Introduction
Camel milk ( Camelus dromedarius) is one of the most important dietary resources in hot climatic zones (Ziaeifar et al., 2018). It differs from bovine milk in terms of composition, protein content and structure, and hence is expected to have functional and bioactive properties unique from bovine milk (Ereifej et al., 2011). Camel milk protein content has been widely researched. Camel milk has less β-lactoglobulin and more β-caseins, α-lactalbumin, and serum albumin than bovine milk (El-Agamy, 2009). Casein micelles account for 75 to 80 % of total proteins in camel milk and are complex association colloids comprised of four naturally phosphoproteins, αS1, αS2, β, and κ-casein, as well as colloidal calcium phosphate (Markoska et al., 2021). The κ-casein forms a so-called "hairy" layer on the outside of the casein micelles, with a thickness of about 5-10 nm and is extremely susceptible to proteolysis and structural modification during coagulation (Al-Shamsi et al., 2018).
Different treatments, including chemical (acid) and biological (rennet) gelation methods, could influence the structural and functional characteristics of casein proteins such as emulsifying behaviour (Ortiz et al., 2004). Acidification and renneting are the two most common procedures for coagulating casein micelles (Acem et al., 2018; Acem et al., 2020). Processes that cause gelation are somewhat distinct. The acid coagulation process affects both the micellar core and the surface κ-casein layer (De Kruif et al., 2012). Because of the loss of colloidal calcium, acid gels are usually more brittle than rennet gels (Li and Zhao, 2019). Acid aggregation is commonly accomplished by the addition of HCL, GDL (Glucono-Delta-Lactone), or by the presence of starter culture (Bjekić et al., 2021). The rennet reaction, on the other hand, is particular hydrolysis that affects the κ-casein layer (Li and Zhao, 2019). Rennet is responsible for the particular cleavage between the (Phe 97-Ile 98) link of camel κ-casein (Hailu et al., 2016). This cleavage divides κ-casein into hydrophobic para-κ-casein, which stays on the surface of the casein micelle, and hydrophilic caseino-macropeptide, which is cleaved off the casein micelle, resulting in the instability of casein micelles and, as a result, milk aggregation (Freitas et al., 2019).
Casein micelles are commonly used as emulsifiers in the formulation of food emulsions due to their amphiphilic nature (Foegeding and Davis, 2011). Caseins' capacity to create repulsive contacts between oil droplets while also forming a rupture-resistant interfacial barrier plays a key role in preventing flocculation and coalescence during long-term storage (Dickinson, 1999). Likewise, it is now established that the functional characteristics of stabilizers/emulsifiers such as casein proteins are heavily influenced by the surface composition and surface features of the elements (Gaiani et al., 2006). However, by altering the κ-casein surface layer of casein micelles (renneting, acidification), interactions between caseins or with oil droplets in food emulsions can be improved or delayed. Therefore, understanding the morphology and the surface composition of acid and rennet casein micelles is critical for enhancing its processing stability and regulating its functionalities (Li and Zhao, 2019). To our knowledge, so far only few studies have been focused on the manufacture of spray-dried camel milk proteins (Abu-Lehia et al., 1989; Sulieman et al., 2014; Zouari et al., 2020). However, none of these researches have studied the surface features of camel casein protein powders (Murrieta-Pazos et al., 2012). Consequently, this study will focus on the two frequently used techniques in the dairy industry: acidification and enzymatic treatment, their effects on the surface composition and morphology of whole camel caseins, and their impacts on emulsifying proprieties.
To validate all of the aforementioned, surface characterization techniques known as X-ray photoelectron spectroscopy (XPS), X-Ray Diffractometer (XRD) and Scanning Electron Microscopy (SEM) will be used. These techniques should result in precise information about the distribution of relative atomic elemental composition at the surface, crystal structure, and secondary structures of the camel casein micelles, respectively. While emulsifying properties of the acid and rennet camel casein powders were studied in terms of the emulsion’s stability over time and the size of oil droplets.
Materials and methods
Materials
Camel ( Camelus dromedarius) fresh milk samples utilized in this study were collected from a local camel farm in the Ghardaïa province (the south of Algeria). The samples were promptly cooled down to 4 °C and transferred to the laboratory within 24 hours of being collected. After collecting the milk and before storing it, the pH levels were measured immediately with a (Adwa AD 8000 pH meter, Romania).
Preparation of samples
The pre-heated milk (30 °C) was skimmed twice by centrifugation (Froilabo SW12RH Centrifuge, France) at 4000 × g for 20 min at 4°C (Felfoul et al., 2017). To achieve full elimination of residual milk fat, skimmed camel milk was filtered three times through Whatman paper filter (Whatman N°40, Maidstone, UK). After that, the defatted milk was stored at 4 °C until further processing and analysis.
Acid coagulation
Caseins and whey proteins were completely separated using a slightly modified technique of Salami et al. (2011). Specifically, the skimmed milk was acidified by adding a few drops of (1N HCl), to reduce pH to 4.3 which is the isoelectric point of camel milk caseins at which precipitation starts. After precipitation the obtained camel caseins were suspended in deionized water and centrifuged again (4000 × g, 60 min, 4 °C). This operation was repeated three times to remove any whey proteins residue (Gaiani et al., 2005). The caseins recovered were dissolved in NaOH (1 mol/L). Finally, the obtained camel casein fractionwas lyophilized using a CHRIST freeze dryer (Alpha 2-4 LSCbasic) at -72 °C, 10.000 Pa pressure and kept in an airtight container at 4 °C for future analysis.
Rennet-induced coagulation
Rennet is an enzyme complex that is widely used in the making of cheese. The active enzyme in rennet is chymosin (Li and Zhao, 2019). The preheated (35 °C) defatted camel milk was coagulated by adding 1 % (w/v) of chymosin (Chr-Max Powder Extra, 2235 IMCU/g; Chr. Hansen Inc., Denmark). The samples were stirred for 40 min and stored at 4 °C for 24h. After precipitation, the obtained rennet caseins were treated as previously described for the acid coagulation.
Surface composition analysis using XPS
XPS is a surface analysis method (Rouxhet and Genet, 2011; Murrieta-Pazos et al., 2012), which was frequently reported as an importing technique for determining the surface composition of dairy powders (Millqvist-Fureby and Smith, 2007; Gaiani et al., 2011).
The XPS measurements were carried out on a Kratos Axis Ultra spectrometer (Kratos Analytical, Manchester, UK) working with a monochromatic AlKα X-ray source (E = 1486.6 eV; P = 90 W). The X-ray source was operated at 90 W to avoid the X-ray-induced degradation of the samples (Gaiani et al., 2011). Rennet and acid camel casein samples were applied to a sample holder using a double-sided conductive adhesive tape. The assemblage was then degassed overnight before being analysed. The XPS was performed in an ultra-high vacuum (10-8 Pa) with a charge neutralizer engaged. High-resolution spectra were obtained at pass energy of 20 eV, with an energy resolution of 0.9 eV. To adjust the binding energies for charge energy shift, the C 1s line of 284.4 eV was used as a reference. The collected spectra (C1s, O1s, and N1s) were analysed using Kratos Vision software (Vision 2.2.2) utilizing the transmission coefficients and photoemission cross-sections included in the Vision software package (Zouari et al., 2020).
Crystallography analysis using X-Ray Diffractometer
The crystallography structure of the camel casein powders (acid and rennet) was examined by an X-ray diffraction instrument (Siemens D5000 diffractometer) using (Cu-Kα1, α2) nickel-filtered radiation of wavelength 1.5405 Å in Bragg-Brentano configuration. Measurements were carried out in continuous scan mode from 5 to 100° (2θ), with a step size of 0.0083555 (2 θ). The X-ray tube (Cu-Kα1, α2) was set to 45 kV and a current of 30 mA.
Morphology analysis using Scanning Electron Microscopy
The morphology of acid and rennet camel caseins was examined by Scanning Electron Microscopy (SEM) using a JEOL JSM 7600F apparatus (JEOL UK Ltd., Welwyn Garden City, England). The samples were placed on double-sided sticky tape connected to SEM stubs and coated with platinum of 10 nm thickness to make them conductive before being sputtered with gold (Young et al., 1993). The experiments were carried out on images captured with an SEM, Quanta 200FEG-SEM (FEI Co. The Netherlands), at 10 kV accelerated voltage and 7.3 mm working distance.
Preparation of model oil-in-water emulsions
Oil-in-water emulsions were created by the dispersed phase (Virgin olive oil was obtained from a local commercial supplier, production 2019 brand CHIALI, Sidi-bel-Abbes, Algeria) and aqueous phases. The emulsions were prepared at casein concentration 2 % in an O/W fraction of 0.4. A hand homogenizer (Bomann, Stabmixer SM 354 CB) was used at room temperature to 18000 rpm for 1 min to prepare all mixtures.
Emulsifying stability
The capacity of emulsion droplets to remain distributed without creaming, flocculating, or coalescing is described as the emulsion stability index (ESI) (Zayas, 1997). A volume of 30 mL (60 %, v/v) of the acid camel sodium caseinate samples (2 %) was homogenized with 20 mL (40 %, v/v) of virgin olive oil for one minute at room temperature using the homogenizer (Bomann, Stabmixer SM 354 CB) performed at 18000 rpm (Lajnaf et al., 2020). Following that, an aliquot of each freshly prepared emulsion (0.1 mL) was pipetted and diluted in 9.9 mL of deionized water. The diluted emulsion mixture was vortexed for 10 seconds, and the absorbance was measured at λ = 500 nm with a spectrophotometer (Uviline 9400 SECOMAM France). Emulsions were left undisturbed for 30 minutes before aliquots of 0.1 mL were collected and dispersed in 9.9 mL of deionized water. The absorbance was also measured at λ=500 nm, as previously described. The same protocol was performed to determine the (ESI) of the rennet camel sodium caseinates.
The emulsion stability index (ESI, %) of camel casein was determined using the following equation suggested by Pearce and Kinsella (1978):

Where ( A0) and ( At) indicate the absorbance at 500 nm at time zero and after, respectively (30, 60, 90, 120, 150, and 180 min).
Droplet size measurement
An optical microscope (NOVEX B- Range, Holland) was used to measure the average diameter of the fatty globules using an ocular micrometre graded from 0 to 10, with graduations spaced 0.1 µm apart. One drop of the resulting emulsion was blended with 0.05 mL of 1 % Soudan III dye (w/v) (Acem and Choukri, 2012). Following that, the mixture was put between the lame and lamella of the previously mentioned optical microscope with a 40 objective magnification and linked to a digital camera (DOM 300, China).
Statistical analysis
The statistical analysis was carried out using the SPSS (Version 19) statistical analysis program. All measurements were made in triplicate, and the findings were represented as the mean standard deviation. To compare various groups, a one-way analysis of variance (ANOVA) was used, and a p-value of 0.05 was considered statistically significant.
Results and discussion
XRD analysis
XRD was used to investigate the crystallographic characteristics of two camel casein precipitates (Figure 1). Table 1 shows the characteristics of the major diffraction peaks from acid and rennet camel caseins. Casein proteins extracted with HCL and chymosin revealed a large crystalline peak at a 2 θ value of approximately 20° and a tiny peak at a 2 θ value of about 10°, respectively (Figure 1). The angle positions of the two proteins were not statistically different (p<0.05). The average particle distance ( dhkl) of casein precipitates produced by acid extraction and enzymatic coagulation at 2 θ around 19° was not substantially different (p<0.05). However, at 2 θ around 10°, the mean spacing between particles achieved by acidification was somewhat larger than that acquired through enzyme coagulation. The findings revealed that the backbone structure of casein precipitates varied just slightly (Zhao et al., 2008). The literature reported that in diffractogram patterns, the peak angles of α-helix and β-sheet structure from proteins were at 2 θ about 10° and 20°, respectively (Zhao et al., 2015). The strength of a peak can also reflect changes in a protein's structure. The intensities of camel caseins extracted using the two techniques at 2 θ around 9° were not substantially different (p<0.05) in this study. The results revealed that the fraction of α-helix in casein proteins produced by the two coagulation techniques was not substantially different. Conversely, the intensity of the peak at 2 θ about 19° of the camel casein obtained by chemical coagulation (188.22 %) was higher than that obtained by biological aggregation (129.81%), indicating a greater β-sheet content in the previous structure, which led to a significant rise in the crystal structure. This showed that acid may affect the amorphous structure of casein.
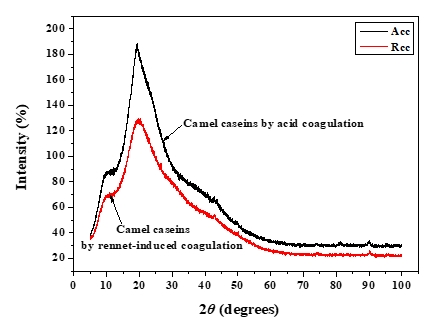
Figure 1. XRD patterns of camel caseins powders
Table 1. XRD’s parameters of camel caseins gotten through acid and rennet coagulation

Scanning electron microscopy
Figure 2 illustrates the microstructure of camel casein powders extracted chemically and biologically. The microstructure of acid casein precipitate (Figure 2a) contained no holes dispersed on the surface. The casein precipitate produced by renneting extraction, on the other hand, exhibited bigger pores and was composed of a discontinuous, loose network with broad holes and a diffuse structure (Figure 2b). The result was consistent with the one demonstrated by XRD analysis, which suggested that the biological treatment effectively altered the structure of the casein proteins' surface. Morphological alterations might have had a major impact on the casein's functional characteristics, such that the proteins produced by rennet had greater emulsifying stability, as shown later in (Figure 7).
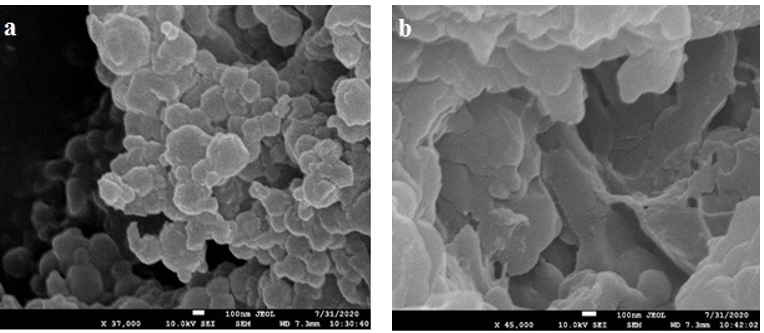
Figure 2. SEM images of camel caseins obtained by gelation with (a) HCL or (b) rennet
X-ray photoelectron spectroscopy
The XPS technique is a well-established method for determining the basic composition of the surface layer (5-10 nm depth) of the biomolecule under investigation (Nikolova et al., 2015). XPS survey and high-resolution elemental spectra were used to examine the distribution of relative atomic elements content of acid and rennet camel caseins surfaces (Figure 3). Indeed, the XPS survey contained several peaks, including the O1s, C1s, and N1s. At varied binding energies, these typical peaks may be deconvoluted into detailed sub-peaks. The resultant sub-peaks showed several well-known chemical functions, such as (C–C (H), C–O, O–C=O, etc.) (Gerin et al., 1995). According to the biochemical compound model, the C, N, and O peaks were deconvoluted into sub-peaks that matched to the different functions of the camel casein proteins that compose the caseins surface, as shown in (Figure 4). The C1s peak was mainly divided into four peaks, one for each of the functions C–(O, N), C–(C, H), C=O, and O–C=O. The O1s peak was separated into three peaks, each of which was assigned to the O–C, O=C, and H 2O, or O–C=O functions. The C–NH and C–NH 3 + functions were decomposed from the N1s peak.
The casein powders' surfaces were mostly made up of carbon, oxygen, and nitrogen (Nawaz et al., 2016). Nevertheless, minerals like phosphorus and sulfur were discovered on the surface too. The survey spectra contained very tiny peaks of P, S, and Ca 2p, and the addition of these three elements was determined to be less than 2 % for the two powders (Table 2). As a result, the contents of S and P were neglected in this study. However, the Ca 2p is one of the most important differences between acid and rennet caseins (Figure 3). Rennet camel casein micelles are phosphoproteins containing phosphorus (P) and calcium (Ca) while the acid camel caseins micelles contained less phosphorus and no calcium on the surface (Table 2). This difference can be explained by the well-known fact that at pH 4.3 all of the remaining calcium ions and colloidal calcium phosphate are dissociated and solubilized from casein micelles (Jacob et al., 2011; Li and Zhao, 2019). Ca 2+, on the other hand, is crucial in the production of rennet gels (Lucey et al., 1996). Therefore, acid and rennet camel caseins may be differentiating according to their mineral composition.
Table 2. Elemental composition of Acid coagulated casein and Rennet coagulated casein powders

Table 2 shows the results of quantitative chemical analysis of the surface of casein protein powders obtained by rennet and acid aggregation. The proportion of peak area of C1s, O1s, and N1s in casein powder surfaces closely mirrored the percentage of C1s, O1s, and N1s concentration. The C1s, N1s, and O1s percentages of casein proteins produced using the two coagulation techniques differed significantly. The carbon and oxygen proportions of the surface of casein protein powders produced by acid aggregation were greater than those obtained by rennet coagulation, but the nitrogen percentage was lower. Li and Zhao (2019) revealed that rennet gelation may change the carbon, oxygen, and nitrogen percentages on the surface of casein micelles, which was consistent with the findings of this study. The atomic ratios provided in (Table 2) are relative to atomic C and may be used to precisely compare concentration changes for each surface. Similar O/C ratios were reported for camel casein coagulated with HCL and rennet, as shown in (Table 2): 0.28 and 0.30, respectively. The somewhat higher value of the latter showed that the surfaces of the chymosin-treated camel casein powders were oxidized during preparation and storage. Furthermore, the fact that additional amino groups of proteins, such as tyrosine residues, were exposed on the surface of precipitates produced via renneting might contribute to an increase in O atom concentration (Zhao et al., 2011). It was intriguing to notice that camel caseins produced by rennet coagulation had a high N/C percentage (Table 2). The difference in N/C proportions between the two aggregation techniques for camel casein surfaces was considerable. These findings revealed that fewer amino groups were available on the surface of acid-coagulated camel casein (Zhao et al., 2011). The fractions of N/C and O/C on the surface of biologically coagulated camel caseins were superior, showing that the major proteolysis sites in the chymosin system were significant during casein coagulation (Nouri et al., 2012).
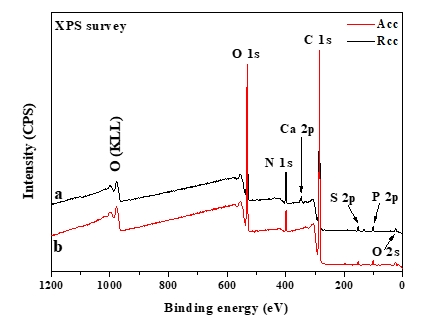
Figure 3. Survey-scan XPS of (a) rennet camel caseins, and (b) acid camel caseins
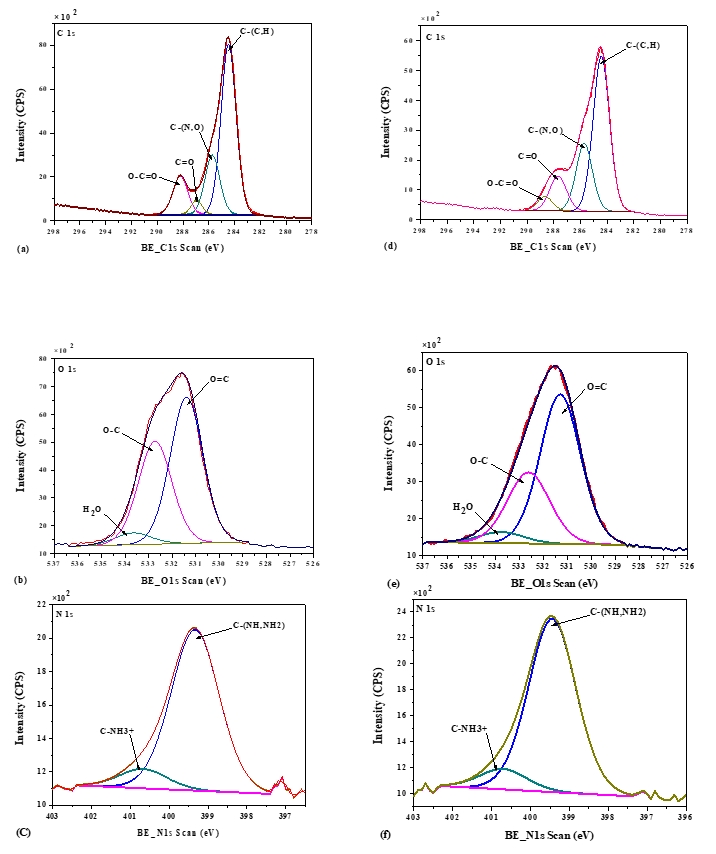
Figure 4. X-photoelectron spectra of C1s, O1s, and N1s of acid camel casein (a, b, c), and rennet camel caseins (d, e, f)
Emulsion stability index
The emulsion stability index (ESI) estimates the relative stability of an emulsion after a given period (Pearce and Kinsella, 1978). ESI values of emulsions prepared with acid and rennet camel casein at 2 % and at oil volume fraction (ɸ = 0.4) versus time curve are showed in Figure 5, while Figure 6 illustrates photonic microscopy pictures of the completed emulsions. The obtained results revealed that both of the stability curves are diminishing with time. However, the emulsion prepared with rennet camel casein (Rcc) showed higher stability values in comparison to the emulsion stabilized with acid camel casein (Acc). Furthermore, the emulsion formed with 2 % of Rcc was characterized by variable stability values: moderate decrease (97 %) at first 60 min, stabilization (89 %) starting from 90 min of storage, against the emulsion prepared with 2 % of Acc, which was characterized by a strong decrease at 60 min (88 %) and stabilization beginning from 90 min (83 %) and another decrease after 150 min. Such behaviour can be explained by the fact that acid camel casein has a greater molecular weight and less flexibility than other caseins (Rcc). As a result, it does not adsorb and unfurl at the interface as quickly as surfactants do. In addition, these results show that the more emulsifiers (camel caseins) are flexible, the more surface area can be occupied, by stabilizing and coating a larger number of smaller oil droplets. The fat globules diameter test for emulsions at an equal concentration (2 %) of the two types of camel casein confirms these results, as demonstrated in Figures 6 and 7. For all emulsions, the mean diameter of fat globules was growing up over time for both emulsifier types. Therefore, the differences between the globule diameters of Acc 2 % and Rcc 2 % emulsions, were very significant (p<0.005).
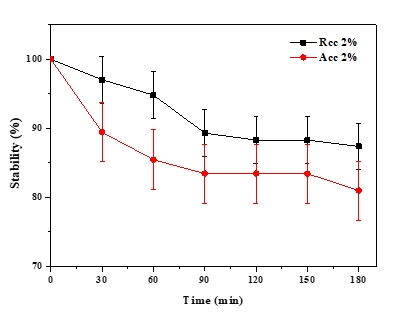
Figure 5. Emulsion’s stability over time of (Acc) and (Rcc)
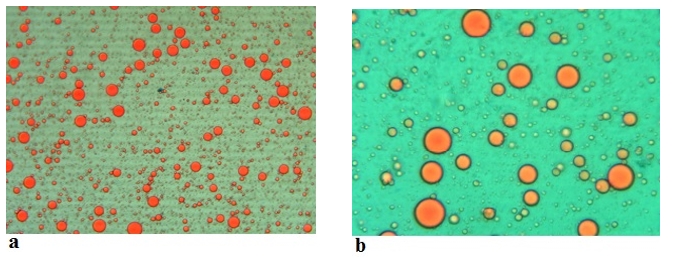
Figure 6. Microscopic images (Mg. ×100) of the emulsions stabilized with acid camel sodium caseinates at (a) 0 min and at (b) 180 min
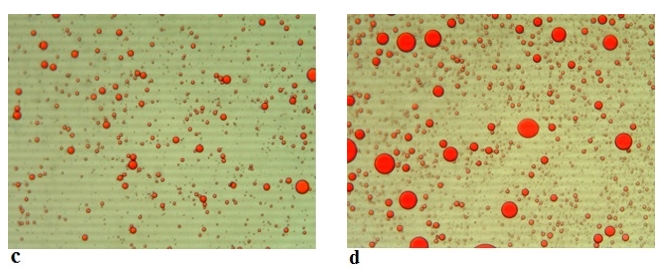
Figure 7. Microscopic images (Mg. ×100) of the emulsions stabilized with rennet camel sodium caseinates at (c) 0 min and at (d) 180 min
Conclusion
In summary, the spectroscopy characterization and the emulsifying potential of acid and rennet camel milk caseins were studied in this paper. It could be confirmed that acid coagulation modifies the surface composition of camel casein proteins as revealed by XPS. Camel caseins produced via rennet-induced coagulation, on the other hand, exhibited a substantial change in surface composition, with a diminution in carbon and oxygen atom concentrations and a rise in nitrogen atom concentration at the surface of proteins. The N/C ratios of camel casein precipitates showed substantial variation, which was linked to chymosin's particular activity. On the other hand, the two examined camel casein precipitates had comparable O/C ratios. As a result of rennet activity, additional amino groups on the surfaces of camel casein precipitates were created by bond rupture, resulting in a rise in the N atomic percentage of the camel casein surfaces. Furthermore, significant variances in XRD peaks and SEM micrographs that related to the rennet action were also found. The emulsifying behaviour of camel casein obtained by rennet-induced coagulation was higher than that obtained by acid aggregation. This indicated that the rennet-induced gelation can enhance the emulsifying properties of casein, thereby paving the way for the use of rennet camel caseins as an emulsifying agent in different food formulations.
Acknowledgements
The assistance provided by Dr. Yasmine Khane (Université de Ghardaia) was greatly appreciated, and we thank her for her comments that greatly improved the manuscript.
Primjena spektroskopskih metoda XRD, XPS i SEM u karakterizaciji kazeina devinog mlijeka koaguliranog djelovanjem kiselina i enzima te u određivanju utjecaja koagulacije na njegovu sposobnost emulgiranja
Sažetak
Kazein, glavni protein u mlijeku, održiv je izvor prirodnih emulgatora koji se često koriste u prehrambenim emulzijama. U ovom su istraživanju korištene metode rentgenske difrakcije (XRD), rentgenske fotoelektronske spektroskopije (XPS) i skenirajuće elektronske mikroskopije (SEM) u svrhu analize i karakterizacije kazeina u prahu dobivenog kiselinskom odnosno enzimskom koagulacijom devinog mlijeka. Spomenute instrumentalne metode rezultirale su opsežnim informacijama o kristalnoj strukturi, površinskom sastavu, odnosno sekundarnoj mikrostrukturi kazeina devinog mlijeka. Iz toga su proizišle su nove spoznaje o morfologiji i sastavu površine, a koje su potrebne za bolje razumijevanje funkcionalnih karakteristika poput sposobnosti emulgiranja i stabilnosti emulzije tijekom vremena i veličini kapljica ulja. Uzimajući u obzir mjerenja pomoću rentgenske difrakcije (XPS), udjeli atoma kisika i ugljika na površini kazeina koaguliranog djelovanjem kiseline bili su veći u usporedbi s kazeinom koji je dobiven enzimskom koagulacijom, dok je udio atoma dušika bio niži. Omjeri kisika i ugljika (O/C) na površini kazeina iz devinog mlijeka dobivenog na dva različita načina koagulacije bili su usporedivi. Međutim, metoda enzimske koagulacije rezultirala je visokim omjerom dušika i ugljika(N/C). Dobiveni rezultati impliciraju da bi enzimska koagulacija mogla utjecati na omjer ugljika, kisika i dušika na površini kazeina devinog mlijeka. Nadalje, rezultati dobiveni SEM i XRD analizom ukazuju da koagulacija kiselinom utječe na morfologiju micele kazeina, što rezultira s više β-nabranih ploča i glatkih struktura. S druge strane, enzimski koagulirani kazein devinog mlijeka pokazuje porozniju koloidnu površinu. Također je utvrđena i korelacija između sastava površine kazeina i sposobnosti emulgiranja kazeinskih prahova, dok su veličina kapljica i stabilnost emulzija značajno poboljšane enzimskom metodom koagulacije.
Ključne riječi: kazein devinog mlijeka; enzimska koagulacija; kiselinska koagulacija; karakterizacija; stabilnost emulzije