INTRODUCTION
Extending the lifespan of the world's population is certainly an achievement of the current century, even so generating many challenges to which we as a society must constantly respond. The increasing frequency of chronic diseases, simultaneously with the diagnosis of new ones, dictates the development of innovative drugs centric on individuals and adapted to different age groups, especially the pediatric population and older adults. One of the pharmacy responses to those issues comprises expanding the development of biological drugs, especially for subcutaneous administration. Biopharmaceuticals are a group of drugs that are gaining in importance, especially in the field of diseases that had worse prognoses or were completely incurable before the revolution of these drugs. This is in particular related to the high selectivity and target specificity of biological drugs (1). Still, the development of protein drugs is very challenging and dictates new pharmaceutical technologies and adaptations of manufacturing conditions, reflected in high drug prices. Due to the instabilities of biological drugs and their susceptibility to degradation in the gastrointestinal tract, parenteral dosage forms are the most common dosage forms for biopharmaceuticals. The majority of marked biopharmaceuticals are still in a form for intravenous ( i.v.) route of administration, nevertheless, subcutaneous application ( s.c.) is becoming the application of choice in treatment with biologicals (2, 3). This preference is related to the appropriateness of s.c. application to be performed less periodically and by patients themselves in the home environment, being of particular importance for chronic patients, children, and older adults, overall contributing to reduced cost of treatment as well as sustainability (4, 5).
Appropriate dosage form and manufacturing process require special consideration with a designed approach within the development of s.c. protein formulations. The most limiting factor in s.c. application is a small injection volume, i.e., below 2 mL, thus highly concentrated protein formulations are required. The concentration of s.c. protein formulation thus exceeds 100 mg mL–1, which poses several issues regarding protein stability and physicochemical properties of the solution (5). Protein molecules are for the most part prone to chemical and physical instability, where the aggregation tendency of molecules increases with increasing protein concentration (4). In order to overcome this issue inclusion of appropriate excipients is of crucial importance, whereby, in addition to buffers, which maintain optimal pH, surfactants, and stabilizers as sucrose, have to be added. Stabilizers restrict protein interactions and form hydrogen bonds with protein molecules as a water substitution. Additionally, protein surface adsorption is prevented by surfactants, usually polysorbates. Although the liquid formulation is the preferred dosage form for injectable protein drugs, the degradation rates are significantly reduced in a dried state. Lyophilisation, a drying method based on the sublimation phenomenon, is commonly implemented to prevent protein instabilities that would potentially occur in liquid forms (6, 7). The lyophilisation cycle starts with freezing, where the initial solution is transformed into ice, and removed during primary drying. To achieve the lowest residual moisture by desorption of unfrozen water, the secondary drying step is performed (7). Lyophilisation is a complex and expensive technological process, therefore optimization of formulation and process parameters is essential. Thus, critical quality attributes, such as reconstitution time and cake appearance of final lyophilisates, have to be provided. In this regard, crystallizing bulking agents, e.g., mannitol, are required. Namely, highly concentrated protein formulations in lyophilised states are faced with prolonged reconstitution time, which is one of the greatest bottlenecks in the development of new biopharmaceuticals for s.c. administration (8). Moreover, the increased viscosity of such formulations is another crucial parameter in terms of optimizing s.c. delivery as it hinders the injectability.
The aim of the present research work was to gain a better understanding of the challenges associated with the development of highly concentrated protein formulations for s.c. injections, thus contributing to sustainability paradigm integration. Firstly, the effect of bovine serum albumin (BSA) concentrations on solution viscosity as the most limiting attribute in terms of s.c. delivery was investigated. Then, for the same formulations, i.e., with varied protein concentrations from 5 to 100 mg mL–1 in phosphate buffer, the effect of formulation composition on reconstitution time and cake appearance was evaluated. To demonstrate the protective effect of sucrose, the dynamic light scattering method was utilized. Since bulking agents are typically added in solutions for lyophilisation, the effect of mannitol on critical quality attributes of lyophilisates was also under investigation. Findings acquired within this study are of great importance, since they represent good foundations for further optimization of the protein formulations development, together with adaptations of technological procedures, processes, and analytical methods for evaluation. As much, the obtained know-how is an update of an existing platform related to research in the field of biopharmaceuticals with larger molecular weight proteins, such as monoclonal antibodies. All of that contributes to reducing the number of experiments based on the “trial and error” principle.
Bovine serum albumin (BSA; Glentham Life Science, United Kingdom), with a purity of more than 96 % was selected. The sucrose used is suitable for biopharmaceutical production (≥ 99.5 % purity; D+; Merck, Germany), and the mannitol is suitable for use as an excipient (bulking agent; > 99 % purity; Merck, Germany). Polysorbate 20, sodium hydroxide, and sodium dihydrogen phosphate monohydrate were from Merck, Germany. Ultra-pure water was obtained from a Milli-Q purification system (A10 Advantage; Millipore Corporation, USA).
Formulations
BSA in concentrations of 5, 20, 50, 70, and 100 mg mL–1 was dissolved in 0.2 mol L–1 filtered (0.45-µm RC membrane filters; LLG Labware, USA) phosphate buffer. BSA solution was compounded with sucrose (in mass ratio 1:1) or sucrose and mannitol (in mass ratio 1:1:0.5) and 0,05 % ( m/V) polysorbate 20. After readjusting pH, 2 mL of the initial solution was again filtered and aliquoted into 6 mL vials for lyophilisation (Schott Type I plus, Germany).
Lyophilisation procedure
Differential scanning calorimetry
Differential scanning calorimetry measurements (DSC 1; Mettler Toledo, Switzerland) were performed to measure the glass transition temperature of maximally freeze-concentrated solutions ( Tg'). Hermetically sealed sample solutions in an aluminum pan were first cooled from 25 °C to –80 °C (cooling rate: 1 °C min–1), and then heated to 90 °C (heating rate: 10 °C min–1). Tg' and melting endotherms were reported as an inflection point during the heating scan. All measurements were performed under a nitrogen atmosphere (50 mL min–1 ).
Rheological measurements
Rotational and oscillatory rheological tests were performed for BSA solutions using a Physica MCR 301 rheometer (Anton Paar, Austria) with a double gap measuring system DG26.7/T200/SS (sample volume 10.0 mL). The rotational tests were used to determine the viscosity, while frequency tests were used to define the storage (G′) and loss (G′′) moduli of the solutions. The shear rate during the rotational tests ranged from 1 to 100 s−1, whilst the frequency measurements were performed at a constant amplitude (4 %) at an angular velocity of 100–0.1 rad s–1. Prior to frequency measurements, amplitude tests at constant angular velocity (10 rad s–1) and shear strain continuously increased from 0.01 to 100 % were performed and as G′ was practically immeasurable, an amplitude for frequency tests was chosen based on stable system response (Fig. A, Supplementary File). All the measurements were performed at 25.0 ± 0.1 °C under Peltier temperature control.
Product appearance and reconstitution time
Lyophilised products were evaluated after each lyophilisation process, whether they were ‘collapsed’, ‘non-collapsed’, or ‘partially collapsed’. The reconstitution times were determined by the dissolution of the lyophilised products in the primary packaging. An adequate amount of ultrapure water was injected into the vial that was then gently swirled for 10 s. If the sample had not completely dissolved, the 10 s steps were repeated until complete dissolution. The reconstitution endpoint was considered when the cake dissolved completely, leaving no visible residue.
Dynamic light scattering
The particle sizes and polydispersity index of the formulations were determined by dynamic light scattering (Nano ZS Zetasizer; Malvern Instruments, UK). The samples were measured in polystyrene cuvettes (Sarstedt AG & Co, Germany) at 25 °C before and after lyophilisation, using deionised water as the solvent (viscosity, 0.8872 mPa s; refractive index, 1.330). Each sample was analysed in triplicate, and the data are expressed as mean diameters and polydispersity index values.
RESULTS AND DISCUSSION
Thermal characteristics of pre-lyophilised solutions
Lyophilisation, specifically primary drying, has to be properly optimized to avoid prolonging the process and thus additional costs. Primary drying temperature depends on the thermal properties of the dried formulations, with Tg' being one of the most important. Tg' is strongly related to the excipients and their physical states during freezing and drying. Thus, our purpose was to explore the influence of mannitol, sucrose, and BSA mixtures on formulations Tg'.
No Tg' was observed on the heating thermogram (Fig. 1a) for 5 mg mL–1 BSA formulations irrespective of the presence of mannitol indicating that formulations most likely exhibit complete crystallisation behaviour during freezing. This is in accordance with a study performed by Dixon et al., where it was reported that protein i.e., Fc-fusion protein concentrations in a range of 1–5 mg mL–1 altered the crystallization of mannitol, while by increasing protein weight fraction the crystallization is inhibited by amorphous material ( i.e., protein and sucrose) (9). We assume that BSA at 5 mg mL–1 has a strong tendency to molecular interactions with mannitol, while sucrose interactions with mannitol are restricted. Then again, by increasing BSA concentrations, amorphous characteristics of formulations through the expression of Tg' on DSC heating scans were confirmed. BSA formulations in the concentration range 20–100 mg mL–1 with sucrose (Fig. 1b-e) displayed comparable Tg' values, namely around –32 ºC for all formulations. The Tg' for an aqueous solution of sucrose without incorporated BSA was reported as –35 °C for (10). This points towards BSA's capacity to increase Tg '. As indicated in Fig. 1b-e formulations with mannitol added showed lower Tg', which was raised by increasing BSA concentrations, with a minimum of –41.9 °C for formulation with 20 mg mL–1 BSA to a maximum of –35.4 °C for 100 mg mL–1 BSA.
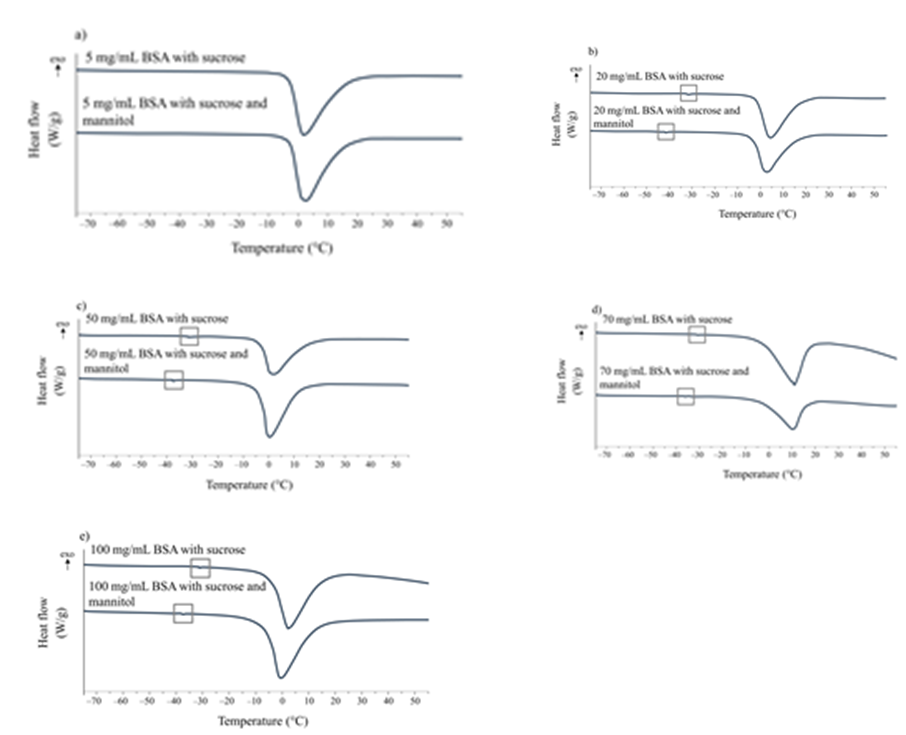
Fig. 1. DSC 1st heating thermograms representing thermal events for pre-lyophilised liquid formulations containing sucrose or sucrose and mannitol with different concentrations of BSA: a) 5 mg mL–1, b) 20 mg mL–1, c) 50 mg mL–1, d) 70 mg mL–1, and e) 100 mg mL–1. The grey square indicates Tg'.
Despite detectable Tg', they were not well defined, indicating at least partial crystallization of mannitol that was confirmed by eutectic melting of mannitol and ice, observed during all heating scans. Lower Tg' values obtained for formulations with mannitol are a contribution of the amorphous part of mannitol. This is with great certainty the consequence of a higher sucrose amount compared to mannitol (ratio 1:0.5, respectively), where the stabiliser hinders the complete mannitol crystallization. Since, as explained below, mannitol did not show beneficial effects either in the appearance or in the reconstitution time of BSA lyophilisates, it is reasonable to assume that for lyophilisation of low molecular weight proteins with sucrose in excess, incorporation of mannitol is not necessary. However, the effect of mannitol and the extent of its crystallisation is formulation- as well as protein-specific (11). To prevent protein drug instabilities due to further mannitol crystallization during storage, i.e., in lyophilisates with only partially crystallized mannitol achieved during the process, the maximum concentration of non crystallising stabiliser not yet hindering the mannitol crystallisation, must not be exceeded.
Rheological characterization of (pre-)-lyophilised formulations
Rotational measurements were applied to define the features of flow properties under an applied stress for each concentration of BSA solutions with and without mannitol added in general providing information in view of injectability on top of manufacturability (12). In the case of formulations with no mannitol added an increase of viscosity was observed with increasing BSA concentration, being in line with what has been reported throughout the literature with regards to serum albumin solutions (13). The same effect observed also for BSA solutions with mannitol, and what is more, mannitol did not affect viscosities as they were practically the same for corresponding solutions of the same BSA concentrations without or with mannitol added, i.e., ranging from 1.30 to 2.07 mPa s or 1.28 to 2.09 mPa s at the lowest measured shear stress ( i.e., 1 s –1), respectively (Fig. 2). The observed viscosities are very under viscosity limit ( i.e., 30–50 mPa s) that still allows normal syringeability (14). As an additional benefit, throughout the range of BSA concentration tested and the applied shear rates, viscosity was independent of shear rate indicating a Newtonian-like behaviour. This is a clear contrast to what has been observed in previous studies of the rheology of globular proteins where an apparent yield behaviour has been reported particularly at lower shear rates when measured with a double gap geometry. Then again, if measured by a microfluid slit rheometer or viscometer-rheometer-on-a-chip (VROC) Newtonian behaviour with viscosities in a comparable range was observed (15, 16). Observed purely viscous Newtonian-like behaviour is likely due to the presence of polysorbate-20, a widely used surfactant in biopharmaceutical formulations, as similar rheological behaviour has been reported for globular protein in buffer solutions containing polysorbate (12).
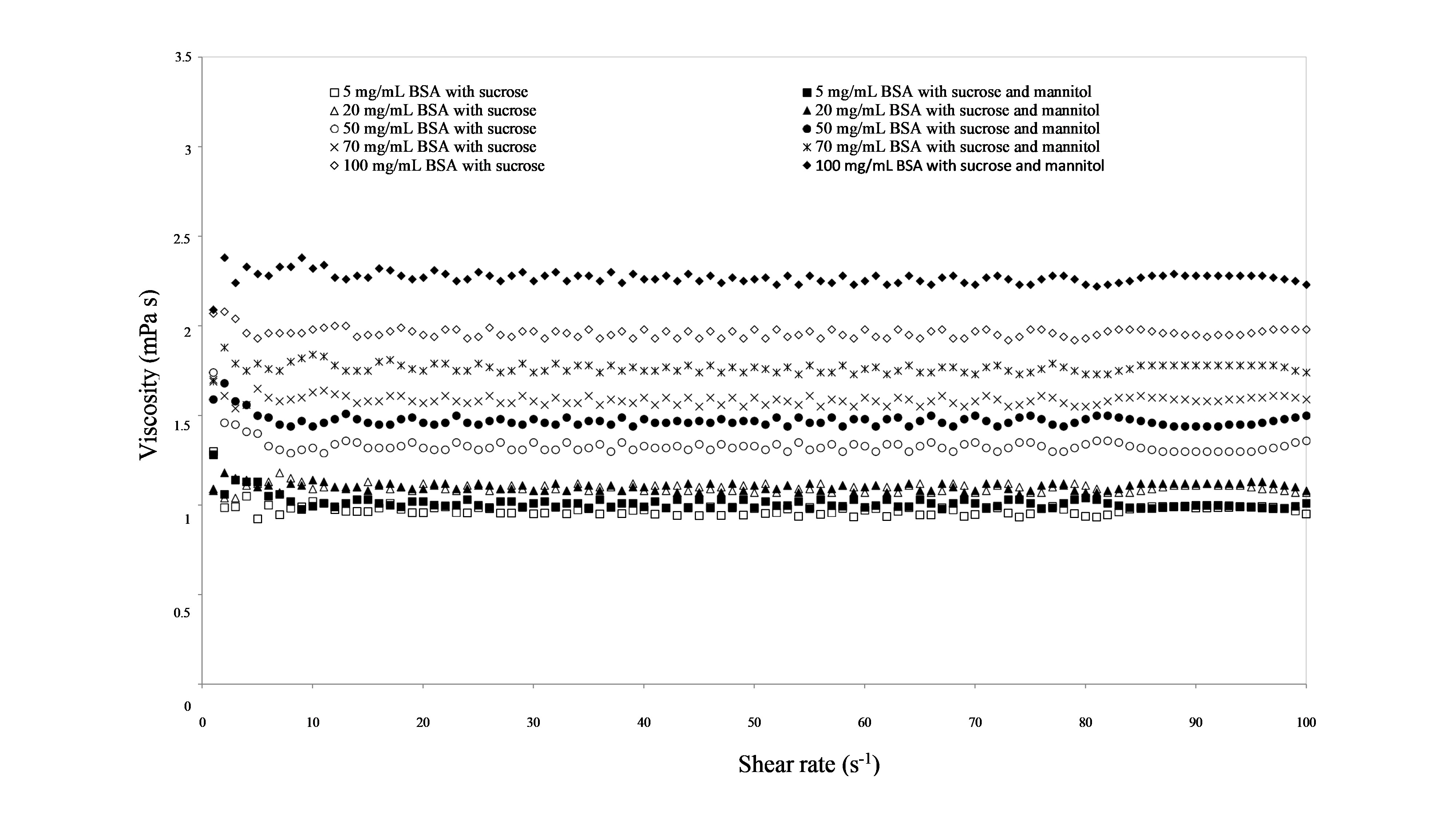
Fig. 2. Viscosity curves of formulations containing sucrose or sucrose and mannitol with different concentrations of BSA.
Considering oscillatory shear frequency sweep measurements (Fig. B, Supplementary File), an observed increase in G′′ with frequency for all tested formulations is the expected attitude for any kind of fluid, either Newtonian or viscoelastic. Irrespective of BSA concentration or mannitol presence, G′ was practically immeasurable, and when, exhibited only a minor magnitude at (some) lower angular frequencies, indicating negligible interactions either attractive or repulsive. Then again, in most cases decreasing attitude of G′ values can be explained by the reduction in intermolecular repulsion that could be responsible for a decrease in bulk ‘‘rigidity’’ and thus G′. Additionally, with the exception of 50 and 70 mg mL–1 BSA formulations, frequency sweeps did not show signs of cross-over between G′ and G′′ at lower frequencies which possibly indicates the fast movement of this protein to the air-water interface (13).
Product appearance and reconstitution time of lyophilised formulations
As reported by Patel et al. it is usually very challenging to evaluate cakes’ appearance in terms of collapse occurrence (17). Cakes can express full collapse, resulting in complete loss of mechanical structure, or it can be just about “cosmetic“ defect. Collapse can be triggered during drying under inadequate drying conditions selected. Due to the addition of mannitol or glycine, drying above Tg' is not detrimental, in case the appropriate ratio between the stabiliser and the bulking agent is selected (18). Reconstitution time is defined as the time required for complete dissolution of dried material (19). By increasing protein concentration, the time for reconstitution drastically increases. In general, increasing protein concentration from 10 to 100 mg mL–1 the reconstitution time increases exponentially from less than 1 min up to 15 min (20). For formulations with monoclonal antibodies in concentrations greater than 100 mg mL–1, reconstitution time can be prolonged up to one hour, thus reconstitution time depends on the type and molecular weight of the protein. The presumption is that amorphous cake dissolves slower than completely crystallized cakes, though the opposite trend has also been observed (21).
Considering Fig. 3a– e, the appearance of cakes differed regarding BSA concentration and the presence of mannitol. In formulation with 5 mg mL–1 of BSA, a shrinkage of the cake was observed, while the addition of mannitol resulted in the collapse. This indicates that a fully crystallised structure led to a loss of mechanical structure. Visual evaluation reveals that the addition of BSA resulted in more rugged cakes with no collapse observed in the absence of mannitol, while at least partial collapse was seen in all formulations with mannitol. This suggests the contribution of the protein itself to the mechanical structure formed during lyophilisation and the unexpectedly detrimental effect of mannitol. It can be concluded that formulations with the lowest BSA concentration and solid content reflect the excessive tendency to crystallization, resulting in a collapse of lyophilisates. In other formulations, the rate of mannitol to sucrose was not high enough to form crystalline scaffolds supporting the amorphous part that provides robust and elegant cake structures. Here again, in addition to DSC results, the preference for sucrose formulations with no mannitol was demonstrated.
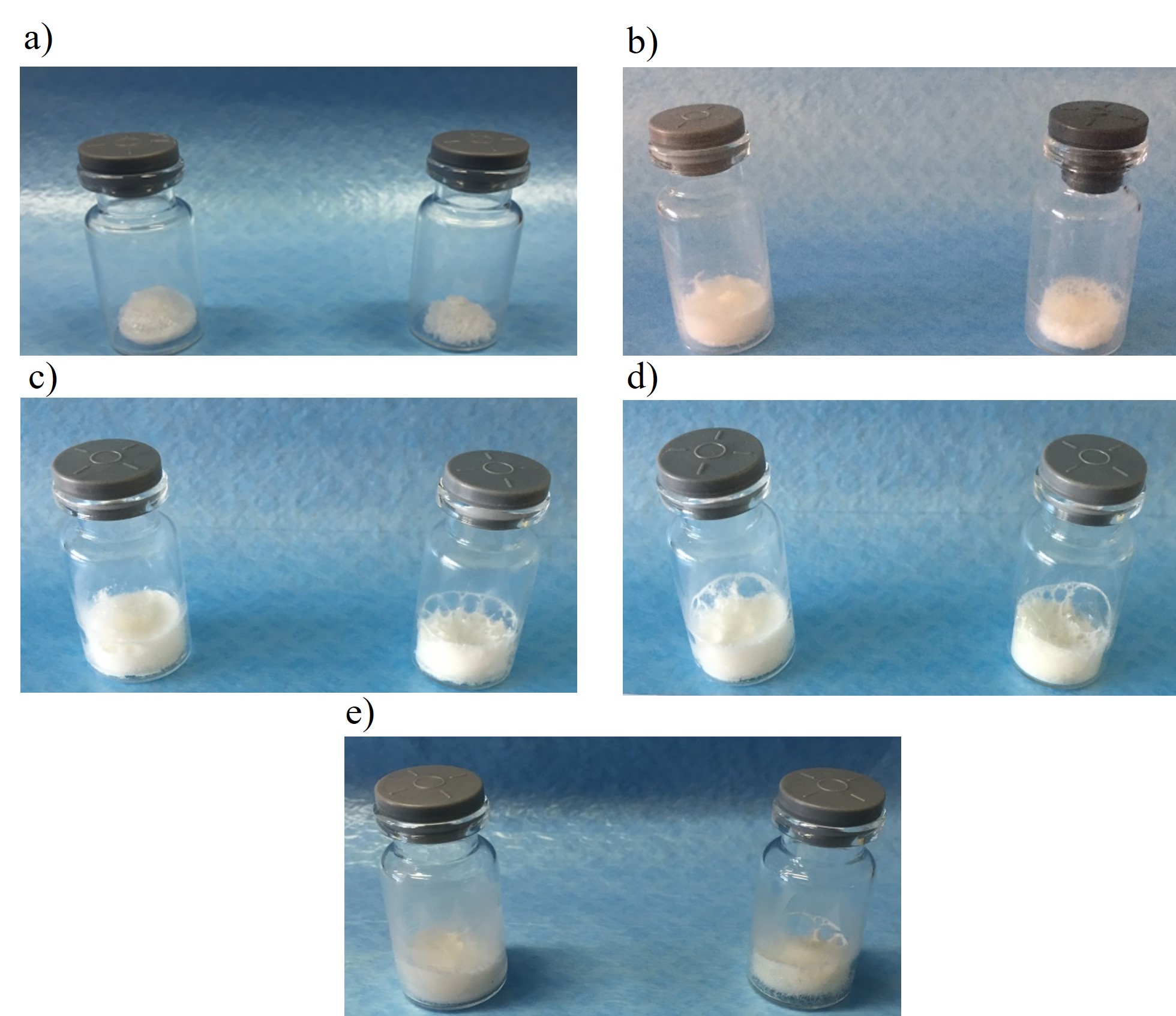
Fig. 3. Visual appearance of lyophilisates containing sucrose (left on the pictures) or sucrose and mannitol (right on the pictures) with different concentrations of BSA: a) 5 mg mL–1 , b) 20 mg mL–1 , c) 50 mg mL–1 , d) 70 mg mL–1 , and e) 100 mg mL–1 .
Table I summarises the observed reconstitution times. As expected, the reconstitution time increased by increasing BSA concentration. In a range from 5 to 50 mg mL–1 BSA, the reconstitution time gradually increased, however at a lower rate than at concentration above 50 mg mL–1 . We can conclude an exponential increase in reconstitution time regarding protein concentration. The obtained values did not reveal a positive effect of mannitol on reconstitution times as they were comparable to formulation with sucrose only. Nevertheless, since BSA is a small protein molecule, all reconstitution times are under one minute, in contrast to monoclonal antibody formulations, where lyophilisate with 100 mg mL–1 protein concentration is reconstituted for up to one hour. At this point, our intention was to show and confirm an ascending trend.
Table I. Reconstitution times of lyophilised formulations with different BSA concentrations
Dynamic light scattering
Aggregation is the most common type of physical instability where aggregates impact product quality and performance and thus represent a potential risk to patient health (22). Therefore, it is necessary to establish permanent control based on highly effective analytical methods over the possible occurrence of aggregates. Dynamic light scattering is typically used to detect very small amounts of protein aggregates and to determine particle size distribution. Since dynamic light scattering is a qualitative method, it should be used in parallel with methods that enable aggregate quantification. The present study was oriented toward monitoring the aggregation as a consequence of lyophilisation process.
To evaluate the effect of the lyophilisation process on the physical stability of BSA, the protein hydrodynamic diameter in pre- and post-lyophilised solutions was compared. With comparable mean particle diameters (Table II, Fig. 4), no visible particles or turbidity of the solutions before and after the drying process, it can be concluded that irrespective of formulation composition lyophilisation did not have a negative impact on protein stability.
Table II. Average particle size in pre-lyophilised solutions and reconstituted lyophilisates
PDI was < 0.25 for all tested formulations.
a The values represent the mean (n = 3) with SD being within 0.2–0.3 for all tested formulations.
This was additionally supported by literature data which state the hydrodynamic diameter of native BSA in a range of ~ 6.5–7.9 nm (23, 24). Our obtained results revealed the BSA hydrodynamic diameters between 6.6 and 8.6 nm, with an average change (pre- vs. post-lyophilisation or vice versa) of 0.7 nm. Complete crystallisation of formulation with 5 mg mL–1 of BSA did not cause aggregation or conformational change in BSA, revealing that despite molecular interactions between BSA and mannitol, interactions between sucrose and BSA were sufficiently intense.
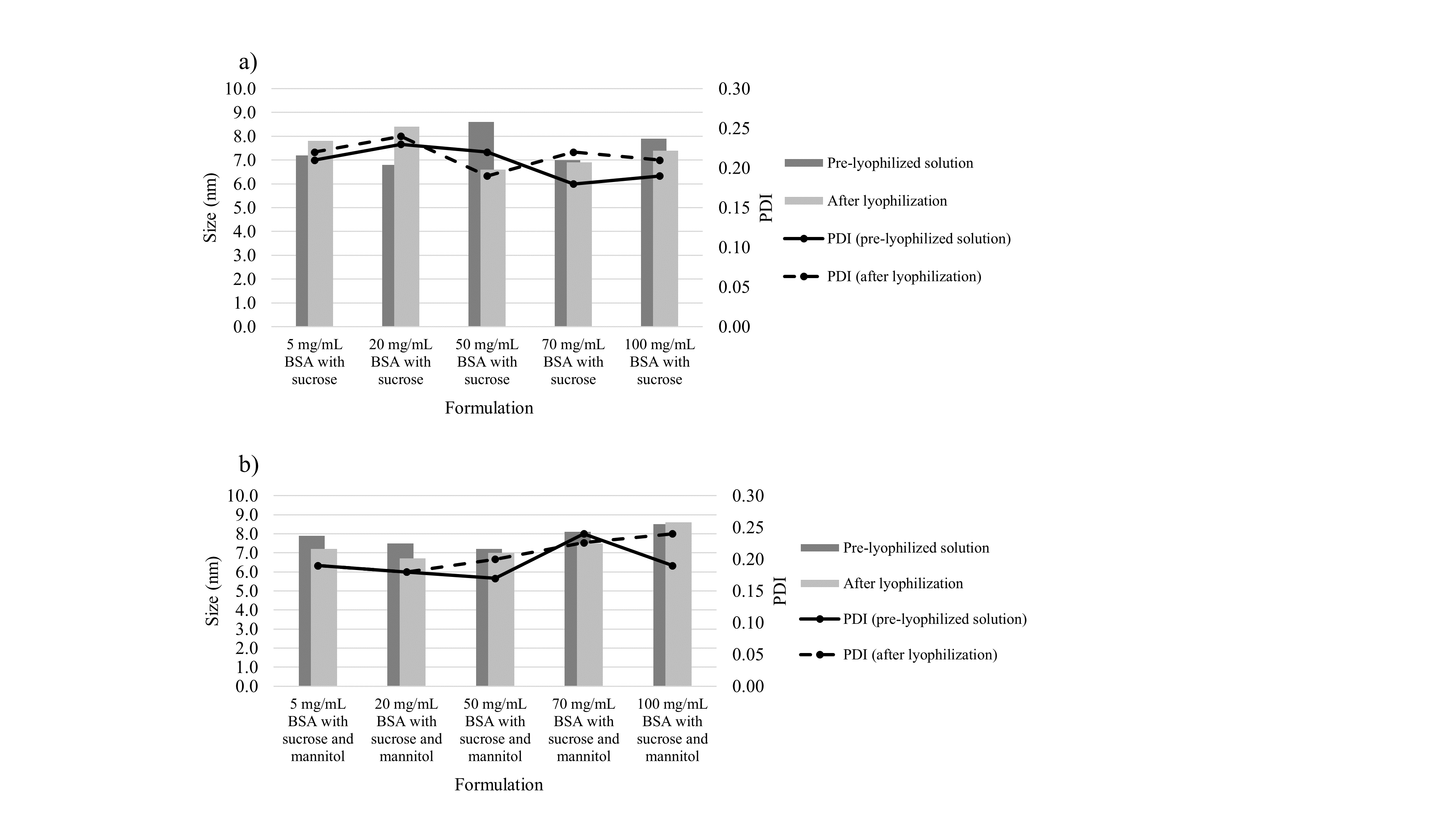
Fig. 4. Average particle size in pre-lyophilised solutions and reconstituted lyophilisates with PDI: a) formulations with sucrose, b) formulations with sucrose and mannitol.
CONCLUSIONS
The importance of gaining new knowledge of highly concentrated protein formulations still persists, especially in light of increasing monoclonal antibodies’ therapeutic formulations, while ensuring opportunities and resources for our descendants, in view of sustainability implementation. Within the present study, it was demonstrated that by increasing BSA concentration the viscosity and reconstitution time increased, representing the major challenge in terms of the development of highly concentrated BSA formulation. Still, protein stability was preserved within lyophilisation due to the addition of sucrose . To obtain a beneficial effect of mannitol it should be included in higher ratios to sucrose, therefore further experiments should be of special concern to critical quality attributes of even more concentrated BSA formulations in correation to excipients used.
Supplementary data. – In the supplementary file, amplitude sweeps for different concentrations of BSA formulations containing sucrose, and storage and loss modulus for different concentrations of BSA formulations containing sucrose or sucrose and mannitol as a function of frequency, are available.
Availability of data and materials. – The datasets generated during and/or analysed during the current study are available from the corresponding author upon reasonable request.
Acknowledgement.– The authors would like to thank Melda Kahraman for her contribution to the laboratory work.
Conflict of interest. – The authors declare no conflict of interest.
Funding. – This work was supported by the Slovenian Research and Innovation Agency (research core funding, No. P1-0189 and grant L1-3160). The Slovenian Research and Innovation Agency had no role in the design and conduct of the study; analysis and interpretation of data; preparation, approval and submission of the manuscript for publication.
Authors contributions.– Conceptualization, M.B.Ž., P.A.G. and M.G.M.; methodology, M.B.Ž. and M.G.M.; investigation, M.G.M. and M.B.Ž.; analysis, M.G.M. and M.B.Ž.; writing, original draft preparation, M.B.Ž.; writing, review and editing, M.G. and M.G.M. All authors have read and agreed to the published version of the manuscript.