INTRODUCTION
Historically, the majority of oral dosage forms administered to children were off-label or unlicensed. To ensure accurate dosing for neonates and children, healthcare professionals often resorted to modifying adult dosage forms, such as crushing tablets and mixing them with liquid, breaking tablets into smaller portions, or opening capsules and dividing the enclosed powder for administration. (1). Since the beginning of this millennium, several tools for personalised medicine have been introduced in paediatrics, such as mini-tablets or 3D printing of drugs (1, 2), but pharmaceutical compounding (dose adaptation done by pharmacists) and manipulation (dose adjustment at the point of administration by nurses or parents) are still the most commonly used alternatives. Several studies have highlighted the difficulty of obtaining an accurate dose from manipulated tablets, with large dose variations and discrepancies between manipulations due to galenics, prescribed dose, or manipulator experience (3–5). Therefore, these practises cannot be considered equivalent to commercial forms manufactured according to good manufacturing practises (6, 7). The consequences of using compounding/manipulation of conventional drugs approved for adults can translate into suboptimal adherence due to bad taste, underdosing with therapeutic failure, or overdosing with adverse effects (8–10).
In the last two decades, the field of paediatric pharmacy has been advanced by the development of improved paediatric formulations. The main focus has been on the development of age-appropriate and child-friendly medicines, with the introduction of a regulatory framework as an incentive. Since 2007, the European pharmaceutical industry has been prohibited from developing new medicines intended only for use in adults. Paediatric Regulation requires that children be included in a form of paediatric development called Paediatric Investigation Plan (PIP), which requires the agreement of the European Medicines Agency (EMA) and its Paediatric Committee (11). In 2007, the World Health Organisation (WHO) also launched its “Make Medicines Child Size” initiative (12). Since 2009, all applications with a new aspect (indication, route of administration, dosage form) must include information and study results that comply with PIP (2). In 2014, the EMA introduced guidelines for the pharmaceutical development of medicinal products for paediatric use (for children younger than 18 years of age) (13). In the United States, the Food and Drug Administration (FDA) enacted the Best Pharmaceuticals for Children Act in 2002 to provide financial incentives for voluntary paediatric studies conducted by companies. In 2003, the Paediatric Research Equity Act required companies to evaluate drug safety and effectiveness of medicines in paediatric patients (14). These two acts were reauthorized in 2007 as part of the FDA Amendment Act. In the United States, the document corresponding to PIP is called the Paediatric Study Plan. The outcomes arising from of all the mentioned paediatric regulation were examined by the EMA, more specifically the Paediatric Committee, as documented in their 2017 10-year report submitted to the European Commission (E.C., 2017). Three main objectives were defined in this report: to promote research and development of pharmaceutical formulations for children; to ensure that paediatric pharmaceutical formulations are designed and approved for their use in the near future; to increase the availability of information on this topic by encouraging stakeholders cooperate with each other and share new information (15). There are already some excellent reviews that address the progress and challenges of paediatric formulation development (7, 16–18).
The term “paediatric” encloses several age groups, including neonates (0–27 days), infants (28 days–23 months), children (2–11 years), and adolescents (12–17 years), leading to differences based on their physiologic development that may be critical to the development of dosage forms (19).
Liquid formulations have traditionally been considered the most optimal medications for children because they are easy to swallow and their doses can be easily adjusted. However, some of their major drawbacks include poor stability, low potential for taste masking and controlled release, and a greater number of excipients. In 2008, WHO and the EMA, in collaboration with experts in the field, stated that flexible solid oral dosage forms are probably best suited for children. These are defined as those that can be administered in more than one way, such as dispersed in water or breast milk or taken orally as a whole (7). Multiparticulate formulations, or in short multiparticulates, definitely meet this requirement well.
Multiparticulates are small solid multiple-unit dosage forms including microparticles, nanoparticles, granules, pellets, beads, and mini-tablets, usually less than 4 mm in diameter, where a dose consists of multiple particles. Depending on formulation characteristics, multiparticulates can be mixed with beverages or soft foods, or they can even be placed directly in the oral cavity. They are not intended to be dissolved in liquid for administration, because this may affect palatability as well as influence pharmacokinetic profile (7). A study assessing the acceptability of placebo multiparticulates of different sizes in healthy children and adults concluded that multiparticulates could serve as a viable means for administering drugs to children aged four years and older (20). It was also found that multiparticulates were the most common solid oral formulation among paediatric oral formulations during 2007–2018, confirming their suitability for children (21). Because dose adjustment is straightforward with such formulations, especially with the novel dispensing systems (26–31), the main focus is on taste masking of poor-tasting active pharmaceutical ingredients (APIs), which can be challenging when dealing with small particles (22–24). The unpleasant, particularly bitter taste of an API has been cited as a key obstacle in paediatric drug formulation development, as children are known to be more sensitive to bitter and unfamiliar-tasting substances than adults (16, 25). Therefore, it is crucial to develop approaches to mask the taste of APIs in order to comply with the children's needs.
The aim of this review is firstly to provide a general overview of taste masking methods of multiparticulates and a critical review of taste masking evaluation methods. Second, the focus will be switched specifically on multiparticulates containing poorly water-soluble APIs where taste masking is implemented. Their formulation, excipients used, effectiveness of taste masking, and potential improvements of dissolution profile will be discussed in detail. It is the authors' belief that this is a particularly interesting research niche because the aim of most taste-masking approaches is to slow the dissolution of the drug, which is exactly the opposite of what we typically want to achieve with poorly water-soluble APIs, especially when rapid release is desired. Therefore, achieving taste masking and sufficient, or even enhanced, dissolution of a poorly water-soluble API in one formulation is particularly challenging.
TASTE MASKING METHODS
Many methods have been developed to mask the taste of bitter-tasting APIs in multiparticulates. The topic has been discussed in several excellent reviews in recent years (32–35). Briefly, three categories of taste masking approaches can be distinguished, which aim to mask the taste at three different levels: the formulation level, the particle level, and the chemical level (35, 36).
At the formulation level, the use of sweeteners, flavors, bitter blockers, and taste modifiers is most common. Both sweeteners and flavors are very intuitive and simple methods that can mask any taste, not only bitter ones, but their success is limited for highly bitter or very water-soluble APIs as well as with APIs with severe and persistent aftertastes (32, 37). Bitter blockers and taste suppressants act by interfering with taste receptors or taste transduction mechanisms (38). Because there are approximately 25 known bitter receptors, bitter blockers often have the disadvantage of acting on only a specific receptor or a few receptors, depending on their structure. On the other hand, if they act on one of the later stages of taste transduction, they may also block other tastes, making such compounds less commercially desirable (32, 38).
At the particle level, the main objective is usually to reduce or prevent the contact of a bitter-tasting API with taste receptors in the mouth by a coating process, as shown in Fig. 1.
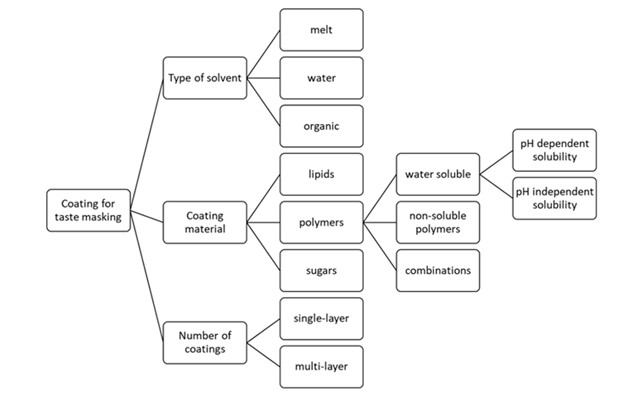
Fig. 1. Coating process for taste masking; adapted from Worku et al. (46)
The most common approaches are microencapsulation, polymer film coating, and lipid barrier systems (35). Polymer coating has been reported as the most viable option for taste masking (33, 37). Different types of polymers can be used, either water-soluble, water-insoluble, or with pH-dependent solubility, as shown in Table I (39). The latter may be the most promising because they do not dissolve at salivary pH, whereas they are highly soluble at gastric pH and release the drug rapidly. Eudragit E PO is a cationic copolymer commonly used for taste masking and seems to provide the most promising results (22, 40–42). Lipid barriers could be an interesting alternative because they are easy to use (melting) and allow for an anhydrous process and formulation, which is suitable for moisture-sensitive APIs. In addition, taste masking with lipids can work in different ways, i.e., the API can be dispersed into the lipid structures, bind directly to the lipid molecules, or adsorb on the insoluble surface of the lipid particles (32, 43). However, impurities can affect the melting interval and thus the whole process, which is a drawback of this method. Scale-up can also be very challenging due to difficulties in temperature regulation (44). Microencapsulation can be considered a modern extension of film coating, intended for small particles and therefore very suitable for multi-unit dosage forms A good example of this is the Actimask technology developed by SPI Pharma. Particles of ibuprofen and acetaminophen are coated with gelatin by coacervation from water, which should provide a smooth mouthfeel in addition to taste masking in the first 2 minutes (45).
Table I. Examples of polymers used for taste masking (34, 47)
The chemical level includes a variety of methods, most of which work by reducing the solubility of API in saliva, reducing the number of API particles exposed to taste buds, or reducing its affinity for receptors. This is achieved by chemical modification of API into a prodrug or into a salt, by conversion into another (less soluble) solid form (polymorph, cocrystal), or by conversion into an inclusion complex with ion-exchange resins or cyclodextrins (32, 33). Complexation with cyclodextrins is considered the most challenging platform for taste masking and also requires large amounts of cyclodextrins, so it is not considered very promising. In addition, there is limited data on the potential toxicity of cyclodextrins in children younger than 2 years of age (36, 48, 49).
Recently, there have been some publications on the use of nanocarrier systems and procedures like nanoencapsulation for taste masking. Various additional advantages have been attributed to these, depending on the type of nanocarrier system (improved stability for submicron lipid emulsions, specific targeting for nanogels, high loading capacity for nanostructured lipid carriers). However, the procedures to prepare such systems are usually lengthy, costly, and complicated, so research is still limited (50). In addition, it has been reported that very small particles may remain trapped on the tongue instead of being swallowed, which is not desirable (51, 52).
TASTE MASKING OF MULTIPARTICULATES BY FILM COATING
As mentioned above, polymer film coating has been reported as the most appropriate approach for taste masking, and several studies using this concept have been published to date (Table II).
Polymers used for taste masking are based on polysaccharide, polypeptide, or vinyl polymer chemistry. They can be water-soluble, cationic, anionic, or neutral insoluble polymers (34). They must be non-toxic and compatible with the API and other formulation ingredients. It is important to precisely know their solubility in water and organic solvents, pH-dependent solubility, and their glass transition temperature, as these properties affect the film coating process, the necessity to add other ingredients to the coating solution, the effectiveness of film coating and its stability (33, 34).
Kollicoat® Smartseal 30 D and 100 P are both copolymers consisting of methyl methacrylate (MMA) and diethylaminoethyl methacrylate (DEAEMA) in a 7:3 ratio. The difference between them is that Kollicoat Smartseal 30 D is an aqueous dispersion, while 100 P is a spray-dried powder. Both also contain macrogol cetostearyl ether (0.6 and 2 % in 30 D and 100 P, respectively) and sodium lauryl sulfate (0.8 and 2.5 % in 30 D and 100 P, respectively) for stabilization (79, 80). Both are soluble below a pH of 5.5 and insoluble at neutral pH, making them enterosoluble polymers.
Eudragit® E 100 and E PO are cationic copolymers based on dimethylaminoethyl methacrylate, butyl methacrylate, and methyl methacrylate in a ratio of 2:1:1. The difference between them is that Eudragit E 100 is a granulate, while E PO is a powder. Both are soluble below pH 5.0 (81). T The manufacturer also states in its guidelines that E 100 should be used with organic solvents as coating liquid, while E PO should be used with water (49).
Eudragit L 30 D-55 is another polymer with pH-dependent solubility, but unlike the previously described polymers, it is soluble above pH 5.5. However, when combined with other polymers such as Eudragit RL 30 D in the right ratio, a certain dissolution pH can be achieved. For example, Chen et al. (74) coated azithromycin-loaded pellets with a mixture of Eudragit RL 30 D and L 30 D-55 in a ratio of 1:4 (w/w). The coated pellets showed rapid release in the gastric environment (pH up to 3), whereas the release of the drug in the oral environment (pH 5–7) was avoided. Eudragit RL 30 D, on the other hand, has pH-independent solubility. It is provided as an aqueous dispersion of a copolymer of ethyl acrylate, methyl methacrylate, and a small amount of methacrylic acid ester with quaternary ammonium groups. Eudragit L 30 D-55 is also provided as an aqueous dispersion, but the components of this copolymer are methacrylic acid and ethyl acrylate copolymer in the ratio 1:1 (82–84).
Usually, the taste-masking polymer is not the only component of the coating. Plasticisers are a common component of a film coating to ensure better distribution of the coating solution and thus the formation of a coherent film. They act by lowering the glass transition temperature of the polymers, which improves the mechanical properties of the film coating and reduces its brittleness (85). The type and concentration of a plasticizer can significantly affect film properties (86). The most commonly used plasticizers are triethyl citrate and polyethylene glycol, but this list is not exhaustive. It should be mentioned that the use of a plasticizer in the coating also has disadvantages. For example, Stange et al. (71) reported that some polymers, such as Eudragit E 100, are sticky, which can lead to aggregation and agglomeration, and this can be exacerbated by the addition of a plasticizer. Instead of adding an additional component, they suspended the polymer in a mixture of water and ethanol before film coating. Since water itself acts as a plasticizer, 12 % ( m/m) water in the coating suspension was sufficient for a coherent film.
Anti-tacking agents are also frequently used in film coatings to reduce agglomeration and aggregation. Talc or magnesium stearate are commonly used for this purpose. The amount of talc in the coating is often quite high, i.e., even up to 50 % ( m/m) (71, 73), while magnesium stearate is usually used in small amounts, i.e., up to 2 % ( m/m) (60, 63), although there are exceptions with as much as 35 % ( m/m) magnesium stearate in the coating (68).
Another important ingredient in a coating that is commonly used is a surfactant, such as sodium lauryl sulfate. This is because the addition of a surfactant can improve the penetration and spreading properties of the coating liquid, which can otherwise be hindered by the hydrophobic components of the coating or core dosage form. Other ingredients that may also be used include colorants and antioxidants (86). Pigments can also be used in film coatings because they are insoluble in water and thus tend not to migrate into and between coatings, as can be the case when using colorants (87).
Table II. Studies dealing with taste masking of multiparticulates by film coating
NA – not available, ODTs – orodispersible tablets, HPMC – hydroxypropyl methylcellulose, PEG – polyethylene glycol, SLS – sodium lauryl sulphate, EC – ethyl cellulose
As shown in Table II, the amount of coating required to achieve complete taste masking is case-specific and ranges from 5 to 62 % ( m/m). Interestingly, both of these values were achieved with the same polymer, Eudragit E 100, applied to the multiparticulates by the fluid bed method. In the formulation with 5 % coating, diclofenac sodium was taste-masked, while in the formulation with 62 % coating, the used API was naproxen sodium. Considering this, the bitter taste of naproxen seems to be detected faster than that of diclofenac sodium. However, looking at the bitterness threshold values in Table II, it appears that diclofenac sodium is far more bitter than naproxen, which is somewhat surprising. Nevertheless, Stange et al. (71) acknowledged in their study that their formulation could contain a lower percentage of coating and still be an effective taste masking agent. Looking more closely at the relationship between the reported bitterness threshold value and the percentage of coating, there is no clear correlation, meaning that a low bitterness threshold value does not necessarily mean that the percentage of coating was high. This suggests that other factors besides the amount of coating are important for taste masking, such as the coating method, the type of polymer, and coating coherency. In addition, particle properties are also of utmost importance. For example, smaller particles have a higher specific surface area, so larger coating amounts are required. The morphology of the particles and their friability can also play an important role. For example, irregularly shaped and brittle particles are more difficult to coat, and thus taste masking is less efficient (24).
EVALUATION OF TASTE MASKING
To assess the effectiveness of taste masking, different evaluation methods can be used, which can be briefly divided into in vivo and in vitro methods.
In vivo methods are usually performed with human volunteers and, despite their high cost and ethical and toxicological problems, are still a very common approach for evaluating taste masking (88, 89). Moreover, their use in the paediatric population is even more problematic due to restrictions and safety concerns. Therefore, it is more appropriate to use the adult population, even though their taste perception may differ from that of children The common procedure of these methods is as follows: A group of subjects is asked to hold the sample in the oral cavity for a specified time, after which they must rate the taste using a numerical or descriptive scale. In addition, subjects are sometimes asked to rate other sensory properties of the samples, such as palatability, mouthfeel, roughness, aftertaste, etc. (63, 71–73). The fact that such properties can only be evaluated by humans could be considered an important advantage of in vivo methods, as these properties can significantly influence the acceptability of the product by patients (71). Because there are no standard protocols for taste masking evaluation (88), the in vivo methods described in research are quite versatile in terms of the number of subjects, time points to assess the taste, and the rating system. The group of volunteers is usually quite small, i.e., about 10 subjects, and it is usually emphasised that they were healthy at the time of testing, which is important because some diseases (influenza, covid-19, etc.) can affect taste perception. Usually, the volunteers are asked not to swallow the investigated sample and should rinse their mouths with water afterwards. The time required to evaluate the taste of the sample can range from 5 seconds to several minutes, which limits to some extent the comparability of the results of different studies. Because multiparticulates are generally easy to swallow, time intervals longer than a minute might seem long, but on the other hand, it is possible that small particles get stuck between the teeth and are swallowed later (71, 90). Therefore, it is useful to determine the amount of fluid to be taken with the medication to ensure that an adequate amount of particles is flushed from the oral cavity. Scoring systems typically include up to five numeric or descriptive options or levels to assess bitterness or other taste qualities. The literature also mentions that facial hedonic scales are appropriate for younger children but are less commonly used because they provide less information than other scoring systems (91).
In vitro methods can generally be divided into electronic tongue measurements and drug release testing (36, 92). Electronic tongues or e-tongues are sensory array systems that can detect individual substances or mixtures by sensor membranes and electrochemical techniques (93). These sensors are designed to mimic taste buds by eliciting changes in electrochemical potentials that should be compared with the actual physiological action potentials that are induced during taste perception (94). After measuring the samples, which must be solutions, the results are usually analyzed by principal component analysis (PCA) or similar statistical methods. To assess whether taste masking has been achieved, the distances between samples and placebos or pleasant and bitter taste references are evaluated in two-dimensional PCA maps (93–96). Although e-tongues have become an important and useful tool in taste masking research, one must be cautious in interpreting the results. For example, sensors respond differently to charged and uncharged molecules, and furthermore, interactions between formulation components can lead to misleading results (93).
Drug release assays for taste masking evaluation are a widely used method, most likely due to their simplicity, objectivity, low cost, and time efficiency. However, there is a lack of a standardized procedure for conducting the analysis. Therefore, there are a variety of in vitro drug release methods that differ in terms of the volume of dissolution medium, its composition, pH, duration of the test, and the device in which the test is performed. The most commonly used dissolution medium is phosphate buffer with a pH of 6.8 and a temperature of 37 °C (58, 60, 71, 97, 98). There are also some cases where distilled water (sometimes with a surfactant) was used instead (68, 93, 95). Sometimes simulated salivary fluid (SSF) is used to closely mimic conditions in the oral cavity, but the composition is not the same in every study (55, 65, 73, 99). Regarding the volume of the dissolution medium, it would probably be most appropriate to use the approximate volume of residual saliva in the oral cavity, which is 1–2 mL (88). However, the volume of the dissolution medium has been found to range from 2 to 900 mL, which is very different from the principles of physiological conditions. There is also considerable variation in the sampling time required to estimate the efficiency of taste masking, which goes even up to 15 min. Interestingly, there are large differences between in vivo and in vitro time points for estimating taste masking, and it would probably be more appropriate to use similar and, more importantly, physiologically relevant time intervals in both types of experiments. Indeed, drug release after 5 minutes can hardly serve as a metric for successful taste masking, since the residence time of oral dosage forms in the mouth is probably much shorter.
Although the USP I/ II apparatus or the shake flask method are commonly used to perform drug release assays, some attempts have been made to better simulate the oral cavity. For example, Keeley et al. developed a flow-through dissolution column (Fig. 2) and compared it to the USP I dissolution test. While both systems were able to distinguish between different types and levels of coating to mask chlorphenamine taste, only the novel dissolution test could give absolute quantification of taste masking based on previously determined taste thresholds. Although the authors also mention the limited size of the dosage forms as a disadvantage of this method, this is irrelevant for multiparticulates, as they are small enough to be introduced into the device (70).
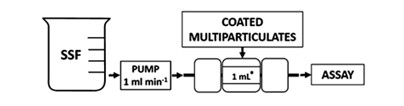
Fig. 2. Flow-through dissolution column designed by Keeley et al. Reprinted from reference (70) with permission from the American Chemical Society (ACS).
It is also important to note that the results of the drug release experiment should be compared to a specific reference value in order to claim the effectiveness of taste masking with greater confidence. The most commonly used, and probably most advisable, reference value to which to compare results is the bitterness threshold value, which is the concentration of the substance above which a bitter taste can be detected. This value is substance-specific and its determination is defined in the European Pharmacopeia in Test 2.8.15, "Bitterness Value" (100). A minimum of six volunteers are recommended, who should rinse their mouths before testing. To correct for differences in taste perception among panel members, a correction factor is first determined across different concentrations of quinine hydrochloride solution. To determine at which concentration the bitter taste of the API is detectable, several dilutions of the drug are prepared according to a specific protocol. If the concentration in the dissolution medium after a certain time point is higher than the specified value, taste masking has not been fully achieved. However, it should be noted that the value reported in the literature may also vary. In addition, if sweeteners or aromas are added to the formulation, they may compensate for the initial release of the bitter API, and taste masking may still be achieved. Another possible reference for comparing release results is the FIP /AAPS (Federation International Pharmaceutique/American Association of Pharmaceutical Sciences) guideline, which states that less than 10 % ( m/V) of the API should be released within 5 minutes to ensure taste masking (101). However, since this is an arbitrary value, it may not be applicable to every single substance and also depends on the dissolution method used (95). Although there are studies that evaluate taste masking based on the dissolution profile of their formulation in the first few minutes compared with the dissolution of the pure API, this comparison may not be appropriate and could lead to misinterpretation of the results. In this case, it is advisable to use a complementary method to evaluate taste masking, such as e-tongue or in vivo tests.
TASTE MASKING OF POORLY WATER-SOLUBLE DRUGS IN MULTIPARTICULATES
As mentioned earlier, the principle of taste masking is usually to prevent contact between a bitter-tasting API and the taste buds by slowing the dissolution of the drug in the oral cavity. However, it is possible that this may also reduce the dissolution of the API further down the gastrointestinal tract. For highly soluble APIs, this may not be a problem if their concentration remains high enough to reach the therapeutic range after absorption. For poorly soluble APIs, on the other hand, this could result in concentrations that are below therapeutic levels. For this reason, taste masking of poorly soluble APIs presents a particular technological challenge. It is therefore not surprising that there are only a limited number of studies in which taste-masked multiparticulate drugs have been formulated using a (model) poorly water-soluble API (Table III).
Table III. Overview of publications dealing with taste-masked multiparticulates including a poorly water-soluble API
a If more than one excipient was tested, only the one giving the best results is given. See the text below for further explanation.
As shown in Table III, most researchers used either Eudragit E or Eudragit E PO, which are both enterosoluble polymers, to mask the taste of bitter-tasting, poorly water-soluble substances. In this way, the polymer prevents the taste buds from coming into contact with the API, but immediately after entering the stomach, the polymer dissolves and no longer interferes with the dissolution process of the API.
Münster et al. (55) attempted to develop a formulation that combined immediate release and taste masking of poorly water-soluble praziquantel. They tested several polymers (Eudragit E PO, RL PO, L100, S100, Kollidon SR) to determine which exhibited the best miscibility with praziquantel. Since dynamic scanning calorimetry (DSC) measurements showed no difference between samples, a small-scale drug release study was conducted in SSF, simulated gastric fluid sine pepsin (spSGF), and fasted state simulated intestinal fluid (FaSSIF) with films of amorphous solid dispersions in a 96-well plate. It was found that Eudragit E PO reduced drug release by 6-fold in SSF compared to pure API, but allowed the highest degree of supersaturation in the other two media. Hot melt extrusion was used to prepare a multiparticulate formulation, and glyceryl monostearate was used as an additional excipient for taste masking, since it is insoluble in water, and as a lubricant to ensure better flowability of the mixture. In fact, it enhanced the taste masking effect by up to 11-fold compared to the pure polymer, as determined by dissolution in SSF, and concentrations of praziquantel remained below the reported bitterness threshold value for the first 5 min. However, as the authors pointed out, these results would need to be confirmed in vivo. Although praziquantel was present in the extrudates in a crystalline state, dissolution in spSGF and FaSSIF was nevertheless improved compared with the pure API due to the improved wettability provided by Eudragit E PO and glyceryl monostearate, as well as the solubilizing effect of Eudragit E PO. The fact that the API was not amorphous also contributed to the stability of the formulations after eight weeks of storage under various conditions, as evidenced by only minor differences in drug release.
Alshetaili et al. (94) used ketoprofen as a poorly soluble, bitter-tasting model drug and mixed it with Eudragit E PO by hot melt extrusion. In this case, no other excipient was needed because ketoprofen itself acted as a plasticizer. The multiparticulate dosage form they formed the extrudate into was mini tablets. DSC measurements confirmed that ketoprofen was completely amorphous in the mini-tablets. When dissolution in an acidic medium was tested, formulations containing 20, 30 and 40 % ketoprofen showed 100 % release within 20 minutes, which was attributed to the solubilizing effect of Eudragit E PO and salt formation between the acidic groups in ketoprofen and the tertiary ammonium groups in the polymer. This was also confirmed by Fourier transform infrared spectroscopy (FTIR). Pure ketoprofen was released only about 20 % in 120 min, showing a significant improvement. In SSF with a pH of 6.8, drug release was less than 0.5 % in the first 2 minutes. Although nothing was said about the threshold value for ketoprofen, it could be concluded that taste masking was indeed achieved, since the formulations were also tested with e-tongue and it was found that the "taste" of the samples was very similar to that of the buffer. Interestingly, after three months of storage, the samples remained amorphous and showed similar release profiles to the freshly prepared samples, which was also attributed to strong interactions between the drug and the polymer.
Stange et al. (71) developed a multiparticulate formulation with naproxen coated with Eudragit E as a taste masking agent. Unlike previous studies in which Eudragit E was used as a film coating polymer, their coating did not contain plasticizers because, as mentioned earlier, these can increase tacking tendency. Instead, they used only talc and a mixture of water and ethanol for the coating, which was done in a fluidized bed coater. As the amount of water increased, the coating efficiency increased and provided better taste masking. Drug release was tested in a two-stage experiment, first in acidic media and after 30 minutes in pH 6.8. After 30 minutes in acidic media, which is supposed to be the shortest residence time of the pellets in the stomach, more than 80 % of naproxen was released from the selected formulation. At a pH of 6.8, only the results for the first 5 minutes are reported, so it is not possible to determine how much API was actually released at the end of the experiment. In addition, no comparison with the dissolution of the pure API is given, so it is difficult to say whether the dissolution rate has changed due to the coating.
Alotaibi et al. (73) developed taste-masked pellets that contained diclofenac sodium, which were subsequently formulated into orodispersible tablets. The sugar pellets were triple-layered; the first layer consisted of diclofenac sodium and HPMC (binder), the second layer was enteric coating consisting of Eudragit L100, talc (anti-adherent), and triethyl citrate (plasticizer), and the third layer was a taste-masking coating made of Eudragit E100, talc (anti-adherent) and PEG 6000 (plasticizer). Dissolution experiments with pellets prepared in a two-step manner (first 2 h at pH 1.2, then 3 h at pH 6.8) showed that the taste-masking coating did not affect drug release in SIF, as Eudragit E100 dissolves in an acidic medium. In SSF, the enteric coating did not significantly delay drug release, but even with 5 % Eudragit E100, drug release was only 3.0 % in 3 minutes. Orodispersible tablets (200 mg) were prepared with the pellets that exhibited the best properties, i.e., the fastest dissolution at pH 6.8 and the slowest dissolution in SSF (12 % Eudragit L100, 5 % Eudragit E100). Different formulations were prepared with varying amounts of superdisintegrants (croscarmellose sodium or crospovidone), microcrystalline cellulose, sodium stearyl fumarate, colloidal silicon dioxide, and mannitol. Mannitol is a commonly used excipient in orodispersible formulations due to its cool, sweet, and mild taste and pleasant mouthfeel due to its negative heat of solution. The formulation that showed the fastest drug release at pH 6.8 and the lowest release at acidic pH was compared with the commercially available Voltaren 25 mg. Drug release in the acidic medium was limited in both cases. However, at pH 6.8, the release rate of the selected formulation was significantly higher than that of Voltaren, suggesting a shorter onset of action and thus faster pain relief. In addition, in vivo studies have shown that the developed tablets effectively mask the bitter taste and aftertaste as well as provide good mouthfeel. The fact that the tablets are small and contain the correct dose of the drug also speaks to their superiority over the marketed formulation.
Yıldız et al. (65) also used Eudragit E (6 or 8 % m/m) to prepare taste-masked mirtazapine granules by coacervation, which is considered an economical approach. They also produced orodispersible tablets from the multiparticulates using some additional excipients to ensure the possibility of direct compression, which is also an economical method. The mass of the tablets produced was somewhat large (> 500 mg), which is not desirable for orodispersible formulations. However, the disintegration times were less than 30 seconds, so a large mass was not an issue. The dissolution profiles at three different pH values (1.2, 4.5, and 6.8) were compared with the marketed formulation Remeron SolTab and showed almost no differences. However, taste masking studies in SSF showed that the marketed formulation had lower dissolution and thus more efficient taste masking than all developed formulations, regardless of the coating ratio. The authors concluded that a higher coating ratio would be required to fully mask the taste of mirtazapine.
Only a few studies used other types of polymers to cover the taste of poorly water-soluble APIs. Al-Omran et al. (63) attempted to mask the taste of diclofenac sodium without reducing its release rate by incorporating it into microcapsules. Their core consisted of the API, lactose, and microcrystalline cellulose, while their wall was based on ethylcellulose. Diethyl phthalate or PEG 600 in various ratios were used as plasticisers in the wall, and magnesium stearate was used to prevent aggregation of the particles. Organic phase separation with toluene as solvent and petroleum ether as non-solvent was used to formulate the microcapsules. It should be noted that toluene in particular is toxic and its use for pharmaceutical products is therefore questionable. Dissolution experiments in phosphate buffer with a pH of 7.4 showed that the release rate depends on the type and concentration of plasticizer, the shape of the core material, the core size, and the core-to-wall ratio. They also compared their formulation to a marketed product Voltaren 25 mg and found that the release rate, as well as the amount of drug released, was higher for a formulation with a core-to-wall ratio of 1:1 and 20 % ( m/m) PEG 600 as a plasticiser, and in formulation with a core-to-wall ratio of 2:1 and 20 % ( m/m) diethyl phthalate as a plasticiser. However, in vivo tests to evaluate the palatability and taste of the formulations showed the superiority of the formulations with a higher wall ratio, making the former the better formulation. Since the article does not provide numerical values or comparisons to evaluate taste and palatability, it is difficult to draw further conclusions.
Chen et al. (74) dealt with taste masking and enhanced absorption of azithromycin, which is poorly soluble in the alkaline but well soluble in the acidic pH. The pellets loaded with the API were therefore coated with a mixture of two Eudragit-type polymers, RL30D and L30D-55. As mentioned earlier, the first has pH-independent swelling properties and is insoluble in water, while the second dissolves only in media with a pH above 5.5. Triethyl citrate was added to the coating as a plasticizer. The coating was applied in a fluidized bed processor. The dissolution test at a pH of 1.2 showed that the entire API was released within 120 minutes. However, after 40 minutes, the drug release was less than 20 %. Since it is known that the residence time of the pellets in the stomach may be shorter, this result might not be very promising. In addition, the dissolution study was conducted with 100 mg of API, while the recommended dose is either 500 mg or 250 mg, which also limits the relevance of these experiments.
In contrast to the previously described studies, Kharb et al. (97) did not use a polymeric substance to mask the taste of a bitter-tasting substance. Instead, Geleol (a mixture of glycerol monostearate and glyceryl stearate) was used to prepare lipid-matrix pellets with ondansetron hydrochloride dihydrate as the API. Several formulations with different ratios of Geleol were tested, but only one had drug release in phosphate buffer at pH 6.8 below the bitterness threshold concentration, indicating successful taste masking. Dissolution tests at pH 1.2 showed that drug release from this formulation was significantly slower than from pure ondansetron (< 40 % vs. 100 % after 120 minutes). To improve the slow drug release, fumed silica (Cab-o-sil) was added to the formulation to ensure faster drug release due to its hydrophilicity and gelling properties. After 120 minutes, almost 70 % of the API from the batch with 3 % Cab-o-sil had been released, so an improvement in the release of the API was evident, although it did not reach the release rate of the pure API.
Obaidat et al. (99) also prepared a taste-masked ibuprofen solid dispersion by incorporating it into a lipid substance, specifically cocoa butter microparticles, using supercritical fluid technology, a technique rarely used in taste masking research. The prepared ratios of ibuprofen were 10, 20 and 30 % ( m/m). For all samples, PXRD measurements showed a decrease in the crystallinity of the drug, while DSC showed complete miscibility in the molten carrier. However, the authors suggest that some of the drug was precipitated in crystalline form due to the weak interactions between the carrier and the drug. Dissolution experiments in phosphate buffer with a pH of 7.2 interestingly showed a significantly lower drug release in the first 20 minutes compared to the drug release of pure ibuprofen, despite the partially amorphous state of the samples. The authors suggest that this may be due to the hydrophobic nature of cocoa butter. However, after 45 minutes, the 10 and 20 % samples showed no statistically significant difference in drug release compared to pure ibuprofen or physical mixtures. However, it is possible that drug release from such formulations is faster in vivo due to enzymatic activity and surface-active agents that contribute to the degradation of lipid components. Since the amorphous state is prone to physical instability, the effect of storage conditions on the physical state and dissolution profile was also tested. Refrigerator storage appeared to provide satisfactory stability of the samples, while room temperature storage resulted in an increase in the crystallinity of the samples. Since the storage time was only 6 weeks, the prepared formulations did not seem to exhibit high physical stability.
Khor et al. (102) attempted to mask the taste of plant-derived flavonoid quercetin with three different water-insoluble excipients: shellac (natural resin secreted by the insect Kerria lacca composed of polyhydroxy polycarboxylic esters and single esters, carnauba wax (Brazilian palm tree wax composed mainly of esters), and zein (water-insoluble prolamine isolated from corn gluten). They use hot-melt extrusion to formulate microcapsules with varying ratios of excipient to API and 20 % ( m/m) water as plasticisers. Taste masking experiments performed in vitro in SSF with pH 6.8 and with an electronic tongue system showed that both carnauba wax and zein decreased the dissolution of quercetin, while shellac actually increased it. This was explained by the high solubility of shellac at pH 6.8 and/or the lower degree of quercetin crystallinity. This may even suggest that shellac was not the optimal choice for taste masking in the first place. Electronic tongue tests also confirmed that the other two excipients performed better in taste masking, but unlike the dissolution tests, shellac performed better than pure quercetin. This shows that the taste masking methods are not absolute and that it is advisable to perform more tests to obtain more meaningful results. The in vitro dissolution test, conducted at a pH of 1.0 (the first 120 minutes) and a pH of 6.8 (120–240 minutes), showed that the formulation containing 60 % shellac had the highest percentage of released quercetin after 240 minutes. However, since it was found to be ineffective in taste masking, the formulation with 70 % carnauba wax might be the better one, as the percentage of released API was similar to that of pure quercetin and its dissolution rate at pH 1.0 was also the highest among the tested formulations.
From the research reviewed in this section, it can be seen that the simultaneous implementation of techniques to improve the dissolution of poorly water-soluble drugs and to conceal their taste is rare. In fact, only 3 studies (55, 94, 99) used techniques such as preparation of solid dispersions and/or amorphization to improve dissolution and also showed that it is improved compared to pure API. There is some research (63, 65, 73) that compared their formulation to the marketed product and showed either equivalent or improved drug release properties, which is also a relevant comparison since it at least ensures that drug release is sufficient to achieve the therapeutic effect. On the other hand, in some research (74, 97), drug release from the prepared formulation was worsened compared to pure API due to taste masking approaches, while Stange et al. (71) do not show results that would deal with drug release in detail. Moreover, a significant lack of in vivo results was noticed; not only regarding taste masking, but also regarding drug absorption. In fact, these results would probably give the best indication as to whether taste masking of poorly water-soluble drugs is truly feasible.
CONCLUSIONS
The increasing use of multiparticulate formulations definitely represents a step forward in the development of child-friendly medications, as they ensure precise dosing and are well tolerated by children of all ages. Although much research has been done to ensure taste masking of bitter-tasting substances, this remains a challenging task as there is no optimal approach and no universal optimal method. Taste masking by film coating appears to be the preferred approach for multiparticulate as well as for other drug delivery systems, especially with polymers that are insoluble in saliva but dissolve in gastric fluid. In fact, this approach also appears to be the best when the drug is poorly water-soluble, as it is least likely to interfere with API dissolution after it leaves the oral cavity. However, because masking the taste of poorly water-soluble APIs is a major challenge, there are not many studies addressing this issue. In addition, it is often not clear how much the dissolution profile of such APIs is compromised by taste-masking excipients. In vivo approaches to assess taste masking are still considered the gold standard, but this is ethically questionable, particularly for the paediatric population. A combination of in vitro methods, i.e. dissolution in SSF and e-tongue, might thus be more feasible for paediatric medicine. Future research and development should therefore deal with evaluating their formulations regarding taste masking and drug release with methods that would give more relevant and meaningful results. Furthermore, it should put a greater emphasis on utilizing techniques to improve water solubility when dealing with poorly water-soluble drugs so as to avoid compromising sufficient drug release with taste masking. It is expected that taste-masked multiparticulate formulations will continue to be the subject of research and that more such formulations will be marketed in the future.
Availability of data and materials. – The datasets generated during and/or analysed during the current study are available from the corresponding author upon reasonable request.
Funding. – This research was co-funded by the Slovenian Research Agency (research core funding P1-0189). The Slovenian Research Agency had no role in the design and conduct of the study; analysis and interpretation of data; preparation, approval and submission of the manuscript for publication.
Conflict of interest. – The authors declare that they have no competing interests.
Authors contributions. – All authors contributed to the study conception, design and contributed to the data collection. A. Baumgartner interpreted the data and drafted the manuscript. All authors have read and approved the entire manuscript.