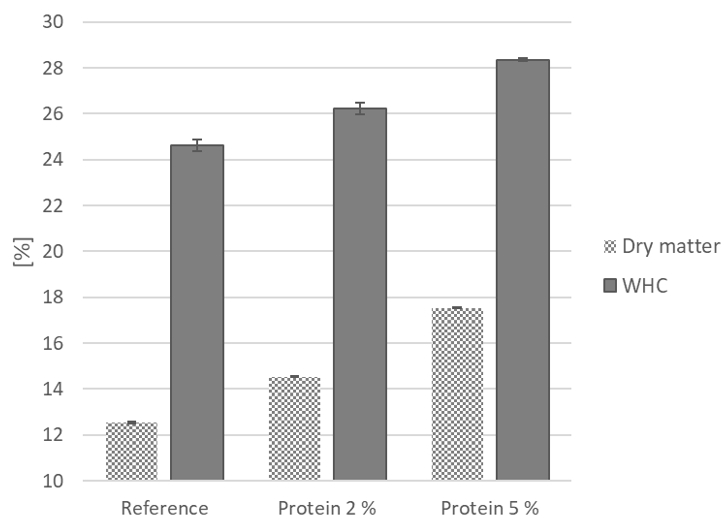
Figure 1. Dry matter (DM) and water holding capacity (WHC) of individual yoghurt samples.
Rheological properties of yoghurt samples
The flow properties of the reference yoghurt and yoghurts with the addition of 2 % and 5 % WBPI were measured as a function of shear rates using the methodology described above. All samples, regardless of the protein concentration, exhibited typical non-Newtonian behaviour, specifically pseudo plastic (shear-thinning) behaviour. In this behaviour, the apparent viscosity decreased with increasing shear rate, reaching a minimum viscosity at the highest shear rate.
Additionally, all samples demonstrated thixotropic behaviour, indicating that the apparent viscosity did not reach the zero-shear viscosity (8.8 Pa.s for the reference sample; see Figure 2) before deformation. After cycling deformation (an increasing and decreasing loop of shear rates), the apparent viscosity significantly decreased to 6.0 Pa.s, suggesting that the sample's recovery was no more than 70 %. This finding is beneficial as it indicates that the flowability of the measured samples can be significantly modulated through shear deformation.
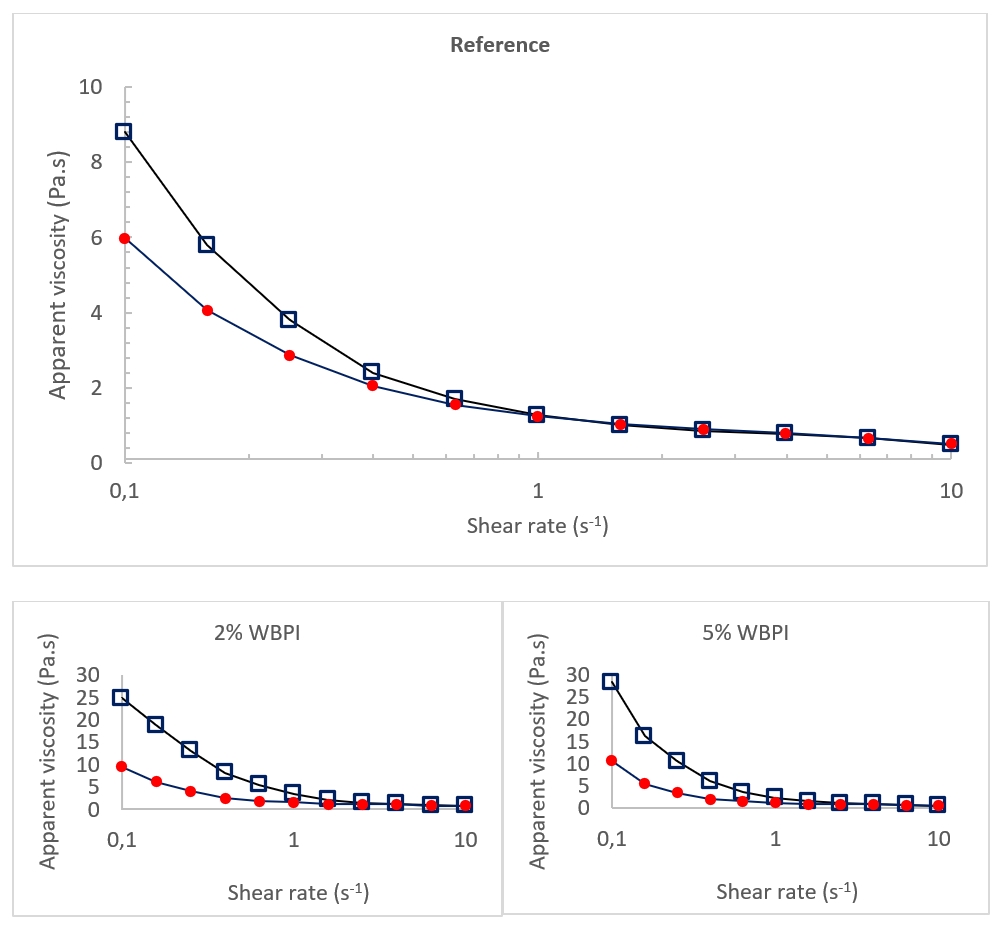
Figure 2. Apparent viscosity as a function of shear rate in forward (●); reverse (□).
One of the most crucial parameters connected with the sensory analysis related to the swallowability and processability could be found in yield stress (t0). The yield stress is the stress at which a material starts to flow. This crucial parameter is related to the structure of yoghurt. The yield stress was calculated through the rheological model Herschel-Bulkley.
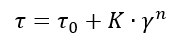
Where t is shear stress measured during the steady-state flow step, t0 is determined yield stress, K is consistency parameter and n is flow index. For all samples, the yield stress can be obtained by averaging the stress from the sweep between 0.1 and 1 s-1 giving the result of 0.94±0.07 Pa (the sample without addition of protein). The values of yield stress for the samples with the addition of protein are summarized in Table 4.
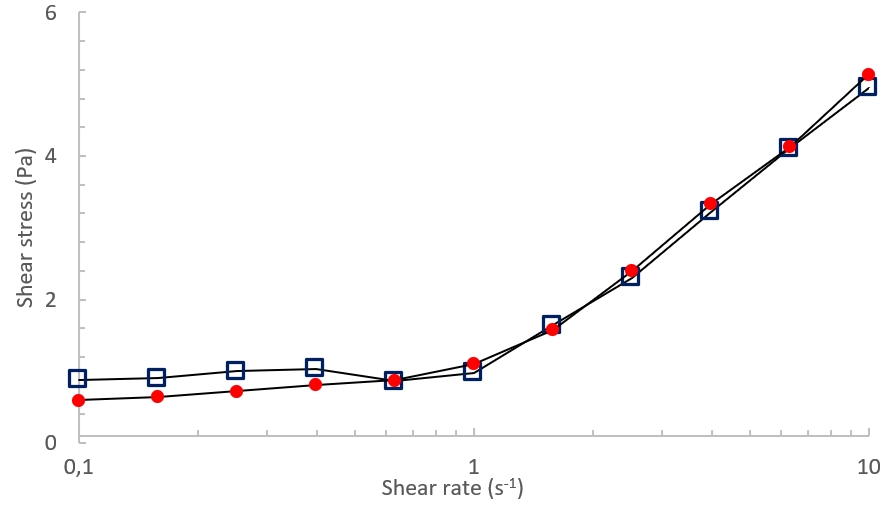
Figure 3. Shear stress as a function of shear rate in forward (●); reverse (□)
One of the primary objectives of the presented rheological data was to establish the relationship between the presence of protein in yoghurt composition and the flow properties of these samples concerning sensory analysis. Therefore, all protein-enriched samples were compared for the following parameters: 1) yield stress (as described above); 2) zero-shear viscosity (the apparent viscosity of the sample before shear application, specifically at the lowest shear rate); 3) infinite-shear viscosity (the apparent viscosity of the sample at the highest applied shear rate – 10 s-1); 4) sample recovery (the ratio of zero-shear viscosities after the application of the lowest shear rate and after the cycling loop deformation). These crucial parameters are summarized in Table 4.
Table 4. Rheological parameters of yoghurt samples
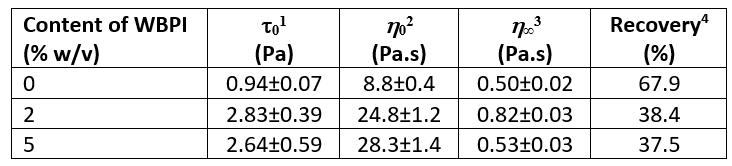
It can be observed that a lower protein content (2 % w/v) in the yoghurt composition influenced the flow properties of the measured samples. The yield stress of yoghurt significantly increased by adding 2 % w/v of WBPI, becoming independent of the protein isolate concentration at higher levels. While the addition of protein can modulate the textural properties of yoghurt, a higher content (5 % w/v) is counterproductive for the flow properties of yoghurts. Higher values of yield stress also contribute to increased kinetic stability in these samples, preventing phase separation of oily and water phases.
Samples with added protein exhibited higher values for both, zero-shear and infinite-shear viscosities, following a similar trend to the yield stress dependence. When a lower content of WBPI (<2 % w/v) was incorporated into the yoghurt composition, both parameters significantly increased compared to the reference sample without protein addition. This could impact the processability, kinetic stability, and swallowability of the samples, affecting the sensory analysis of yoghurts.
In summary, the relationship between flow properties and protein addition indicates that both textural and flow properties, especially yield stress and apparent viscosities, are significantly influenced by a relatively low content of WBPI (2 % w/v). These concentrations of protein lead to a substantial increase in yield stress, positively affecting the kinetic stability and textural properties of yoghurts. However, higher protein content in the yoghurt composition is inconsequential, as all significant parameters (especially yield stress and zero-shear viscosities) remain almost constant, considering the standard deviation, at the same values as the sample with 2 % w/v protein in the composition.
Flow cytometry analysis
One of the beneficial impacts of yoghurt consumption is its positive effect on gut health, attributed to Lactobacillus delbrueckii subsp. bulgaricus and Streptococcus thermophilus. These yoghurt culture bacteria provide numerous positive effects and therapeutic applications, such as enhancing the gastrointestinal mucus layer, stimulating the immune system, reducing lactose intolerance, and preventing lipid peroxidation (Dempsey and Corr, 2022; Fuller R., 1989). The quantity of live bacteria is thus directly related to the quality of the product.
As part of the experiments, the quantity of live yoghurt bacteria was determined using flow cytometry, and the impact of WBPI addition on cell viability was evaluated. The results are presented in Table 5.
Table 5. Number of bacteria cells in yoghurt samples
The results of the cytometric analysis showed that fortification of yoghurt by WBPI did not influence the viability of cells compared to the reference yoghurt. All samples contained more than 1.109 viable cells. The slight decrease in the number of cells per millilitre in samples containing protein, within one order of magnitude, was likely due to the presence of phenolic compounds (2.3±0.15 mg/g GAE), which are extracted during the isolation process. Phenolic acids are known to inhibit the growth of gram-positive lactobacilli strains (Cueva et al., 2010).
These results can be compared with the commonly used whey protein fortifier. In an earlier study conducted by Ranok et al. (2021), it was revealed that whey protein fortification can positively influence the number of viable cells in yoghurt during long-term storage and also during transit through the gastrointestinal tract. It was concluded that whey protein concentrate slowed down the damage to probiotic cell proteins, enhanced protein repair, and mitigated the effects of acidic environments (Begley et al., 2005; Vargas et al., 2015).
Sensory analysis
The reference yoghurt and WBPI-fortified samples were evaluated through sensory analysis, as described in the experimental section. The medians of the scores assigned to all descriptors are illustrated in the radar graph (Figure 4).
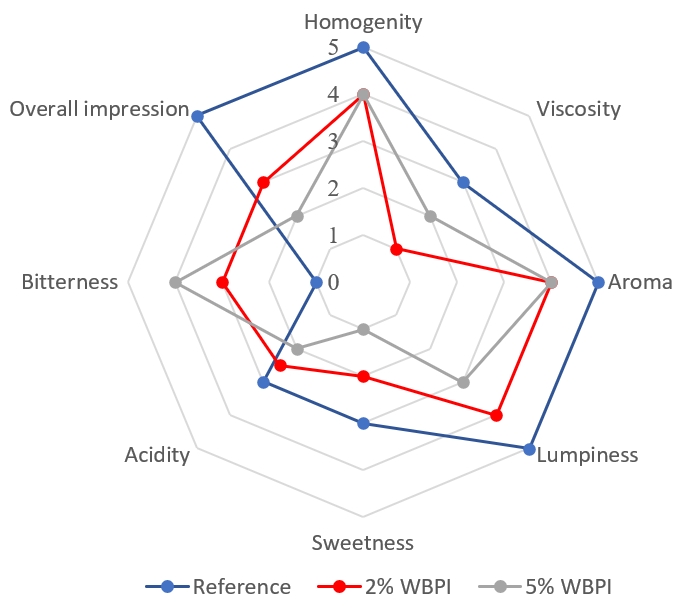
Figure 4. Radar graph of sensory descriptors scores of yoghurt samples
The descriptors were divided into texture, flavour, and overall impression groups. The addition of WBPI influenced all evaluated descriptors. Sensory analysis indicated that the addition of WBPI to yoghurt had a negative impact on consumer acceptability. Fortified samples exhibited sensory defects, leading to a decrease in the overall score with an increase in WBPI content. The overall consumer impression was correlated with most sensory descriptors (Table 6).
Table 6. Correlation coefficients of individual sensory descriptors

The overall score was mainly influenced by the bitterness descriptor, with a correlation coefficient of -0.8013. Bitterness in protein hydrolysates is often attributed to small molecular weight peptides interacting with taste bud cells (Aluko,2017). The relatively high concentration of hydrophobic amino acids, such as proline (9.5 mg/g), in WBPI may contribute to the bitterness in fortified yoghurts (Sonklin et al., 2018). Additionally, the proteolytic enzymes in the outer layers of wheat kernel can support the formation of small molecular weight peptides.
Several other potential causes of bitterness in WBPI-fortified yoghurt include the content of free non-volatile phenolic compounds, which can adhere to taste receptors, and the development of bitter taste during lipid oxidation due to wheat bran's lipoxygenase activity (Galliard, 1986; Heiniö et al., 2016; Cong et al., 2022).
Other taste descriptors (sweetness, acidity, aroma) were less affected by WBPI addition compared to the bitterness score. Slight decreases in sweetness and increases in acidity were observed with increasing WBPI concentration. The aroma was evaluated one point worse, likely due to the presence of grain scents from wheat bran.
Regarding textural descriptors (homogeneity, viscosity, lumpiness), WBPI addition increased dry matter and altered texture scores compared to the reference sample. The addition of non-milk protein can disrupt gel structure in yoghurts, leading to changes in texture descriptors. Homogeneity decreased, and lumpiness increased due to the presence of visible WBPI particles. Higher WBPI fortification (5 %) resulted in lumpier yoghurt, negatively perceived by the sensory panel. Resolving components' incompatibility in yoghurt samples could involve substituting milk with plant-based milk (e.g., soymilk). This substitution would eliminate the reliance on casein micelle chains and clusters for yoghurt gel formation (Xu et al., 2022).
Viscosity scores indicated that fortified yoghurts were stiffer compared to the reference yoghurt. The 2 % WBPI addition caused a more significant decrease in viscosity score than the 5 % addition, aligning with measured rheological characteristics, which showed the highest modulation of textural properties up to 2 % w/v protein addition. This observation is most probably related to the formation and stability of the yoghurt structure, which is negatively impacted by higher WBPI concentration.
Conclusions
The fortification of yoghurts with WBPI resulted in significant differences in product characteristics. The presence of WBPI altered not only the nutritional but also the physicochemical properties of yoghurts, with more pronounced changes observed with increasing WBPI addition. The presence of WBPI had a relatively positive impact on water-holding capacity (WHC) and viscosity, leading to reduced syneresis in fortified yoghurts. Interestingly, even a higher addition of WBPI (5 %) did not affect bacterial growth during fermentation. However, the original bitter taste of the protein isolate was not masked by the fermentation process and was notably detected during sensory analysis. The descriptor of bitterness emerged as the primary reason for the lower overall acceptability score of the fortified samples.
In conclusion, WBPI-fortified yoghurts cannot be considered comparable to regular yoghurts. It is crucial to acknowledge the inherent bitter taste of WBPI as a characteristic of plant protein-fortified yoghurts. Alternatively, efforts should be directed towards finding ways to mitigate the bitterness of WBPI to enhance product appeal for consumers.
Funding
This research was supported by Technology Agency of the Czech Republic, project no. FW02020135 and by project no. FCH-S-23-8330 financed by the Ministry of Education, Youth and Sports of the Czech Republic.
References
https://doi.org/10.3390/sports7010012
Moro, J., Tomé, D., Schmidely, P., Demersay, T., Azzout-Marniche, D. (2020): Histidine: A systematic review on metabolism and physiological effects in human and different animal species. Nutrients 12 (5), 1414.https://doi.org/10.3390/nu12051414