Introduction
Cheese has been a staple in the human diet for thousands of years, and it is known for its diverse flavours and textures. The creation of cheese is a complex and multifaceted process, which involves the careful manipulation of milk, the action of various microorganisms, and a controlled ripening parameters. Cheese ripening, also known as cheese maturation, is the period during which the cheese undergoes biochemical and physical changes that transform it from a fresh, mild product into one with complex and often robust flavours and aromas. The development of these sensory attributes during ripening is the result of several interconnected processes. Central to cheese ripening is the activity of microorganisms, including bacteria, yeasts, and moulds. These microbes play an integral role in the transformation of cheese, metabolizing various compounds in the cheese matrix and producing a range of flavour compounds. Lactic acid bacteria (LAB), for instance, are mainly responsible for acidifying the cheese and contributing to its tangy flavour (McSweeney, 2004). Bacteria, yeasts and moulds can produce volatile organic compounds that impart distinctive aromas to different cheese varieties. Enzymes present in the cheese, or introduced by microbial action, catalyse a series of reactions that break down proteins and fats into simpler compounds (Skeie, 2010). In the case of proteins, this process is known as proteolysis, and it generates peptides and amino acids, some of which contribute to the cheese's salty, savoury, astringent, bitter and umami flavours (Ozturk et al., 2013). Lipolysis, the breakdown of fats, yields free fatty acids that can add creaminess and richness to the cheese (McSweeney, 2007). Furthermore, environmental conditions, such as temperature, humidity, air flow and oxygen levels play a vital role in shaping the ripening process. These conditions vary widely depending on the cheese type. Adjusting the ripening temperature regime can optimize the course of propionic fermentation and the formation of cheese eyes in Emmental cheese (Bachmann, 2011). Furthermore, the higher the temperature and humidity in the ripening rooms, the greater the growth and development of yeasts (Leclercq-Perlat et al., 2015), which is undesirable in the ripening of hard and semi-hard cheeses such as Emmentaler, Gouda and Tilzit, and desirable in the maturation of smear-ripened cheese, like Limburger or Romadur. All of the above have an influence on cheese quality, and one of the most crucial aspects of its quality is aroma. The diverse range of aromas in cheese is a result of the complex interactions between volatile compounds produced during ripening. The presence of curd’s breakdown products, such as, free amino acids, fatty acids, amines, ketones, lactones, ethanol, etc., is directly related to the cheese making process and the conditions during ripening (Zheng et al., 2021). Some of these compounds are responsible for fruity, nutty, buttery, or earthy aromas, while others contribute to the characteristic pungency and tang found in aged cheeses (Gao et al., 2022). Understanding the development of these aromatic compounds during ripening is of utmost importance to cheese producers and researchers, as it allows for the intentional manipulation of flavour and aroma profiles to meet consumer preferences.
In recent years, the food industry has shown increasing interest in high-pressure processing as a non-thermal method to enhance food safety, extend shelf life, and influence the texture and flavour of various products. The mentioned treatment involves subjecting food products, in this case cheese, to pressures typically ranging from 100 to 600 megapascals (MPa), which is substantially higher than atmospheric pressure. This process can be applied at different stages of cheese production, including the ripening phase. It has been proven that high pressure is effective in inactivating harmful microorganisms such as Listeria monocytogenes, E. coli, Pseudomonas flourescens and other gram-negative microorganisms (Gervilla et al., 2000). This significantly enhances the safety of cheese, particularly for soft and semi-soft varieties. High-pressure processing can also extend the shelf life of cheese by inhibiting the growth of spoilage microorganisms, which can be particularly beneficial for cheese varieties that mature over a long period. Furthermore, some studies have shown that high pressure can enhance the release of volatile compounds, leading to intensified aroma development, and can improve the functional and rheological properties of certain cheese types (O'Reilly et al., 2003; Ozturk et al., 2013). On the other hand, disadvantages of using high-pressure processing can be seen in the following: expensive equipment to acquire and maintain (financial challenges for smaller cheese producers), loss of certain beneficial microflora (e.g. high pressure can deactivate LAB bacteria), and limited application (more suitable for certain cheese types, particularly those with higher moisture content).
While the high-pressure processing is extensively used in the cheese production, including non-thermal pasteurization of milk, during curd formation, and after manufacture for a short period of time and even after the maturation period, as evidenced by Nunez et al. (2020), but it has never been applied in a specially designed ripening chamber during cheese maturation, which is the topic and focus of this scientific paper. Therefore, the objectives of this research were: (a) to determine the optimal conditions for the cheese ripening in an overpressure chamber according to optimal process parameters of cheese maturation verified through GC/MS analysis; (b) to provide microbiological analyses production with the implementation of microbiological controls of yeasts, moulds, Listeria monocitogenes and Salmonella spp.
Materials and methods
Milk collection
Raw whole cow’s milk was obtained from a local dairy farmer in Karlovac (Croatia) in the year 2023, kept overnight and transferred cold (+4 °C) in PVC buckets to the dairy practicum of Karlovac University of Applied Sciences where the cheese production and ripening were carried out.
Cheese production
After receiving the milk, the production of semi-hard cheese began with a Low Temperature Long Time (LTLT) vat pasteurization, where the milk was heated for 25-30 minutes at 63-65 °C. Cheese was produced in a 30 L volume double-stack duplicator with tap water as the heating and cooling medium. After that, 0,00182 % (w/v) (in grams: 0,546) of lyophilized dairy culture (MYStarter CT 330 Series; Streptococcus salivarius ssp. Thermophilus; producer: Maysa Gida, Turkey) and 0,00777 % (w/v) (in grams: 2.33) of microbial rennet (MUCOREN 2000; Rhizomucor miehei; producer: Maysa Gida, Turkey) were added to the pasteurised milk. The cheese curd was cut, drained, moulded and pressed under a pressure of 1-1.5 bar. The final stage of cheese production was brining in a salt concentration of 13% and pH levels 4.5-4.7 for 24 hours.
Cheese ripening
Ripening of cheese was carried out in two specially designed ripening chambers (Fasek d.o.o., Zagreb, Croatia), a control chamber and an overpressure chamber (Figure 1), with temperature, humidity, and airflow control option on a customizable local touch screen display.
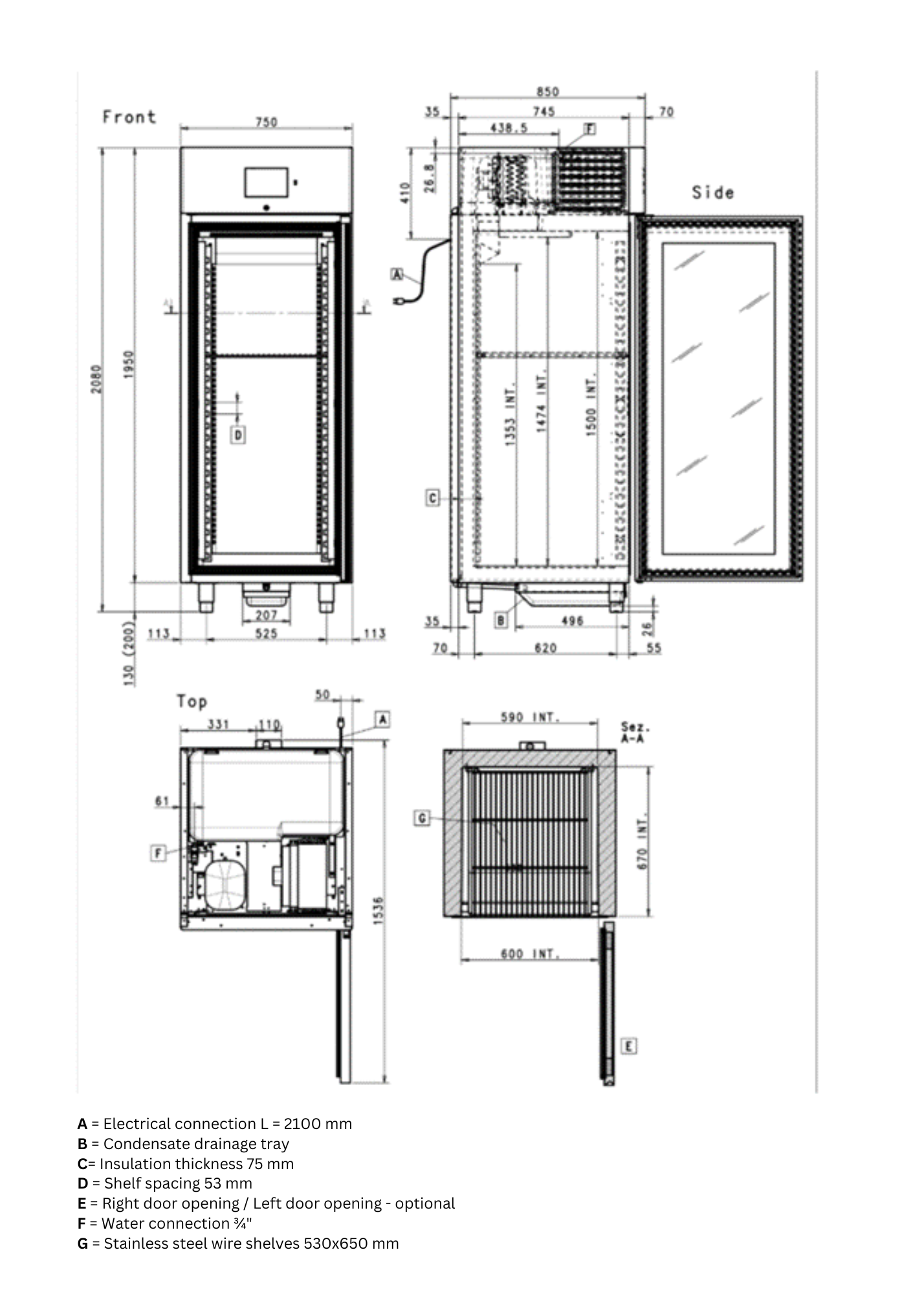
Figure 1. Process scheme of ripening chambers
The monitoring and regulation of temperature (+0 °C to +30 °C), humidity (40 % to 95 %), airflow velocity (1xØ200 mm - 10W), overpressure, CO2 and O2 concentration was also done through the SCADA system that includes trend logging, graphing and statistical analysis, alarm notifications and email alerts, automated reporting (PDF, CSV), and remote access and control. The ripening chambers are equipped with an audio-visual alarm for set parameters, with adjustable alarm limits for all measured variables. The interior chamber volume for ripening is 530x650x1500 mm (WxDxH), with 5 mobile polypropylene shelves, and a product capacity ranging from 100 to 150 kg.
Control (chamber 1) and overpressure chamber (chamber 2) have the same technical specifications with the exception of additional equipment for overpressure control, ranging from 0.1 to 5.0 mbar) in chamber 2. Cheese samples were held under different pressure treatments (atmospheric pressure of 1.01325 bar in chamber 1, and 1.01443-1.01656 bar in chamber 2) for 3.59 weeks to 6.41 weeks (according to RSM and CCRD design explained in detail in section Experimental design and statistical analysis), as shown in Table 1, with temperatures set lower at 10 °C at the beginning of ripening, increasing to a higher 16 °C in the middle of the process, and then decreasing back to lower levels towards the end, along with relative humidity starting at 75 %, peaking at 85 % mid-ripening, and then dropping back towards 75 % by the end of the ripening period. It is important to note that no care of the cheese was carried out during its ripening, which would include its brushing, washing, coating with various protective agents, etc. The only thing that was carried out was turning the cheeses daily at the beginning of the ripening stage.
Table 1. The uncoded and coded levels of independent variables used in the RSM design
Microbiological analyses
Raw milk, pasteurized milk, whey and cheese were analysed for microbiological quality. Before the start of cheese production, 1 L of raw milk was excluded, and during production, 1 L of pasteurized milk and 1 L of whey. Different batches of cheese during ripening were also analysed after week 1, 2, 3, 4, 5 and 6, both in chamber 1 and 2.
Conducting microbiological analyses on cheese samples
Determining yeasts and moulds according to HRN ISO 21527-1:2012 (Horizontal method for the enumeration of yeasts and moulds - Part 1: Colony count technique in products with water activity greater than 0.95) and HRN ISO 21527-2:2012 (Horizontal method for the enumeration of yeasts and moulds - Part 2: Colony count technique in products with water activity less than or equal to 0.95). The methods include sample preparation, inoculation, and incubation of the test sample. Determining the presence of Salmonella spp. bacteria using the method HR EN ISO 6579-1:2017 (Horizontal method for the detection, enumeration, and serotyping of Salmonella). Determining the presence of Listeria monocytogenes bacteria using the method HR EN ISO 11290-1:2017 (Horizontal method for the detection and enumeration of Listeria monocytogenes and other Listeria spp.)
Experimental design and statistical analysis
Response surface methodology (RSM) and central composite rotatable design (CCRD) was used for determining optimal conditions (Bas & Boyaci, 2007) for cheese ripening using overpressure ripening chamber. The considered parameters during cheese ripening optimization were as follows: overpressure (1.18-3.31 mbar), and time (3.59-6.41 weeks) (Table 1). Experimental data were fitted with second order response surface model with the following form:
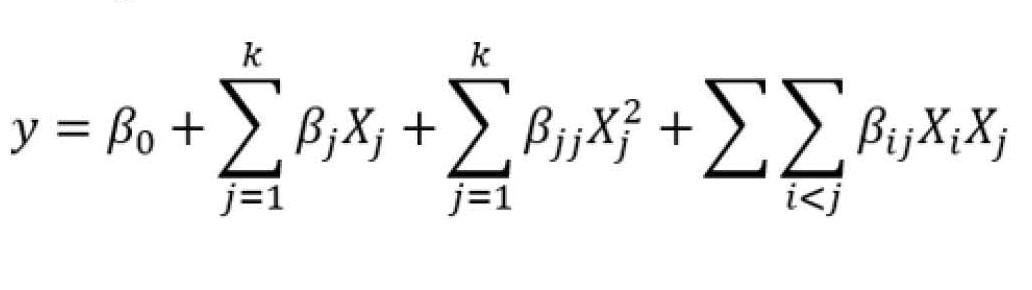
(1)
where y is response (the main detected volatile compounds, respectively), β0, βj, βjj, βij are constant coefficients of intercept, linear, quadratic, and interaction terms, respectively; Xi and Xj are coded independent variables [overpressure (X1), and time (X2)]. Ripening temperature and relative humidity were not included in the optimization process because they were constant for all types of cheese in both chambers. The response values were mean of the replicate measurement. Analysis was performed using commercial software Design-Expert® (ver. 12, Stat-Ease Inc., USA). The overall predictive capability of the model is commonly explained by the coefficient of determination (R2). The analysis of variances (ANOVA) was also used to evaluate the quality of the fitted model. The test of statistical difference was based on the total error criteria with a confidence level of 95.0 %. The lack-of-fit is significant at p<0.05 showing the adequacy of the quadratic model selected.
GC-MS analysis of volatile components
Gas chromatography and mass spectrometry (GC-MS) analyses were carried out on an Agilent Technologies (Palo Alto, CA, USA) gas chromatograph model 7890B with 5977A mass detector. Operating conditions were: column HP-5MS (5 %-phenyl-methyl polysiloxane, 30 m x 0.25 mm i.d., coating thickness 0.25 µm); Helium as carrier gas: 1 mL min-1; injector temperature: 250 °C; HP-5MS column temperature programmed at 70 °C isothermal for 2 min, and then increased to 200 °C at a rate of 3 °C min-1 and held isothermal for 18 min; 1:50 the split ratio; ionization voltage: 70 eV; ion source temperature: 230 °C; mass scan range: 45-450 mass units. The sample was prepared and analyzed using the HS-SPME procedure (solid phase microextraction) with PAL3 RSI 120 autosampler with SPME fiber DVB/CAR/PDMS (divinyl-benzene/carboxen/polydimethylsiloxane) which was conditioned according to Supelco Co. instructions before extraction. The cheeses (1 g) were placed separately in a glass vial (20 mL) that was hermetically sealed using PTFE/silicone stoppers. The vials were preheted on 60 °C during 5 min and then extraction by HS-SPME was performed (40 min). After the extraction, thermal desorption (7 min) was performed on the GC-MS injector. Injection of SPME fiber in GS-MS inlet indicates start of analysis.
Results and discussion
The high-pressure processing is extensively used in the cheese production, but it has never been applied in specially designed ripening chamber during cheese maturation like in this study. So, it was interesting to compare data obtained with classic cheese ripening with cheese ripening in overpressure chamber in terms of volatile compounds and microbiological analysis.
Furthermore, to optimize the cheese ripening process in a newly designed overpressure chamber, Response surface methodology (RSM) was used. The RSM method was first presented by Box and Wilson (1951) and since then RSM and mathematical modelling have become indispensable tools in optimizing processes. RSM can be defined as a set of statistical and mathematical methods used to model polynomial models and initial data that must display the behaviour of a dataset for the purpose of making statistical predictions. This model is useful in optimizing, designing, developing and improving processes where responses are influenced by multiple variables. Primarily, with the RSM technique not only optimized process parameters were got, but also data on the probability of obtaining a solution and on the sensitivity of the system to changes in given conditions within the experimental range. A great advantage of this method is the interpretation of the results through graphic illustrations (3D graphs), which certainly facilitates the visual experience of the influence of independent variables. In this study, the effect of different independent values of overpressure and cheese ripening time on the volatile compounds (responses) were investigated.
In Table 2 is the screening of the obtained volatile compounds of cheese obtained in overpressure chamber compared to control sample. Ripening chambers have a precise regulation of pressure, humidity, temperature, and time, so all repeated experiments are at exact decided process parameters.
According to that data it can be seen influence of selected overpressure process variables on the most abundant compounds detected using GC/MS (Table 3).
Table 2. Screening of the obtained volatile compounds of cheese obtained in overpressure chamber compared to control sample (RI from the literature used for identifying the compounds)
- not detected
Table 3. The experimental design and data for the response surface analysis (in %-percentage)
From the data obtained in Table 3 it can be seen that the concentration of hexanoic acid is relatively low compared to other developed compounds ranging from 1.56 to 11.21 %. Hexanoic acid, also known as caproic acid, is a saturated fatty acid naturally found in various foods and is known for its pungent, acidy and goat-like smell. In moderate amounts, it can contribute to the aroma profile of some cheese varieties giving it a sweaty, cheesy aroma (Fan and Qian, 2006). Octanoic (caprylic) acid can also contribute to the aroma of some cheese varieties. This saturated fatty acid has a slightly fruity and sweet but also rancid, musty, wax-like and goat-like notes. It is most commonly found in coconut oil and certain dairy products (Altinoz et al., 2020). The highest concentrations of caprylic acid are recorded by cheeses in experiment at run 9 and run 13, which ripened under 2.25 and 3 mbar for 3.59 and 4 weeks, respectively. The concentrations of octanoic acid vary widely across the samples, ranging from as low as 3.80 to as high as 25.99 %. In addition to giving cheese aroma, caprylic acid is also recognized and used as an antimicrobial agent (Nair et al., 2005). Another saturated fatty acid with slightly rancid odour is n-decanoic (capric) acid. Capric acid is typically not a desirable component of cheese aroma. Its sour, rancid, and animal-flavour attributes are generally considered unpleasant and should be minimized during cheese production (Güler, 2005). The lowest concentrations were observed under the lowest overpressure treatment 1.19 and 1.50 mbar. Caproic, caprylic, and capric acid have been identified in different cheese types such as aged Cheddar, Grana Padano, and predominantly in goat cheese, and can be desirable when it imparts a characteristic "goaty" or tangy aroma (Tian et al., 2020). The highest concentration of the mentioned compounds was proven under the lowest cheese ripening time and overpressure conditions of 2.25 mbar. However, excessive levels can lead to an unpleasant odour, so its presence should be carefully controlled. During cheese production, especially in the early stages of fermentation, lactic acid bacteria (LAB) and other microorganisms present in the milk break down triglycerides as a result of their metabolic activity, various fatty acids are released, including hexanoic acid, octanoic acid and decanoic acid. Studies show that the presence of 3,5 dihydroxydecanoic acid 1,5 lactone can be influenced by the metabolic activity of various types of fungus (Laili et al., 2017; Mentle, 1987; Vesonder et al., 1972). This cyclic ester was found to be the most abundant aroma component found in cheese samples. Lactones are known for imparting a rich and fatty character to the aroma profile of cheeses which would make them a desirable compound in cheese, as it can contribute to a creamy and slightly sweet aroma. Furthermore, dodecanoic acid, or lauric acid, is a saturated fatty acid with a twelve-carbon chain. It has soapy and waxy notes that can lead to off-flavours, and is not typically a desirable aroma compound in cheese and should be kept at low levels (Güler, 2005). Consequently, lower concentrations of lauric acid (1.27-13.30 %) were found in cheese samples compared to other aroma components. Delta-dodecalactone can be a desirable component in cheese aroma, as it imparts sweet and creamy notes. It is often associated with a pleasant buttery, caramel-like aroma, and its presence can enhance the sensory experience of certain cheese varieties. Higher concentrations were found at higher overpressure treatments (2.25-3.31 mbar). Cheese samples with the same overpressure and time may have significantly different aroma components concentrations. It seems that neither overpressure (measured in mbar) nor time duration (in weeks) alone can fully explain the variation in aroma components concentration.
The effect of the linear, quadratic or interaction coefficients on the response was tested for significance by analysis of variance (ANOVA). Regression coefficients of intercept, linear, quadratic, and interaction terms of the model were calculated using least square method. The degree of significance of each factor is represented by its p-value. Table 4 shows the corresponding p-values for selected response variables for each obtained coefficients and interactions. From Table 4 it can be noticed that the overpressure exhibited the most statistically significant influence (p<0.05) on all six investigated responses. However, time exhibited statistically significant influence only on the amount of octanoic acid and n-decanoic acid. The interaction between overpressure and time show significant influence only on the amount of octanoic acid and n-decanoic acid.
Table 4. Regression coefficient of polynomial function of all response surfaces
X1 - overpressure; X2 - time; * p<0.01 highly significant; 0.01≤p< 0.05 significant; p≥0.05 not significant.
Table 5. Analysis of variance (ANOVA) of the modelled responses
Analysis of variance (Table 5) shows that the regression models for all investigated responses were statistically relevant with a significance level ranging from p<= 0.0031 to p=0.0275, and the models had no significant lack of fit (p>0.05). The fitted model represents the experimental data well with high correlation coefficients, R2, varying from 0.7843 to 0.8892, depending on investigated responses.
The three-dimensional plots used to express the investigated responses (y) as a function of independent variables (in terms of coded values) are shown in Figure 2. According to the Figure 2 it can be seen that by increasing of overpressure the amount of all six responses (hexanoic acid, 3,5 dihydroxydecanoic acid 1,5 lactone, dodecanoic acid, delta-dodecalactoneoctanoic acid, and n-decanoic acid) increase significantly. Time od cheese ripening shows different influence on observed responses. In general, the trend of aroma components formation was increasing with ripening time. The concentration of hexanoic acid and octanoic acid records a slight decrease in values after the 4th week of ripening, which increases as time progresses. While the values of dodecanoic acid, delta-dodecalactoneoctanoic acid, and n-decanoic acid show a positive trend. The concentration of the mentioned aroma components increases during ripening, up to about 5.5 weeks of ripening, and towards the sixth week, the concentration decreases slightly.
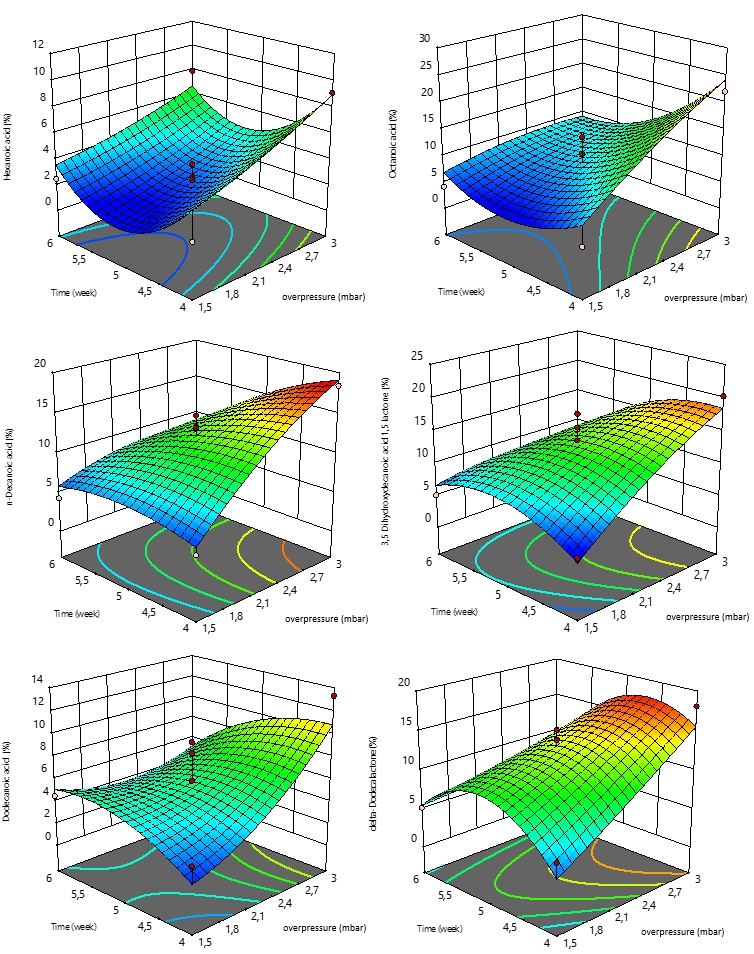
Figure 2. Three-dimensional plots for obtained responses as a function of time of cheese ripening and overpressure
Microbiological analyses of cheese samples showed the absence of pathogenic and unwanted microorganisms, the total number of microorganisms was within the limits. By reviewing Table 6 and Table 7, it can be observed that the number of yeasts and moulds in the control and overpressure chamber was mostly maintained at a low level (<2.00 log CFU/g) throughout all ripening times. This suggests that the cheese ripening process under controlled conditions successfully controlled yeast growth. On the other hand, mould levels have shown different trends. Overpressure levels of 2.25 and 3 mbar increased mould numbers over ripening time from weeks 1-3. After week 3, the number of moulds in the cheese sample began to decrease until the end of the ripening period suggesting that the initial growth phase of moulds was followed by a decline, possibly due to the prolonged period of pressure treatment, or factors such as competition for nutrients or changes in environmental conditions (pH changes, moisture content, oxygen levels, etc.) that were altered by the pressure treatment. It is evident that the difference in the level of pressure used affected the growth dynamics of yeasts and moulds.
Table 6. Microbiological quality of cheese samples obtained in control chamber compared to the cheese samples obtained in an overpressure chamber with a 2.25 mbar
Table 7. Microbiological quality of cheese samples obtained in control chamber compared to the cheese samples obtained in an overpressure chamber with a 3 mbar
Conclusion
As known, free fatty acids are important, or even predominant, components of cheese flavour. The concentration of aroma compounds in cheese during ripening is the result of a complex interplay between pressure and time, as well as other cheese-specific ripening parameters. While higher pressures may lead to higher concentrations of certain compounds, and longer ripening times can influence the concentration of some aroma compounds, the relationship is not consistent for all compounds. The optimal conditions for the cheese ripening in an overpressure chamber according to GC/MS analysis, and best cheese microbiological quality, would include shorter cheese ripening time and overpressure conditions of 3.00 mbar. However, the data reveals that the concentration of aroma compounds can vary significantly from one cheese sample to another, even under the exact same pressure and time conditions. This suggests that cheese ripening is influenced by more factors, including the specific microbial and enzymatic activities involved in the ripening process, complex biochemical processes, cheese production process, cheese care, position in the ripening chamber, and more. Based on the analysed data, it can be concluded that the cheese ripening process significantly impacts the presence of yeast and mould in cheese samples. Controlled ripening conditions appear effective in suppressing yeast growth, while variations in mould presence depend on different ripening conditions and pressure levels. Further studies may be needed to better understand the mechanisms behind these changes and to optimize cheese ripening conditions for microbiological quality control.