Introduction
Photosynthesis is an essential energy-converting process for producing complex carbohydrates and sustaining oxygenic life on Earth by releasing molecular oxygen. It involves light reactions taking place in the thylakoid membranes, and carbon fixation reactions in chloroplast stroma. Photosynthetic charge separation, electron transfer, and redox reactions, in synchrony, generate the proton motive force necessary for the synthesis of ATP and directing electrons towards the production of stromal reducing equivalent NADPH. The transport of photosynthetic electrons terminates at the stromal side of photosystem I, where the electrons are being primarily handed over from reduced ferredoxin (Fd) to ferredoxin:NADP+ oxidoreductase (FNR). Although in chloroplasts the main sink for electrons from ferredoxin is NADPH, depending on the organelle needs, other stromal acceptors can receive photosynthetic electrons as well. Such acceptors are oxygen in pseudocyclic electron transport (Allen 2003), ferredoxin-thioredoxin reductase, which is important for the regulation of carbon assimilation (Buchanan and Balmer 2005), nitrogen and sulfur assimilation reactions, the biosynthesis of chlorophyll, phytochrome and fatty acids (Hanke and Mulo 2013). Cyclic electron transport (CET) around PS I, where the electrons are returned from PS I to the cytochrome b6f complex (Joliot and Joliot 2006) is also possible.
The important regulator of this final linear electron transfer step is the thylakoid-membrane incorporated thylakoid rhodanese-like protein (TROL) that interacts with the FNR by dynamic tethering (Vojta et al. 2012, Vojta and Fulgosi 2012, Vojta and Fulgosi 2016, Kekić et al. 2020), and consequently influences the end point of photosynthetically derived electrons. TROL contains the binding region for the FNR (Jurić et al. 2009, Kekić et al. 2020) and seems to be the exclusive docking site for this protein (McKenzie et al. 2020). However, it has been shown that TROL is not essential for Arabidopsis growth and development (Jurić et al. 2009, Vojta et al. 2015). In TROL KO mutants, because there is no TROL to dock FNR, the photosynthetic electron transfer is not primarily directed to the production of NADPH, which is an electron donor for the Calvin-Benson cycle, but other electron acceptors are more likely to receive the electrons produced. Such acceptors are nitrite reductase, sulfite reductase, fatty acid desaturase, glutamine-2-oxoglutarate amino transferase, and ferredoxin-thioredoxin reductase (FTR). The FTR pathway constitutes an indispensable redox-signaling cascade for light-dependent reduction of chloroplast stromal proteins (Yoshida et al. 2022). FNR may also act as the direct ferredoxin:plastoquinone reductase (Fd:PQ), establishing redox regulation and antioxidant defense point (Jurić et al. 2013). Therefore, in the absence of TROL, the distribution of high-energy electrons is directed towards ROS scavenging pathways, rather than to the NADP+ reduction (Vojta et al. 2015). TROL knock-out (KO) plants show increased stress resistance along with significantly enhanced ROS scavenging (Vojta et al. 2015). Also, the absence of TROL leads to the complete loss of the dynamic FNR-binding and release to/from the thylakoid membrane (Vojta and Fulgosi 2016). Therefore, the TROL-FNR interaction seems to be an important mechanism for the regulation and prioritization of energy-conserving and energy dissipating pathways in vascular plant photosynthesis (Fulgosi and Vojta 2020).
Recently, the topology of TROL has been investigated in detail to define the localization and orientations of its domains (Vojta and Fulgosi 2019). TROL spans the thylakoid membranes with the two segments, one at the N-terminus of the protein and the other close to its C-terminal part. The C-terminal polyproline (PEPE) domain and FNR-interacting ITEP domain protrude to the chloroplast stroma and provide a flexible binding site for the FNR (Jurić et al. 2009, Vojta and Fulgosi 2019, Kekić et al. 2020). In the thylakoid lumen, encompassed by the two transmembrane helices, resides inactive rhodanese (RHO) domain, which most probably has the regulatory role (Jurić et al. 2009, Vojta and Fulgosi 2019).
TROL is one of the few known dually localized chloroplast proteins. Seventeen other proteins have been identified in both chloroplast envelope and thylakoid membranes (Klasek and Inoue 2016). They all carry an N-terminal signal for import into chloroplasts. These proteins are involved in protein transport, tetrapyrrole biosynthesis, membrane dynamics, transport of nucleotides and inorganic phosphate (Klasek and Inoue 2016). One of these proteins is Tic62, a redox sensor in the inner envelope membrane and the thylakoids, proposed to anchor FNR, just like TROL (Benz et al. 2010) and is involved in chloroplast protein import (Küchler et al. 2002). Besides the thylakoids-envelope, there can also be dual localization in the stroma-envelope compartments, as in the case of protein CPSAR1, which is dually localized in the stroma and the inner envelope membrane and is involved in thylakoid biogenesis (Garcia et al. 2010). Also, chloroplast-nucleus localization has been confirmed, as for the protein NPR1 which switches its location as adaptive response to salt stress (Seo et al. 2020). Finally, it was reported that approximately 5% of mitochondrion and chloroplast proteins are dually targeted in plants (Sáiz-Bonilla et al. 2023).
In addition to being mainly located at the stroma thylakoids, in its fully processed form of 66 kDa, TROL can also be found embedded in the chloroplast inner envelope membrane (IE) in its non-processed form of 70 kDa (Jurić et al. 2009, Vojta et al. 2018), with an as yet unraveled role. This dual localization has been proven by the proteome analysis of chloroplast envelopes and thylakoid membranes (Peltier et al. 2004). Both forms remain protected after thermolysin digestion of intact chloroplasts, indicating their complete incorporation into these membranes (Vojta et al. 2018).
During protein import into chloroplasts, the information contained in targeting sequences are sequentially decoded resulting in localization of the polypeptide to the appropriate organelle subcompartment (Cline and Henry 1996). TROL is a nuclear-encoded protein, synthesized with the cleavable N-terminal 69 amino acids long presequence that directs the protein to the chloroplasts. TROL is, like most of the IE membrane proteins, directed through the general import pathway (Toc and Tic complex) to cross the envelope membranes. This transfer might end at the level of the IE membrane, where we suppose TROL is released from the translocon machinery, as instructed by a hydrophobic stop-transfer signal in its sequence (Vojta et al. 2007). The majority of the TROL protein pool continues its way towards the thylakoids, by entering the chloroplast stroma where the preprotein is recognized and processed by the stromal processing peptidase (SPP), the mature protein being finally incorporated into thylakoid membranes (Vojta et al. 2007, Vojta et al. 2018). Proteins targeted to the thylakoid membrane require dual targeting signals that direct their import across the chloroplast envelope membranes and subsequent incorporation into the thylakoids. The targeting signal for thylakoids can be in the form of a single bipartite transit sequence that carries the information for targeting to stroma at the N-terminal region and to the thylakoids at the C-terminal region of the presequence (Cline and Henry 1996, Schnell 1998). Since the single targeting domain is predicted for the transit sequence of TROL, the signals for the targeting of TROL to the thylakoids seem to reside within the primary sequence of the mature polypeptide, as reported for other integral thylakoid membrane proteins, such as light-harvesting chlorophyll a/b binding protein (preLHCP) and the precursor to the 20-kDa subunit of the CP24 complex (Schnell 1998, Lamppa 1988).
In our previous experiments, we determined that a single amino acid mutation around the protease processing site of the presequence of TROL, Ala67Ile, directs the entire pool of in vitro synthesized and imported TROL to the chloroplast IE membrane, without incorporation in the thylakoids, and if so, then to a very low extent (Vojta et al. 2018). This result led to the idea of creating Arabidopsis plants that would accumulate TROL located at the single intrachloroplastic site – the IE. Such plants could unravel the still unknown function of TROL in this membrane. The importance of constructing TROL-IE plants lies in the fact that chloroplast envelopes make up only around 3% of total chloroplast membranes. The vast majority of membranes are thylakoids and by investigations performed on chloroplasts or total membrane extracts we are not able to properly investigate the role of TROL in the IE. By constructing TROL-IE plants we will be able, for the first time, to properly assess the role/s of TROL in the IE and the interactions with its IE-associated partners.
Plant material and breeding conditions
Several Arabidopsis lines have been used in this research. Arabidopsis thaliana (L.) ecotype Columbia (Col-0) plants, or wild-type (WT); TROL knock-out plants (KO), that do not accumulate protein TROL due to the T-DNA insertion into the gene At4g01050 (SAIL_27_B04, obtained from NASC) Col background (Jurić et al. 2009); TROL overexpression line (OX) that was constructed by transformation of KO A. thaliana plants with the plasmid vector pH7WG2.0 (35S promoter) containing TROL-HA-FLAG construct, in which the HA and FLAG tags were added C-terminally (Vojta and Fulgosi 2019); and finally TROL-IE plants, that contain TROL only in the inner envelope membrane of chloroplasts and were constructed in this research. All plants were grown under 16 / 8 light / dark period with a photosynthetic light intensity of 25 μmol photons m−2 s−1 (Osram Flora lamps) at 22 °C, relative humidity 60% during the day and 70% during the night. Plants were grown on a potting substrate (A400, Stender GmbH, Schermbeck, Germany) in a growth chamber (Kambič, Slovenia).
TROL presequence mutagenesis
Using the QuikChange Multi Site-Directed Mutagenesis method (Agilent Technologies, Santa Clara, California, United States), 67Ala → 67Ile substitution was introduced into the TROL presequence. Amino acids 67–78 of the sequence of TROL, AKSLTYEEALQQ, represent a partially conserved N-terminal part of the polypeptide, around the predicted transit peptide cleavage site. The substitution 67Ala→67Ile leads to the absence of processing of the transit peptide and the subsequent arrest of the protein TROL in the inner envelope membrane of chloroplasts (Vojta et al. 2018). Besides the Ala67 to Ile67 amino acid exchange FLAG (DYKDDDDK) and HA (Human influenza hemagglutinin, YPYDVPDYA) tags have been added to the C-terminus of the protein for easier downstream detection and purification. The whole construct was cloned into the pH7WG2.0 vector under the control of 35S promoter (Vojta et al. 2018).
Cultivation of Agrobacterium tumefaciens bacteria for transformation
Competent Agrobacterium tumefaciens EHA 105 bacteria were transformed chemically with the TROL(A/I)-FLAG-HA construct in the binary vector pH7WG2.0. Transformed Agrobacteria were multiplied by growth in liquid YEB medium with addition of rifampicin and spectinomycin in a horizontal shaker at 28 °C and 250 rpm until OD600 reached 1-1.5. The bacterial suspension was then transferred to tubes and centrifuged for 15 min at 1460 g. The centrifugation procedure was repeated to collect the remaining precipitate. The bacterial pellet was resuspended in infiltration medium with the addition of 0.03% (v/v) of the mild surfactant Silwet L-77 (Momentive Performance Materials GmbH & Co KG, Leverkusen, Germany) to lower surface tension and to enable bacteria to enter hard-to-reach parts of the flower during transformation by “Floral-dip” method.
Cultivation of A. thaliana TROL KO plants for transformation
Eighteen Arabidopsis TROL KO plants were grown in jars in a climate chamber as described. When the plants began to develop the primary inflorescence, it was necessary to cut it at the base, in order to break the apical dominance and allow the development of the secondary inflorescence. After 5-7 days, the stems with the secondary inflorescences were 2-10 cm long, representing the optimal stage for transformation.
In planta transformation of A. thaliana TROL KO plants by the "Floral-dip" method
Arabidopsis plants with developed secondary inflorescences were immersed in a suspension of Agrobacteria transformed with the TROL(A/I)-FLAG-HA construct in pH7WG2 vector, without using a vacuum (Clough and Bent 1998). This way, the transformation of the entire plants or seeds occurs, without the need for in vitro tissue culture. The pots with the plants were turned upside down and the inflorescences were immersed in a glass containing 500 ml of infiltration solution for 20-30 seconds, with gentle stirring of the solution with a glass rod. The plants were then placed horizontally on a plastic mat, covered with baking paper, and stored overnight in a climate chamber under low light conditions. On the next day, the jars with the plants were placed upright, filled with water, and exposed to light (~25 μmol photons m-2 s-1). The floral-dip procedure was repeated after 8 days. The plants were watered for the next two weeks, then the watering was stopped, and the plants were allowed to dry in order to collect the seeds. Seeds from each of the transformed plants were collected separately, and represented the T1 generation of the transformants.
Selection of transformed A. thaliana seeds
Sterilization of the seeds was carried out using 70% EtOH and 0.05% Triton X-100. Seeds were mixed in the solution by shaking for 5 min. Supernatant was discarded, followed by subsequent incubation with 70% EtOH for 10 min. The sterilization was finished by raising the seeds in sterile water. Following surface sterilization, seeds were selected for hygromycin resistance on solid MS medium (Moorashige and Skoog basal salt mixture, Sigma) containing 15µg/ml hygromycin. Seeds were stratified for 2 days in the dark at 4 °C and then transferred to the growth chamber for 4-6 h at 22 °C in continuous light (PAR 25 µmol photons m-2s-1) to stimulate germination. Plates were wrapped in aluminum foil and incubated for 2 days at 22 °C. The foil was then removed, and seeds were incubated for a further 48 h in continuous light (PAR 25 µmol photons m-2s-1). Seedlings with short hypocotyls were dismissed, while those with long hypocotyls were transferred to the soil to produce T1 plants and T2 seeds. The T2 seeds were selected by transferring seeds from each individual plant to the MS medium + hygromycin and treated as described previously. Only seeds from T1 plants (T2 seeds) that produced 70% seedlings with long hypocotyls and 30% with short ones were used in further selection steps. They developed into T2 plants and their T3 seeds were further selected on selective hygromycine plates. Only those with all long hypocotyls were supposed to be homozygous for the Ala67Ile67 mutation and were used further. T3 seeds and plants were tested by PCR, DNA sequencing and Western blotting. DNA isolation was performed using Phire Plant Direct PCR kit (Thermo Scientific™), and PCR was performed using the forward primer for TROL (5’ ggaatTCATGGAAGCTCTGAAAACCGCA 3’) and the reverse primer for FLAG_linker_HA tag (5’ CTTGTAGTCTAACTTGACAGCAGCAGCGTA). This way, only TROL from the transformed plants (containing FLAG and HA tags on the 3’ end of the gene) should multiply. Western blotting analyses were performed on total leaf extracts, intact chloroplasts, and chloroplast membranes from T2 plants by using anti-TROL (1:2000, Agrisera AS194257) and anti-HA antibodies (1:1000, Sigma H9658) and anti-rabbit IgG peroxidase (1:50000, Sigma A0545) as a secondary antibody for anti-TROL, and anti-rat IgG peroxidase (1:30000, Sigma A9073) as a secondary antibody for anti-HA. Plants with a stronger signal that were also PCR-positive were used for further investigations. Those were plants enumerated 8 and 18. Their sequence (mutation Ala67 to Ile67 GCT to ATT and C-terminal tags) was also successfully checked and confirmed by DNA sequencing. Therefore, the two mutant lines used in this study were designated TROL-IE8 and TROL-IE18, and these two lines were used for future experiments.
Production of the preTROL antiserum
The first 66 aminoacids of the presequence of TROL (MEALKTATFSPMSVLSEKRSEPRKPFSLPNLFPPKSQRPISQESFLKRFNGGLALLTSVLSSATAP) were custom synthetized and prepared for the immunization of two rabbits by the company Davids Biotechnologie Gmbh. (Regensburg, Germany). 15 mg of the peptide of purity >85% was synthetized, quality checked by HPLC and MS and conjugated to KLH carrier for immunization. The antigen in the concentration of 10 mg/ml was used for the immunization of two experimental animals. 35 days after immunization test, sera were taken from the rabbits and verified, together with the preimmune serum, for sensitivity and specificity to our plant extracts. Antiserum produced in this way (anti-preTROL) was expected to detect only the inner envelope membrane form of the TROL and not the thylakoid mature one. The immunization continued until day 63 to increase the titer of antibodies in the serum. From both sera samples, a part was purified on affinity matrix with the antigen. Final products were four different sera: serum 1 (anti-preTROL1), serum 1 affinity purified (anti-preTROL1AP), serum 2 (anti-preTROL2), and serum 2 affinity purified (anti-preTROL2AP). They were all tested (together with the preimmune sera) for specificity and sensitivity of TROL-IE detection in isolated chloroplasts of TROL OX and TROL IE plants. The optimal dilution of the anti-preTROL antisera was determined to be 1:100.
Isolation of total leaf extracts from Arabidopsis plants
Leaves of four-week-old Arabidopsis plants (WT, OX, IE-8, and IE-18) were cut and ground in liquid nitrogen using mortar and pestle. Laemmli buffer (Laemmli 1970) was added to the extract, samples were vortexed and heated at 70 °C for 5-10 min. Centrifugation at 13000 g for 5 min at room temperature pelleted the insoluble leftovers and the extracts were stored at -20 °C (for faster use) or at -80 °C (for later use).
Isolation of intact chloroplasts from Arabidopsis plants
Leaves of four-week-old Arabidopsis plants (WT, OX, IE-8, and IE-18) were cut and ground in ice-cold isolation medium (330 mM sorbitol, 20 mM TRIS/HCl, 5 mM EDTA, 10 mM Na2CO3, 0.1% BSA), filtered through 4-layered gauze and Miracloth filter (Sigma-Aldrich) and sedimented by centrifugation at 1500 g for 5 min, at 0 °C. The pellet was resuspended in a small amount of isolation medium and purified through 55%/35% Percoll density gradient. Centrifugation at 5000 g was performed for 10 min at 0 °C, in the swing-out rotor, with the slowest possible deceleration rate. Intact chloroplasts were collected from the interphase between 55% and 35% Percoll, washed twice in isolation medium, sedimented at 1500 g for 5 min at 0 °C, and chlorophyll concentration was measured spectrophotometrically.
Isolation of total chloroplast membranes from Arabidopsis plants
After the determination of chlorophyll concentration, intact chloroplasts corresponding to 2 µg chlorophyll were pelleted at 1500 g for 1 min at 0 °C, resuspended, and incubated in lysis buffer (10 mM Tris-base, 5 mM MgCl2, pH 7.9) for 30 min on ice. By subsequent centrifugation at 25000 g for 20 min at 4 °C the sample was separated to supernatant (stroma) and membrane pellet (consisting mostly of thylakoids). The pellet was resuspended in Laemmli buffer to the final concentration of 2 mg chlorophyll/ml buffer and stored at -20 °C until further use.
Western blot analysis
10-30 µg of isolated chloroplasts or thylakoids of the corresponding Arabidopsis line were loaded on 10% or 12% SDS-polyacrylamide gels and separated by electrophoresis (SDS-PAGE). Afterwards, Western transfer to the nitrocellulose membranes was performed, and membranes were immunodecorated with the anti-TROL (1:3000, Agrisera AS194257), anti-preTROL (1:100), and anti-HA antibodies (1:1000, Sigma H9658). As secondary antibody, anti-rabbit IgG peroxidase (Sigma A0545) was used for anti-TROL (1:50000) and anti-preTROL (1:10000) and anti-rat IgG peroxidase (1:30000, Sigma A9073) for anti-HA. Final detection was carried out by enhanced chemiluminescence (ECL) and exposure to X-ray films.
Results
Construction of Arabidopsis TROL-IE plants containing TROL only in the inner envelope membrane
In our previous work we exchanged several amino acids around the protease processing site of the TROL presequence. The mutation of Ala67 to Ile67 was the only one that influenced the processing of the protein, by arresting the TROL in the chloroplast inner envelope membrane (Vojta et al. 2018). In order to investigate in vivo the role of the TROL in this compartment, we had to construct the Arabidopsis plants containing TROL at this single location. Therefore, we have cloned the construct of TROL with the Ala67 to Ile67 amino acid exchange and the C-terminal FLAG (DYKDDDDK) and HA (Human influenza hemagglutinin, YPYDVPDYA) tags in the pH7WG2.0 vector. This construct was used to transform TROL KO Arabidopsis plants. Seeds from transformed plants were collected (T1 generation) and homozygous TROL-IE plants were selected up to the T3 generation. Selection for hygromycin resistance, PCR, and testing for expression of recombinant proteins by Western blot analysis, in combination, enabled us to select the homozygous plants carrying TROL only in the IE of chloroplasts. The two homozygous mutant lines that we selected for future investigations were named TROL-IE8 and TROL-IE18.
As controls, we used AtTROL containing the entire wildtype sequence (WT), as well as plants overexpressing TROL, with C-terminal FLAG and HA tags (OX). Whole leaf extracts, isolated chloroplasts and chloroplast membranes were tested for the presence of TROL and HA-tags in these lines. Both antibodies (anti-TROL and anti-HA) could detect the newly transformed protein in the inner envelope membrane (IE8 and IE18, Fig. 1A lanes 6-9 and 17-20). Also, TROL was successfully recognized by the anti-TROL and anti-HA in the overexpressor line (Fig. 1A, lanes 11-15). Anti-HA antibody did not recognize TROL or any other protein in the WT plants, since in those plants there was no HA-epitope. Due to a stronger background in all tested extracts, we attempted to analyze the lower amount of intact chloroplast samples (Fig. 1B). In this way, we greatly reduced unnecessary background bands and once again confirmed that the IE plants express the modified TROL.
Although we have successfully confirmed the presence of TROL in the IE plants, we did not manage to confirm the exact molecular weight of these proteins on the SDS-PAGE, even when using strongly denaturating urea gels (data not shown, Sirpiö et al. 2011). The calculated size difference between the precursor and the mature form of the TROL is only 4 kDa. To prove that the protein TROL that we detected in the IE plants was indeed the precursor, and indeed only in the inner envelope membrane, we had to develop a method that would recognize only the precursor of the TROL and not its processed form.
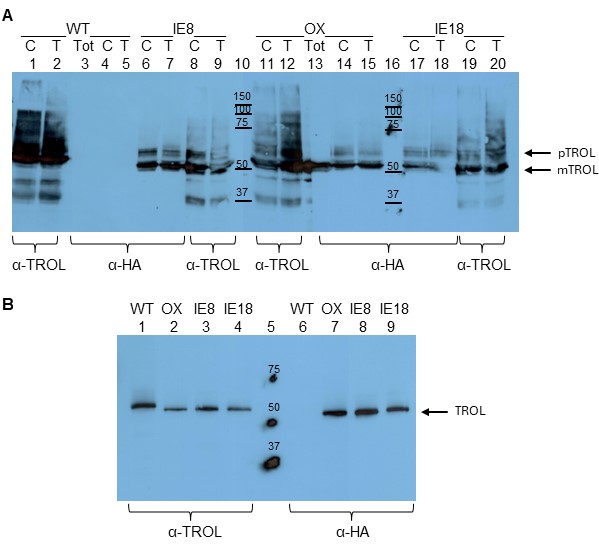
Fig. 1. Western blot analysis of A. thaliana TROL KO plants transformed with the TROL(A/I)-FLAG-HA construct. A. Wild type (WT, Lanes 1-5), TROL overexpressor (OX, Lanes 11-15) and TROL inner envelope lines of Arabidopsis (IE8, Lanes 6-9, and IE18, Lanes 17-20) have been analyzed. Total leaf extract (Tot), intact chloroplasts (C) and total chloroplast membranes (T) in the amount corresponding to 30 µg chlorophyll were analyzed by 12% SDS-PAGE and Western blotting. Membranes were immunodetected with anti-TROL (α-TROL) and anti-HA antibodies (α-HA). Conjugated secondary antibodies anti-rabbit IgG peroxidase for anti-TROL, and anti-rat IgG peroxidase for anti-HA, have been used. Results were visualized by enhanced chemiluminescence (ECL) and exposure to X-ray films. Lanes 10 and 16 represent protein markers in kDa. Arrows denote the positions of TROL preprotein (pTROL) and mature TROL (mTROL). B. Intact chloroplasts of WT, OX, IE8 and IE18 lines in the amounts corresponding to 5 µg chlorophyll were analyzed by 12% SDS-PAGE and Western blotting, as described for A. Lane 5 represents protein marker in kDa. Arrow denotes the position of TROL (since there is less sample loaded on gel, preprotein is not visible).
Phenotype of TROL-IE plants
Arabidopsis IE8 and IE18 lines grew well under the same conditions as the WT plants. Their hypocotyls developed later (Figure 2A); they grew somewhat slower due to the delayed germination (Fig. 2). At 3.5 to 4 weeks of age, before flowering starts, the plants are most suitable for biochemical experiments. At this stage TROL-IE plants had smaller rosettes than the WT, and smaller biomass. As they grew, their stems seemed to be slightly thinner than the stems of WT. The flowering was slightly delayed, and they produced a significantly smaller number of siliques and seeds than the WT. Otherwise, they resembled the phenotype of WT Arabidopsis.
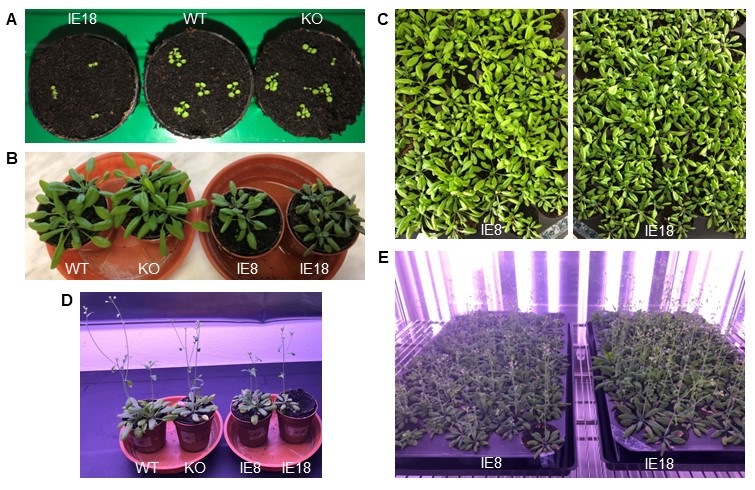
Fig. 2. The phenotype of Arabidopsis plants that contain protein TROL only in the inner envelope membrane of chloroplasts (TROL-IE plants). A. 12 days old TROL-IE plants in comparison to the WT and TROL KO. B. and C. 4 weeks old plants. D. 5 weeks old plants that started flowering. E. 9 weeks old plants. WT denotes wild-type Arabidopsis plants ecotype Columbia (Col-0); KO denotes Arabidopsis TROL knock-out mutant; IE8 and IE18 are Arabidopsis mutant lines that contain TROL only in the inner envelope membrane of chloroplasts.
Production of the antibody against the presequence of TROL
Since anti-TROL antibody recognizes both the inner envelope membrane (precursor) form and the thylakoid membrane-located (mature) TROL protein, we decided to produce additional antibody against the N-terminal presequence of the protein (not recognized by existing anti-TROL). For that purpose, synthetic polypeptide of 66 N-terminal amino acids of TROL presequence was produced by custom peptide synthesis (Davids Biotechnologie Gmbh., Regensburg, Germany). There were two sera produced: serum 1 (anti-preTROL1) from rabbit 1 and serum 2 (anti-preTROL2) from rabbit 2. Both sera were rather unspecific with strong background (Fig. 3, Lanes 3, 4, 10, 11). Therefore, both sera were purified on affinity matrix with the bound antigen (synthetic polypeptide of 66 N-terminal amino acids of TROL presequence). Final products after purification were: serum 1 affinity purified (anti-preTROL1AP) and serum 2 affinity purified (anti-preTROL2AP). They seem highly purified and specific (Fig. 3, Lanes 5, 6, 12, 13), just of a very low titer. Therefore, we determined experimentally that a dilution of 1:100 was the highest required for the proper detection of the TROL precursor. Between those two purified sera, anti-preTROL2AP proved to be a bit stronger and therefore we decided to use it in further experiments.
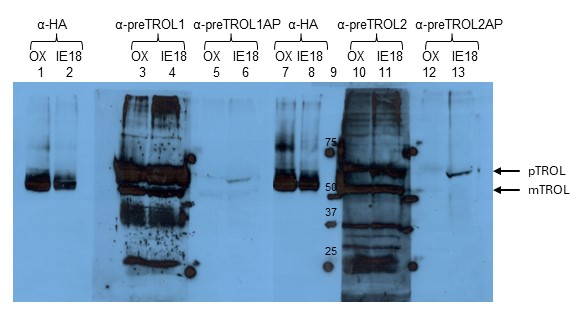
Fig. 3. Testing the anti-preTROL sera on OX and IE18 Arabidopsis lines by Western blot analysis. TROL overexpressor (OX) and TROL inner envelope membrane IE8 lines of Arabidopsis have been analyzed. Intact chloroplasts in an amount corresponding to 10 µg chlorophyll for anti-HA analyses and 40 µg chlorophyll for anti-preTROL analyses were separated by 10% SDS-PAGE, transferred to a nitrocellulose membrane, and immunodecorated with the anti-preTROL (α-preTROL) and anti-HA antibodies (α-HA), as a control. Conjugated secondary antibodies anti-rabbit IgG peroxidase for anti-preTROL, and anti-rat IgG peroxidase for anti-HA have been used. Results were visualized by enhanced chemiluminescence (ECL) and exposure to X-ray films. Lane 9 represents the protein marker in kDa. Arrows denote the positions of TROL preprotein (pTROL) and mature TROL (mTROL). Non-purified anti-preTROL antisera (α-preTROL1 and α-preTROL2, Lanes 3, 4, 10, 11) seem to be unspecific. Affinity purified anti-preTROL antisera (α-preTROL1AP and α-preTROL2AP, Lanes 5, 6, 12, 13) seem to be very specific, showing a single clear band in TROL-IE plants, indicating TROL precursor.
After the preTROL antiserum was successfully produced, we tested the chloroplasts from OX and IE lines by SDS-PAGE and Western blot analysis, as described previously. We used anti-HA and anti-preTROL antisera. Affinity purified anti-preTROL clearly recognized only a single band in TROL-IE8 and TROL-IE18 lines (Fig. 3, Lines 6 and 13; Fig. 4, Lanes 2 and 3) and not in the TROL OX line (Fig. 3, Lines 5 and 12; Fig. 4, Lane 1). Anti-preTROL that was not affinity purified did not differentiate between TROL-IE and TROL OX lines (Fig. 3, Lanes 3, 4, 10, 11), except that the resulting signal/band was more intensive in TROL-IE line (Fig. 3, Lanes 4, 11). When compared to the anti-HA blots on TROL-IE lines, it was clear that the affinity purified anti-preTROL recognized the precursor and not the mature form of TROL. A very weak signal was observed on the TROL OX line (Fig. 3, Lanes 5 and 12). It corresponded to the IE form of TROL in this line, which was in the vast minority in comparison to the thylakoidal form of TROL. This weak signal was visible in TROL OX extracts only when a sample corresponding to 40 or more μg chlorophyll was loaded on gel. These experiments confirmed that TROL is located exclusively in the inner envelope membrane in TROL-IE8 and TROL-IE18 Arabidopsis lines, and that the affinity purified preTROL antiserum can be further used to exclusively detect the inner envelope membrane form of TROL.
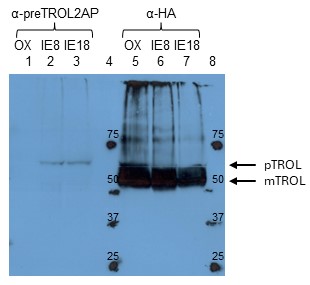
Fig. 4. Detection of TROL in OX, IE8, and IE18 Arabidopsis lines by using anti-preTROL serum. Intact chloroplasts in an amount corresponding to 40 µg chlorophyll for anti-preTROL analyses (Lanes 1-3) and 10 µg chlorophyll for anti-HA analyses (Lanes 5-7) were separated by 10% SDS-PAGE, transferred to a nitrocellulose membrane and immunodecorated with anti-preTROL (α-preTROL2AP) and anti-HA antibodies (α-HA), as a control. Conjugated secondary antibodies anti-rabbit IgG peroxidase for anti-preTROL, and anti-rat IgG peroxidase for anti-HA, have been used. Results were visualized by enhanced chemiluminescence (ECL) and exposure to X-ray films. Lanes 4 and 8 represent a protein marker in kDa. Arrows denote the positions of TROL preprotein (pTROL) and mature TROL (mTROL). Anti-preTROL2AP serum seems to be very specific, showing the single clear band in TROL-IE plants (lanes 2 and 3), indicating TROL precursor. Anti pre-TROL serum does not show visible signal on OX line because there is too little amount (undetectable) of the TROL precursor in this sample.
Analysis of TROL localization in TROL-IE plants
By newly produced anti-preTROL affinity purified sera we confirmed that the TROL in these plants was found only in precursor form in the inner envelope membrane (Fig. 4). TROL OX line was a good control for determination of the localization of TROL since, just as TROL-IE8 and TROL-IE18 lines, it was under the control of a strong constitutive promotor 35S and has C-terminally added FLAG and HA tags for easier detection and purification. Therefore, when using anti-HA antibody, we managed to detect TROL in all three lines (OX, IE8, and IE18, Fig. 4, Lanes 5-7). However, when using anti-preTROL serum, TROL was detected only in the IE lines (Fig. 4, Lanes 2 and 3). This observation confirmed that the accumulation of the precursor due to mutagenesis occurred in the inner envelope membrane. OX line lacks the signal because the majority of the TROL in this line is in the thylakoids, as a mature form of the protein, which cannot be recognized using antibodies against the presequence.
Discussion
The role of the TROL in the thylakoid membranes has been investigated for more than 15 years, since the protein was characterized in 2009 (Jurić et al. 2009). TROL has been shown to be involved in photosynthetic electron distribution by docking the FNR to the thylakoid membranes and therefore bringing it to the vicinity of PSI, namely ferredoxin (Fd) (Jurić et al. 2009, Kekić et al. 2020). Fd distributes high-energy electrons to a multitude of enzymes involved in chloroplast metabolism. By the interaction of TROL and FNR, electrons are preferentially directed to carbon assimilation, which requires NADPH, and so the majority of Fd is immediately oxidized by the enzyme ferredoxin:NADP+ oxidoreductase (FNR), which associates with the thylakoid membrane (Jurić et al. 2013).
Apart from thylakoids, TROL resides in the inner envelope membrane (IE) and its role in this compartment has remained unexplored to date. This is because the smaller portion of TROL precursor in the IE is being masked by the majority of the mature form of TROL located in the thylakoids. Dual localization has been shown for other chloroplast proteins of various functions (Klasek and Inoue 2016). In some cases, protein is dually localized because its activity is needed in more than one location. Cells usually either use multiple gene products (differently located isoforms) or target a single gene product to multiple locations by various mechanisms (Klasek and Inoue 2016). At this stage we can only speculate on the role of TROL in the IE membrane. It might represent a “storage” from which TROL is mobilized to thylakoids when needed. There is also a possibility that TROL in the IE membrane is someway involved in the electron transfer at this membrane. Mass spectroscopy analyses (Vojta et al. 2023) indicated its possible interaction with Tic62 and Tic20, proteins that build a Translocon at the chloroplastic inner envelope membrane. This might indicate involvement of TROL in a protein translocation process across the chloroplast envelope, probably as a possible additional redox regulator through interaction with FNR. There is also a possibility that IE membrane TROL is involved in metabolic regulation by docking FNR and regulating NADPH production in this compartment. To test these hypotheses and to investigate its role in the inner envelope membrane of chloroplasts in vivo, we had to “remove” TROL from the thylakoids.
In our previous research, by Western blotting of isolated outer and inner chloroplast envelope membranes, thylakoids, and stroma, we confirmed that TROL preprotein is associated almost exclusively with the inner envelope membrane and that the mature part is located in the thylakoids (Jurić et al. 2009). We managed to find the determinants for the dual localization of TROL around its presequence protease processing site (Vojta et al. 2018). We mutated Ala to Ile at the position 67 in TROL precursor and by performing in vitro protein import experiments we proved that the majority of such preprotein was not processed and transferred to its destination in the thylakoid membrane (Vojta et al. 2018). These results provided us with the tool to “remove” TROL from the thylakoids for the purpose of studying its function in the inner envelope membrane. By applying the “Floral dip” method of Agrobacterial transformation of Arabidopsis plants with the TROL knock-out background with the mutated construct TROL(A/I)-FLAG-HA in pH7WG2.0 vector we successfully managed to create plants that contain TROL only in the inner envelope membrane (TROL-IE plants). These plants were selected on selective growth medium with the addition of specific antibiotic and tested by PCR, DNA sequencing, and Western blotting at different stages of the selection process (Figure 1). Plants that were selected as homozygous were named TROL-IE8 and TROL-IE18.
TROL-IE8 and TROL-IE18 plants grow well throughout the whole life cycle and produce viable seeds. In comparison to the WT Arabidopsis, their development is delayed. Their hypocotyls develop several days later, and they grow slower due to delayed germination (Figure 2). TROL-IE plants have smaller rosettes and weaker stems, and their flowering is delayed. Otherwise, they resemble the phenotype of the wild type of Arabidopsis. The life cycle of the TROL-IE plants surprisingly does not resemble the TROL KO plant cycle. TROL KO plants, in which there is no production of TROL, follow the WT developmental cycle, and their hypocotyls, rosettes and seeds are all slightly bigger than the WT (Jurić et al. 2009, Vojta et al. 2015).
Although we confirmed which plants were successfully transformed, by using SDS‑PAGE and subsequent Western blotting using anti-TROL or anti-HA antibodies, it was not possible to differentiate the precursor and the mature form of TROL according to the difference in size and therefore not possible to determine whether it was localized in the inner envelope membrane or/and in thylakoids. The reason for this is that when using anti-TROL or anti-HA in most cases a bulky signal was visible on gel. The addition of 8 M urea in the samples and/or in the gel itself (data not shown) also did not help to reliably differentiate the thylakoidal (66 kDa) vs inner envelope membrane form of TROL (70 kDa). Therefore, it was essential to produce the antiserum that would recognize only the precursor form of TROL. We selected N-terminal 66 amino acids of TROL to custom produce a polypeptide, an antigen that would, by immunizing experimental animals, give a specific antiserum. Anti-preTROL antiserum (antiserum against the N-terminal presequence of TROL) was successfully produced (by Davids Biotechnologie Gmbh., Regensburg, Germany). While testing it, it was clear that the antiserum must be further affinity purified, since it showed a strong background signal when we assessed the plant extracts. Anti-preTROL serum that was not affinity purified did not differentiate between TROL-IE and TROL OX lines, except that the resulting signal/band was more intensive in the TROL-IE line. Affinity purified antiserum proved to be highly specific, recognizing a single clear band in TROL-IE lines, but not in the TROL OX line. A weak visible signal in the TROL OX line was present only when there was a lot of sample loaded on gel. This is because TROL preprotein in TROL OX line was present in extremely small amounts, while the vast majority of TROL was in thylakoids, as a mature form.
We analyzed the chloroplasts from WT, TROL OX, TROL KO, and TROL-IE lines by SDS-PAGE and Western blotting, as described in Materials and Methods section. We used anti-TROL, anti-HA, and anti-preTROL sera. It was clearly visible that there was no signal using any of the antisera in TROL KO plants (data not shown). Affinity purified anti-preTROL clearly recognized only a single band in TROL-IE 8 and TROL-IE 18 lines and not in the TROL overexpression line (Figures 3 and 4). When compared to the anti-HA blots on TROL-IE lines, it was clear that affinity purified anti-preTROL recognized the precursor form of TROL and not the mature one (Figure 4). When using the anti-HA antibody, mature form of TROL could be detected also in the IE lines (Figure 4 Lanes 6 and 7), however in lesser amount than in the OX (Figure 4 Lane 5). In the IE lines processing of TROL still occurred to some extent (Vojta et al. 2018) and mature TROL was visible because of the much larger amount of thylakoids, although the prevailing amount of TROL was in the inner envelope membrane. Therefore, when taken together with the previous localization results (Jurić et al. 2009, Vojta et al. 2018), these experiments confirmed that in the TROL-IE8 and TROL-IE18 Arabidopsis lines TROL is located almost exclusively in the inner envelope membrane, and that the affinity purified anti-preTROL antiserum can be further used to exclusively detect the inner envelope form of TROL. By these experiments we provided the tool to investigate TROL in the inner envelope membrane of chloroplasts: its role in organelle metabolism and energetics, abiotic stress response, its interaction partners, and finally, its possible role in electron transfer at the level of the IE.
Author contribution statement
L. V. devised the research concept, acquired funding, designed, and performed all experiments except the floral dip transformation, analyzed results, and wrote the manuscript. H. F. co-designed experiments, discussed results and reviewed the manuscript. A. T. P. performed floral dip transformation. E. D. grew experimental plants.