Introduction
Cheese is a highly dynamic product undergoing significant transformations throughout ripening. It refers to various fermented food products made from milk diverse in flavour and form, worldwide. The production of cheese typically involves several common technological steps, including milk cooling, heat treatment, milk standardization, the addition of dairy cultures and rennet, curd cutting, curd drying, molding, pressing, salting, ripening, packaging, storage and transportation.
Each stage in cheese production plays a vital role in achieving the desired sensory properties. As a result, cheese production technology aims to establish specific parameters that contribute to the desired characteristics, such as taste, aroma, texture, solubility and stretchability. Additionally, it aims to develop a standardized protocol for cheese production and ripening to ensure consistent quality of the final product.
Traditionally cheeses have been recognized for their diverse microbiota and complicated metabolic processes that influence their unique flavour. As a result, bacteria play an essential role in creating cheese flavour (Zheng et al., 2021). Depending on the type of cheese and the method of production, various enzymes such as the coagulant (usually chymosin), endogenous milk enzyme (plasmin), enzymes of starter (such as lactic acid bacteria - LAB) and of non-starter microflora (non-starter lactic acid bacteria - NSLAB), as well as enzymes of the added yeast culture are used in the cheese production process (Fox et al., 2017). Complex chemical-biochemical changes in the breakdown of organic compounds to simpler ones via primary and secondary metabolism occur during cheese ripening (McSweeney and Sousa, 2000). Principal modifications consist of glycolysis, lipolysis, and proteolysis and the metabolism of residual lactose, of lactate and citrate, lipolysis and metabolism of fatty acids and proteolysis and amino acid catabolism. Subsequently secondary biochemical reactions involving the metabolism of fatty acids and amino acids, which directly contribute to the creation of several volatile organic compounds linked to cheese flavour (Ardö et al., 2017). Primary metabolism, which comprises carbohydrate breakdown, protein hydrolysis, and fat breakdown determines the base flavour of the cheese. Secondary metabolism in cheese production is responsible for creating distinct aromas in different types of cheese. It involves various biochemical processes such as converting amino acids through decarboxylation, transamination, deamination, desulfurization, beta-oxidation of fatty acids, and esterification (Marilley and Casey, 2004).
Additionally, yeast plays a significant role in some specific types of cheese by efficiently producing several secondary metabolites (such as esters, higher alcohols, carbonyls, fatty acid derivatives and sulphur compounds) contributing to cheese quality and production of complex bouquets of aroma compounds (Dzialo et al., 2017). In the case of most cheese varieties, the initial breakdown of casein is primarily carried out by chymosin, with a lesser contribution from plasmin and aspartate proteinase (cathepsin D). This process forms large and medium-sized peptides, which are further broken down by the enzymes in the starter and non-starter cultures latter in the cheese production process (McSweeney, 2017).
Metabolism of lactose, lactate, and citrate
Lactose is the primary carbohydrate found in the milk of nearly all mammals. When metabolized, it produces L-lactate, DL-lactate, or a mixture of both, which is crucial for developing flavour in all types of cheese. However, certain bacteria, such as Leuconostoc spp., can generate additional by-products like ethanol (Vedamuthu, 1994). In the case of Cheddar, Dutch, and similar cheeses, the non-starter microflora converts the L-lactate produced by the starter bacteria Lactococcus lactis and/or Lactococcus cremoris into DL-lactate (McSweeney, 2017). However, an excessive amount of DL-lactate can negatively impact the sensory characteristics of the cheese. Pyruvate, an intermediate product of lactose metabolism, serves as a precursor for the production of various aromatic compounds with short carbon chains, including acetate, acetoin, diacetyl, ethanol, and acetaldehyde (Melchiorsen et al., 2002).
In Swiss cheese, Propionibacterium spp. metabolizes lactate during ripening to produce propionate, acetate, carbon dioxide, and water. Notably, carbon dioxide forms the characteristic "cheese eyes" (Duru et al., 2018). Acetate is an important component contributing to the aroma of various cheeses. In mature cheeses like Camembert and Brie, the lactic acid in the surface layer undergoes metabolic breakdown by molds and yeasts, producing water and oxygen. This process leads to an increase in pH, which in turn leads to migration of Ca to the cheese surface and the considerable softening which characterizes these varieties (Lucey and Fox, 1993). The conversion of lactose into lactic acid is a critical step in cheese production, facilitated by carefully selected cultures of LAB added to the milk shortly before adding rennet. These cultures, known as starters, initiate acid production and are often referred to as lactic cultures because they primarily produce lactic acid. However, their enzyme systems also play a crucial role in developing cheese flavour during ripening.
During the cheese production process, lactic acid production is important for three functions. Firstly, it stimulates the activity of the coagulant. Secondly, it aids in expelling whey from the cheese mass, reducing thereby the moisture. Lastly, it helps preventing the growth of undesirable bacteria in cheese (Fox et al., 2017). The concentration of lactose in cheese can decrease through washing or whey replacement. In such cases, any remaining lactose in the cheese curd is rapidly metabolized, increasing the pH.
Consequently, cheeses with low lactose concentrations exhibit a fresh and mild taste, while those with high lactose concentrations can have a strong and sharp taste due to the low pH. In milk, citrate primarily exists as ionized salts at concentrations ≤1.8 g/L, most of which is lost in the whey during cheese production. This is because nearly 94 % of citrate is in the soluble phase of milk (McSweeney, 2017). Streptococcus thermophilus or thermophilic lactobacilli do not metabolize citrate, but specific mesophilic lactobacilli in the non-starter microflora can. Citrate-positive lactococci (Cit+) and Leuconostoc spp. can produce essential aromas such as acetate, diacetyl, acetoin, butanediol, and carbon dioxide from citrate. Diacetyl, in particular, is a vital aroma compound that undergoes conversion to acetoin, 2,3-butanediol, and 2-butanone in certain cheese types like Dutch cheeses, quark cheese, and cottage cheese (Dimos et al., 1996). Citrate metabolism plays a significant role in Dutch cheeses, where the production of carbon dioxide is responsible for the formation of cheese eyes. Additionally, citrate could be the substrate for Cit+ starter cultures and non-starter bacteria (Fox et al., 2017).
Proteolysis and catabolism of amino acids
Protein hydrolysis is a crucial biochemical process that significantly influences cheese flavour formation and the release of flavour compounds during cheese ripening (Gan et al., 2016). Proteases in cheese degrade proteins, producing peptides and free amino acids (FAA), which act as precursors for various aromatic components in cheese. Casein hydrolysis is particularly important in the flavour development of hard and semi-hard cheeses. Microbial proteases and peptidases catalyse the breakdown of polypeptide chains, generating free amino acids that contribute to aroma formation during cheese production and ripening (McSweeney, 2017). Changes in cheeses resulting from proteolytic degradation of proteins have been intensively studied. Monitoring of proteolytic changes and peptide degradation provides an insight into the formation of bitter taste in cheese (Urbach, 1995; Fox et al., 1999). Enzymatic hydrolysis of proteins results in peptides of smaller molecular weight, some of which, predominantly hydrophobic, have a bitter taste. In the case of incomplete hydrolysis of such peptides, they accumulate and the cheese is thus perceived as bitter. Non-starter lactic acid bacteria (NSLAB), starter and rennet enzymes play an important role in the creation of bitter peptides. The decomposition of αs1-casein (αs1-CN) and β-CN by rennet or microbial peptidases results in a formation of certain bitter peptides, which can be desirable in mature cheeses. By increasing the concentration of bitter peptides above the permitted limit, the cheese becomes bitter (Mikulec et al., 2010).
The catabolism of free amino acids plays a vital role in producing aldehydes, alcohols, carboxylic acids, amines, and sulphur compounds. Amino acid aminotransferase catalyses the transamination reaction, converting aromatic amino acids, branched-chain amino acids, and aspartic acid into α-ketoacids. These compounds are metabolized into branched and aromatic aldehydes, acyl-CoA, hydroxy acids, and methanethiol (Ganesan and Weimer, 2017). Transamination of valine, isoleucine, and leucine leads to the production of 2-methylpropanal, 2-methylbutanal, and 3-methylbutanal, while transamination involving aspartic acid results in the release of oxaloacetate, which is then converted into acetoin, diacetyl, or 2,3-butanediol (Ardö, 2006).
Aromatic aldehydes primarily arise from α-ketoacids, derived from the spontaneous oxidation of tryptophan and phenylalanine, releasing benzaldehyde. Conditions favouring a predisposition for redox reactions, influenced by temperature, are necessary since temperature increases accelerates catabolism (Klačanová et al., 2010). Further metabolic pathways of aldehydes include conversion into alcohols or oxidation into carboxylic acids by several dehydrogenases (Ganesan and Weimer, 2017).
Moulds and yeasts play a significant role in the biosynthesis of primary and aromatic alcohols, leading to the subsequent release of corresponding carboxylic acids. Studies on Geotrichum candidum and yeasts isolated from Camembert have provided insights into the mechanisms involved in alcohol and carboxylic acid production through the metabolism of free amino acids (Yvon and Rijnen, 2001).
Uncontrolled proteolysis in cheeses during ripening, caused by unfavourable environmental conditions and extended duration, can result in high levels of free amino acids. Biogenic amines are produced through the microbial decarboxylation of amino acids. Biogenic amines, such as histamine, tyramine, cadaverine, and putrescine, are synthesized from histidine, tyrosine, lysine, and ornithine, respectively, and are associated with potential negative effects on consumer health. The consumption of cheese containing high levels of biogenic amines such as histamine or tyramine can trigger symptoms in susceptible individuals such as headaches, migraines and heart palpitations (Schirone et al., 2018).
Another significant aspect of free amino acid catabolism involves elimination reactions, where the enzyme lyase cleaves the side chain of amino acids.
The majority of volatile sulphur compounds (VSCs) originate mainly from the breakdown of L-methionine by the microorganisms present in cheese. This process results in the production of methanethiol (MTL), which is later transformed into various sulphur-containing substances like dimethyl disulphide (DMDS), dimethyl trisulphide (DMTS), and S-methyl thioesters. These compounds have a low odour perception, notably impacting cheese flavour (Curioni and Bosset, 2002).
Lipolysis and catabolism of free fatty acids
The process of lipolysis significantly affects the flavour of cheese (Curioni and Bosset et al., 2002). Various sources contribute lipases to cheese, including milk, rennet, starter cultures, added cultures, NSLAB, and external enzymes. These lipases catalyze the breakdown of triglycerides, resulting in the production of medium-chain (up to 10 carbon atoms) and long- medium and long-chain fatty acids and partial glycerides, as well as glycerol (Collins et al., 2003). Among all lipases, lipoprotein lipase plays a crucial role in flavour development in cheeses made from raw milk but has a limited impact on the taste of cheese made from pasteurized milk (Sert et al., 2014). The presence of fatty acids and pH values directly affects the flavour characteristics of cheese, and these factors influence each other. Cheeses with higher pH values demonstrate reduced liberation of fatty acids from compounds that play a substantial role in flavour enhancement. Instead, these fatty acids undergo a conversion into non-volatile salts, potentially resulting in unwanted "soapy" aromas. In contrast, when the pH is low, fatty acids are present in a volatile form within the milk matrix, and an excessive increase in their concentration tends to result in a rancid taste (Ardö et al., 2017). Lipolytic enzymes found in LAB can hydrolyse the substrate, producing free fatty acids (FFA), triacylglycerides, diacylglycerides, and monoacylglycerides. The esterase enzyme present in LAB specifically acts on <C18-monoacylglycerides, with a particular sensitivity towards C8-monoacylglycerides, but it does not have any effect on >C6-diacylglycerides (Holland et al., 2005). Propionic acid bacteria (PAB) exhibit a much greater ability, ranging from 10 to 100 times, to break down fats compared to LAB. In Swiss cheese, propionic acid bacteria play a crucial role in converting lactose into acetate, the production of characteristic flavour through carbon dioxide, and the generation of free acids (Schwenninger et al., 2011). The process of lipolysis in cheese primarily involves the breaking of ester bonds between triglycerides and fatty acids by lipase, resulting in the formation of monoacylglycerides, diacylglycerides, and free fatty acids (Deeth and Touch, 2000). Fatty acids significantly affect the taste and flavour of cheese. During the fermentation and ripening of cheese, milk fat is broken down, forming medium-chain and short-chain fatty acids (C>4). These compounds contribute to the characteristic aroma of cheese and serve as important indicators of cheese maturity. This process can result in an unpleasant odor associated with rancidity, observed in spoiled Gouda, Cheddar, and Swiss cheeses (Forde and Fitzgerald, 2000). Short-chain fatty acids contribute to distinct and robust flavours in cheese, some of which serve as flavour precursors and undergo conversion into other aromatic compounds, including lactones and alcohols (Temizkan et al., 2015). Primary lactones found in cheese are γ-lactones and δ-lactones, which consist of five and six-membered rings and possess intense aromas. Esters in cheese are formed through an esterification reaction between the short-chain fatty acids and the medium to long-chain fatty acids, which result from the breakdown of milk fat, as well as primary and secondary alcohols produced during lactose fermentation or amino acid metabolism in the fermentation process (Fox et al., 2017). These esters contribute to sweet, fruity, and floral aromas in cheese. However, excessive ethyl butyrate and ethyl caproate can produce an overly strong fruity aroma (Castada et al., 2019). Additionally, the reaction of free fatty acids with sulfhydryl groups leads to the formation of thioesters such as S-methylthioacetate, thioethyl-2-methylpropanoate, and S-methylthiobutyrate, which impart a garlicky, sulphurous, or eggy flavour (Iwasawa et al., 2014). Lastly, β-oxidation and subsequent decarboxylation of free fatty acids, particularly in certain cheeses like blue cheese, resulting in the production of methylketones or alkan-2-ones, notably heptanone and nonanone (McSweeney et al., 2004). Lipolysis and the catabolism of fatty acids represent the predominant biochemical processes that occur during cheese ripening (Collins et al., 2003). Carboxylic acids, ranging from C2 (acetic acid) to C10 or C12 (decanoic or dodecanoic acid), are typically the most prevalent group of volatile organic compounds in cheese. They are followed by various other compounds, including aldehydes, lactones, ketones, alcohols, esters, and phenolic compounds (Faccia et al., 2018).
Volatile organic compounds contribute to the flavour of the cheese
Analysing the distribution of volatile organic compounds in cheese is essential for understanding the factors contributing to its aroma and flavour. It helps cheese manufacturers and researchers to determine the key volatile compounds responsible for specific cheese varieties and their sensory characteristics. This knowledge can be used to control and optimize the cheese-making process, develop new flavours, and ensure consistent quality and flavour profiles in cheese production. Table 1 shows the composition of volatile organic compounds (acids, alcohols, aldehydes, ketones, esters, lactones) responsible for the flavour of some types of cheese. The following sections describe the individual groups and their influence on the final cheese product in more detail.
Table 1. Distribution of volatile organic compounds (%) in different types of cheese
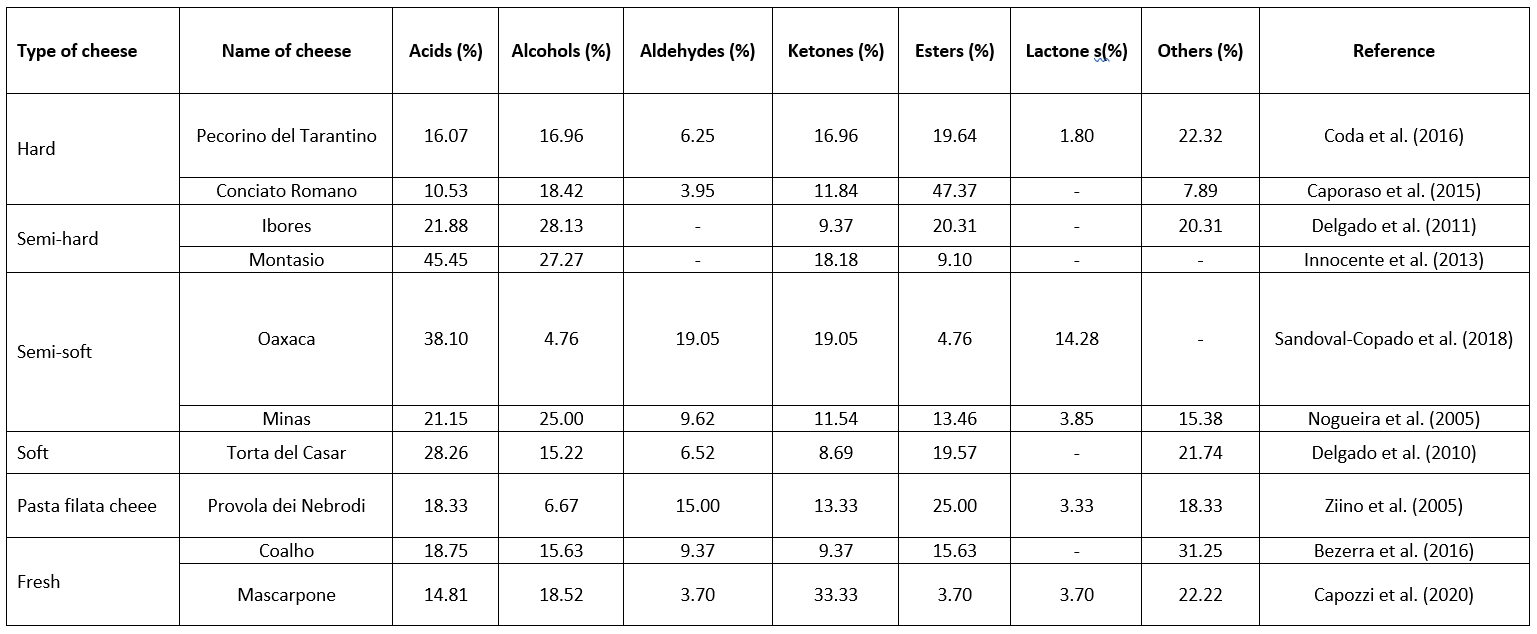
Acids
Fatty acids are important, even dominant, components of the flavour of many types of cheese. Fatty acids are aroma compounds and serve as precursors of methyl ketones, alcohols, aldehydes, lactones, and esters. Free fatty acids containing four or more carbon atoms can arise during cheese ripening from milk fat lipolysis (Urbach, 1993). Lipolysis can result from the action of natural milk lipases in cheese produced from fresh milk or by the action of microbial lipases. LAB present in starter cultures usually have a mild or weak lipolytic effect, which means they have limited capability to break down fats or lipids. This characteristic can influence the flavour and texture of the final products, by contributing to the release of fatty acids during ripening. However, most fatty acids, with four to twenty carbon atoms, come from the lipolysis of triglycerides. Shorter fatty acids typically contain less than 6 carbon atoms and can undergo oxidation to ketones, esters, and aldehydes. These compounds like acetic acid (2 carbons), propionic acid (3 carbons), and butyric acid (4 carbons) contribute to the aroma and flavor profiles of various cheeses (Molimard and Spinnler, 1996). It is also important to note that the pH value of cheese affects the flavour perception of volatile fatty acids. Only the free, protonated form of fatty acids has an aromatic effect and contributes to cheese flavour (Brennan et al., 2002). These are mainly acids dissolved in the fat phase of the cheese. Long-chain fatty acids (>12 carbon atoms) play a smaller role in taste due to relatively high perception thresholds. Short and medium fatty acids, with an even number of carbon atoms (C4-C12), have much lower perception thresholds, each with a characteristic note. Acetic acid is the main organic acid in Cheddar (ripened, regular, and low fat), Gruyere, Roncal, and Emmental cheese varieties (Preininger et al., 1996). It could be synthesized by catabolism of lactose, citrate, and free fatty acids and can be obtained from amino acid metabolism. It is associated with a sharp, vinegary, and sour aroma (McSweeney and Sousa, 2000). Butyric acid has a rotten cheese smell and plays an important role in the flavour of many cheeses; such as Gruyere, Pecorino, Ragusan, and Roncal cheese (Fox et al., 2017). However, large amounts of this volatile acid, are undesirable. Butanoic and hexanoic acids are considered the main causes of strong and, in some cases, unpleasant odours, which are described as cheesy, fierce, and "sweaty", and their tendency to increase in concentration during the ripening of hard cheeses has been widely observed and described (Zabaleta et al., 2016). The general increase in the concentration of free fatty acid, such as butanoic and hexanoic acids, is usually explained by the extent of autolysis of the starter cultures during cheese ripening that leads to the release of enzymes, especially lipases, which promote lipolysis by cleaving the ester bond between the fatty acid and glycerol triacylglycerol (Collins et al., 2003). Octanoic acid is considered the main "goat" component in dairy products and is said to have a waxy odour that contributes strongly to the flavour of hard goat cheese. Also, decanoic and dodecanoic acids undoubtedly affect the taste of hard cheeses, and their increase is generally associated with a soapy taste (Woo et al., 1984). In mature Cheddar, other straight-chain fatty acids, such as hexanoic (caproic), decanoic (caprylic), and dodecanoic (lauric) acid, also contribute significantly to its aroma. Hexanoic acid is also a characteristic component of the flavour of Grana Padana and Roncal cheese, while octanoic and decanoic acids are known as the main odour compounds for these types of cheese. From a sensory point of view, nonanoic acid significantly contributes to the goat cheese flavour (Le Quéré et al., 2022). Furthermore, 3-methylbutanoic acid (isovaleric acid), produced from amino acid catabolism and with a rotten, cheesy, and foul smell, contributes to the aroma of very mature cheese (Yvon and Rijnen, 2001). Phenylacetic acid, also derived from the metabolism of amino acids, with notes of honey, contributes significantly to the aroma profile of Gruyere cheese (Rychlik and Bosset, 2001).
Aldehydes
Aldehydes are potent aromatic compounds commonly linked to food flavour defects known as oxidative spoilage (McSweeney and Sousa, 2000). Aldehydes such as hexanal (green-like), heptanal (green-like), pentanal (fermented-like), and 3-methyl butanal (fruity) could be detected at different stages of ripening in the cheese. The first three as straight-chain aldehydes in cheese can be produced by β-oxidation of fatty acids, while 3-methyl butanal as a branched aldehyde can be generated by amino acid metabolism (Kilcawley, 2017). The release of linear chain aldehydes is known for its pleasant flavour characteristics (Bertuzzi et al., 2018).
Ketones and alcohols
Ketones and alcohols are formed mainly through biochemical processes involving the breakdown of triglycerides and the oxidation of saturated fatty acids. Production of α-ketoacids from amino acids through amino acid catabolism, which undergo decarboxylation to form ketones that can subsequently be reduced to alcohols (Urbach, 1993). A specific range of methyl ketones contribute to the distinctive flavour of traditional blue cheeses. Methyl ketones are produced by Penicillium roqueforti and Penicillium camemberti from the fatty acids in the cheese by the first four steps of the β-oxidation pathaway (Collins et al., 2003). Methyl ketones have been reported to have low perception thresholds in water, ranging from 0.09 mg/100 g for 2-heptanone to 4.09 to 50.0 mg/100 g for 2-propanone (Bertuzzi et al., 2018).
Esters
Esters represent a group of organic compounds indirectly metabolizing free fatty acids (Collins et al., 2003). Many of these compounds have a low perception threshold and are widely associated with pleasant aromas characterized by sweet, fruity, and floral tones. They are formed by a one-step transesterification reaction between ethanol and partial glycerides (Holland et al., 2005). The specific types and concentration of esters can vary depending on the type of cheese, the starter cultures used, as the ripening condition. In addition, esters are valued for their role in reducing the bitterness and pungency of cheeses, often caused by the high content of biogenic amines and free fatty acids. It is known that esters such as ethyl acetate can impart fruity notes, while ethyl butanoate can add a pineapple-like aroma. These compounds are often present in small amounts but play an important role in the overall sensory experience of cheese (Liu et al., 2004).
Lactones
The main precursors of lactones are hydroxylated free fatty acids, which are incorporated into milk fat triglycerides and released due to enzymatic lipolytic mechanisms or heating processes. Hydroxylated free fatty acids can also be produced by the action of enzymes lipoxygenases or hydratases of microbial origin within the catabolism of unsaturated fatty acids. The one-step transesterification reaction effectively synthesizes lactones from hydroxylated free fatty acids (Bertuzzi et al., 2018). These mechanisms strongly and positively affect the taste of cheese since lactones are associated with extremely pronounced fruity notes, although it was found that they also contribute to the "buttery" characteristic of cheese (Castada et al., 2019). The synthesis of lactones leads to the release of α- and β-lactones that are reported to be highly reactive and unstable, while γ- and δ-lactones are stable and have been identified in several dairy products (Ianni et al., 2020). In Cheddar cheese, the concentration of lactones increases rapidly in the early stages of ripening, reaching levels well above the threshold of taste perception. δ -Octalactone was reported as the most abundant lactone in Parmigiano Reggiano cheese, while δ-decalactone, ɣ-decalactone, δ-dodecalactone, and ɣ-dodecalactone were detected in several French blue cheeses (Thierry et al., 2017).
Phenolic compounds
Phenolic compounds can be found in milk and dairy items due to various factors such as their origin from pastures, catabolism of proteins by bacteria, deliberate or accidental addition, inclusion during processing, contamination with sanitising agents, or environmental contamination (O’Connel and Fox, 2001). Regardless of whether these compounds are present due to direct or indirect actions, intentional or unintentional, they are likely to significantly impact the sensory characteristics of milk and dairy products. According to O'Connell and Fox (2001), most of the volatile phenolic compounds identified in milk and milk products are closely associated with the diet of ruminant animals, although some may result from the breakdown of amino fatty acids, with tyrosine being the preferred precursor. Phenolic compounds produced through amino acid catabolism include phenol, 4-ethylphenol and p-cresol. While the complete understanding of the role of natural phenolic compounds in affecting the sensory characteristics of milk and dairy items is not yet fully clarified, it is suggested that in small quantities, these naturally occurring compounds might add pleasant flavours like sweetness, smokiness, or caramel notes to various dairy products. However, in larger amounts, they could lead to undesirable sensory qualities such as sharpness, medicinal notes, or a barnyard-like aroma (O’Connell and Fox, 2001). It's important to acknowledge that the influence of natural phenolic compounds on the sensory attributes of dairy goods is usually quite subtle. When present at concentrations below the perception threshold, they contribute to the delicate balance of flavour components. Adding of external phenolic compounds (PCs) as extracts, it seems that the heightened chemical reactivity of flavonoids and tannins renders them more appropriate choices as functional ingredients, serving both health and product quality purposes in many instances.
Sulphur compounds
Sulphur compounds in cheese can originate from methionine degradation and result from the cleavage of the bond between carbon and sulphur by the enzyme methionine-demethylase (Yvon and Rijnen, 2001). They are present in cheeses ripening with moulds on the surface, and low molecular weight compounds such as dimethylsulfide, dimethyldisulfide, and dimethyltrisulfide are considered important contributors to cheese flavour (Curioni and Bosset, 2002). Kilcawley (2017) states that dimethyltrisulfide is generally associated with the odorous notes of vegetables, sulphur, garlic, and rot, while dimethyldisulfide is related to green, sour, and "garlic" notes. The most common S-compound in cheese is methional (3-methylthiopropanal). This volatile compound has a characteristic smell of boiled potatoes and a sharp, intense smell and plays an important role in the aroma profile of various types of cheese, such as Camembert, Cheddar, Emmental, goat cheese, Gorgonzola, Grana Padano, Gruyere, Pecorino and mature Ragusan (Curioni and Bosset, 2002). Methanethiol is another important sulphur-containing compound. It originates from methionine and can be a precursor for further sulphur compounds such as dimethyldisulfide and dimethyltrisulfide through oxidative reactions. Many microorganisms can produce methane thiol from methionine, especially P. camemberti, G. candidum, and B. linens (Jollivet et al., 1994). Among these microorganisms, coryneform bacteria, especially B. linens, are crucial for producing sulphur compounds in mould-ripened cheeses (McSweeney and Sousa, 2000).
Terpenes
Terpenes are a large and diverse group of organic compounds found in different types of plants. The basic structure of terpenes is the isoprene unit or 2-methyl-1,3-butadiene (C 5H 8). Based on the number of isoprene units in the molecule, terpenes can be classified as monoterpenes (C10), sesquiterpenes (C15), di-terpenes (C20), sister-terpenes (C25), and triterpenes (C30). Monoterpenes are volatile compounds and essential oils' basic components. Diterpenes have a low volatility and a high boiling point and are considered resins. Triterpenes are often solid compounds with a high melting point (Setzer et al., 2006). Terpenes are secondary metabolites produced by higher plants, algae, and fungi. These compounds can have different biological roles (i.e., protection, attraction) such as antimicrobial, antifungal, antiparasitic, antiviral, anti-allergic, anti-inflammatory, and chemotherapeutic properties. Finally, their use as flavours and fragrances in food and cosmetics has been widely documented (Ajikumar et al., 2008). They come from the plants that make up the pasture mixture, then transferred to the milk of grazing animals and finally to cheese (Mariaca et al., 1997). The analysis of terpenes in natural products is very challenging and demanding. Terpenes show great diversity not only in their basic carbon skeletons but also in their functional groups. Belviso et al. (2011) proved that isolated LAB strains ( Lactococcus lactis, Lactococcus cremoris, Streptococcus thermophilus, Streptococcus macedonicus, Lacticaseilactobacillus paracasei) from Toma Piemontese cheese (which contains the PDO label) could degrade, modify and biosynthesize monoterpenes. Among LAB isolated from cheese, only Lactobacillus acidophilus has been found to have terpene biosynthesis activity (Belviso et al., 2011). Terpene composition is generally characterized by a wide concentration range of analytes in the sample (from ppb - parts per trillion to percentage levels). In addition, many terpenoids are isomeric; they can be geometric, positional, and optical isomers with the same molecular formula but different structures.
Analysis of aroma compounds in cheese
The current approach to studying aromatic compounds involves multiple stages, with the primary focus being identifying the specific compounds responsible for the distinct flavour of the cheese. The aromatic elements in cheese primarily arise from the breakdown of fats and proteins, which occur during the ripening process and play a vital role in generating the aroma. These aroma molecules typically exhibit hydrophobic or lipophilic properties, causing them to accumulate in the fat content of cheese due to the water-fat partition coefficient. This aspect is crucial for researchers as they need to extract these molecules from the cheese structure in order to subject them to appropriate analyses for volatile compounds (Le Quéré, 2022). Advances in analytical techniques, such as gas chromatography–mass spectrometry (GC–MS) and GC–O, provide better opportunities for the convenient and precise analysis of flavour compounds in food, including cheese. These techniques, collectively known as flavouromics, enable more efficient and accurate characterization of the diverse flavours in cheese and other food products.
Types of extractions
Distillation extraction, simultaneous distillation-extraction (SDE), solvent-assisted flavour extraction (SAFE), purge and trap (P&T), thermal desorption (TD), and solid-phase microextraction (SPME) are the most common extraction processes used in the determination of volatile components from cheese. In order to detect volatile compounds in matrices, extraction techniques such as solid-phase dynamic extraction (SPDE), in-tube extraction (ITEX), stir bar sorptive extraction (SBSE), and sorptive headspace extraction (HSSE) were used (Bertuzzi et al., 2018).
The distillation extraction technique enables the separation and concentration of volatile compounds from cheese samples through distillation. It is usually performed under reduced pressure (<10 -3 Pa), the so-called "vacuum distillation", to avoid the thermal decomposition of volatile compounds. The cheese sample is mixed with water, flushed with nitrogen, and sealed. The resulting suspension is distilled under a vacuum, and the extract is collected in cold traps. The extract can be concentrated by removing water by solvent extraction. A solvent (acetonitrile, pentane, dichloromethane, or diethyl ether) is usually added to the extract, and further vacuum distillation is carried out. The pH of the final extract can be adjusted to obtain different fractions (acidic, neutral, or alkaline) for injection into the gas chromatograph. Adjusting the polarity of the final extract causes the separation of volatile compounds. The acidic fraction contains mainly phenolic compounds and free fatty acids; the neutral fraction includes mainly alcohols, aldehydes, ketones, esters, and lactones; the alkaline fraction mainly contains nitrogen compounds, sulphur compounds, and terpenes (Bicchi et al., 2004). It is possible to perform extraction by distilling volatile compounds directly into the solvent. This extraction, called simultaneous distillation extraction (SDE), is faster than traditional distillation extraction. Distillation requires a lower temperature since the solvent usually has a low boiling point. This technique allows the extraction of volatile compounds from condensed solvent vapour (containing volatile compounds), separated from condensed water vapour (containing non-metallic compounds) concerning their density, with subsequent extract injection directly onto the GC column. SDE guarantees a high percentage of extraction and is an ideal technique for low-volatility components (Bertuzzi et al., 2018).
Solvent-assisted flavour extraction (SAFE) is another technique that allows the separation and concentration of volatile compounds by vacuum distillation. The distillation system consists of a vacuum pump and normally the distillate is collected in a round bottom flask, which is cooled with liquid nitrogen. The cheese sample is mixed with the solvent, and volatile compounds are collected by distillation with the solvent in the first flask, while impurities and water are condensed in the second flask. SAFE is a valid method that allows the extraction of volatile compounds without extensive preparation and is particularly suitable for analysis by GC-olfactometry due to the preservation of volatile compounds through low-temperature extraction (Zabaleta et al., 2016). However, SAFE has some limitations in extracting highly volatile compounds A disadvantage of solvent extraction techniques is the removal of any associated matrix effect (due to the composition of the cheese), and thus the technique favours the extraction of heavier volatiles, rather than volatiles released during mastication of the cheese in the mouth (Kilcawley, 2017).
In extraction methods such as P&T, TD, SPDE, and ITEX, the cheese sample is heated, and the volatile compounds are continuously removed and concentrated in a cold freezer or adsorbed on an inert support before being injected into a GC capillary column. Table 2 provides an overview of some important extractions.
Table 2. Different extraction methods applied for cheese sample
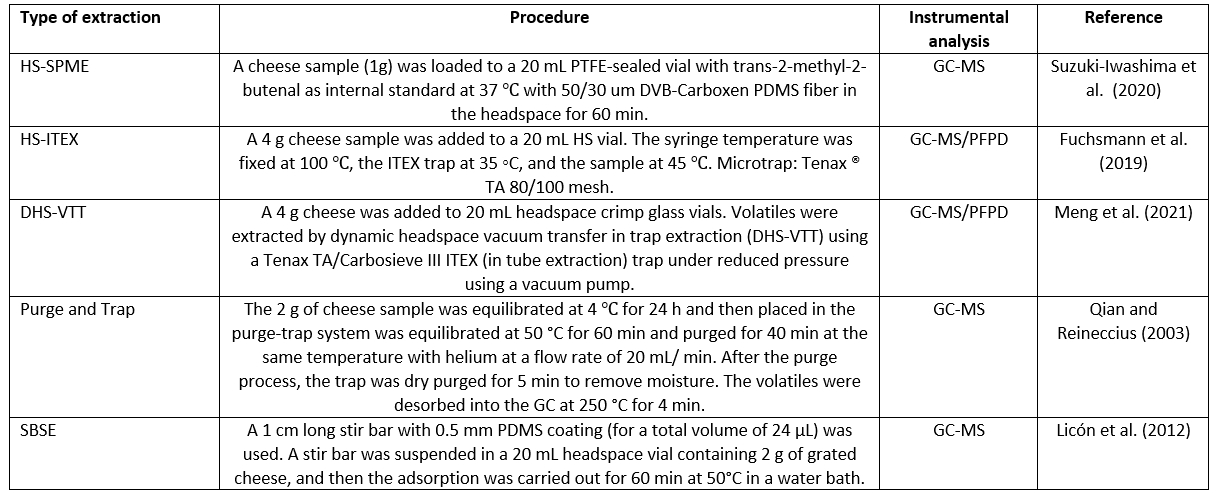
Abbreviations: GC-MS - gas chromatography-mass spectrometry; HS-SPME - headspace solid-phase microextraction; HS-ITEX - headspace in tube - extraction; DHS-VTT - dynamic headspace vacuum transfer in trap extraction; SBSE - stir bar sorptive extraction; PFPD - pulsed flame photometric detector
Solid phase microextraction is nowadays a common technique developed by Arthur and Pawliszyn (1990). SPME enables the extraction and concentration of volatile or semi-volatile compounds from the sample matrix (usually an aqueous solution used in the headspace) and good transfer to gas chromatography using a split less universal injector without modification. SPME has numerous advantages over traditional sample preparation approaches. SPME is an effective aroma analysis tool and solvent-free sample preparation technology. In solid-phase microextraction (SPME) a needle coated with a fibre is inserted directly into either a liquid or into the headspace of a sample to collect compounds. In contrast to conventional methods, which require a quantitative recovery of the analyte, SPME sampling is a one-time process, making quantitative adsorption difficult. In flavour research, the most commonly used sorption materials for SPME fibres are dimethylsiloxane (polydimethylsiloxane - PDMS) and carboxen/dimethylsiloxane (carboxen/divinylbenzene/polydimethyhylsiloxane - CAR/DVB/PDMS). The main disadvantages of SPME are the limited sorption capacity and the effect of substituting analytes with lower affinity for the coating. This is an undesirable phenomenon, especially when analysing substances in trace range.
Gas chromatography
GC is the most often used analytical technology in flavour research for separating and identifying volatile components. GC techniques employ a mobile phase, an inert carrier gas (such as helium, hydrogen, nitrogen) transport the extracted volatile chemicals through the GC column. The separation of compounds is based on the different strength of the interaction of the compounds with the stationary phase ("like-dissolves-like"-rule). The polarity of the stationary phase varies, usually depending on the analytes to be separated. The choice of GC column is the most influential factor for detecting compounds (Imhof and Bosset, 1994). GC capillary columns can have polar stationary phases (such as polyethylene glycol or nitro terephthalic acid modified with polyethylene glycol), resulting in a very efficient separation of polar compounds such as acids and some alcohols. Alternatively, columns can have non-polar stationary phases (such as PDMS) to separate compounds with low polarizability, such as aldehydes, ketones, esters, amines, hydrocarbons, and phenols. Non-polar columns are usually much more stable than polarized columns. Columns with intermediate stationary phases (phenyl-methylpolysiloxane, cyanopropylphenyl-methylpolysiloxane, or cyanopropylphenyldimethylpolysiloxane) have become more popular in recent years because they can detect a wider range of polarized and non-polarized volatile compounds. GC columns are heated in an oven according to a temperature program while analysing volatile chemicals. The column is then heated to a temperature below the maximum to eliminate any volatile or less volatile elements, leaving the column intact for the next sample. The volatile chemicals must be identified and quantified after the GC column separation. The detection techniques employed are diverse and complex, and the appropriate technology is chosen based on the product being examined and the range of analytes of interest. The most commonly used detectors in dairy product research for identification and quantification are quadrupole mass spectrometry detectors (MS), flame ionization detectors (FID) especially for high volatiles compounds, flame photometric detectors (FPD), and pulsed flame photometric detectors – (PFPD). High-resolution mass spectrometry (MS) techniques like Time-of-Flight Mass Spectrometry (TOF-MS) and Orbitrap are gaining popularity due to their increased sensitivity. However, their broader utilization is accompanied by higher costs and a need for more advanced technical expertise to ensure efficient operation. It is also possible to perform an olfactory analysis using the human nose called GC-olfactometry (Bertuzzi et al., 2018).
Conclusion
Aroma is a key component of the sensory experience of cheese. Consumers highly value the distinct aroma of different cheese varieties. By identifying and analysing the specific aroma compounds present in cheese, the food industry can ensure consistent quality and flavour profiles of their products. This helps maintain customer satisfaction and brand reputation. Determining aroma compounds in cheese is important in overall flavour perception. Aroma compounds contribute to the complex sensory experience of cheese, influencing its taste and texture. The distinct aroma of a cheese can evokes strong associations and emotions, making it a key factor in consumer preference. Additionally, analysis of aroma compounds in cheese.
helps cheese producers maintain consistency in quality, detect any undesirable odours or off-flavours, consumer satisfaction, contributes to innovation, marketing and food safety considerations.
Funding
This research was funded by the project "Potential of microencapsulation in cheese pro- duction" KK.01.1.1.04.0058 from the Operational Program Competitiveness and Cohesion 2014-2020 and supported by the “Food Safety and Quality Center” KK.01.1.1.02.0004 project, funded by the European Regional Development Fund.
Formiranje aromatskih spojeva u siru
Sažetak
Sir je jedan od najznačajnijih mliječnih proizvoda s velikom raznolikošću vrsta, oblika, teksture, okusa i arome dostupnih lokalno i globalno. Provedene su brojne studije o spojevima koji sudjeluju u formiranju arome i okusa te je identificirano više od 3000 hlapljivih i nehlapljivih spojeva u siru. Hlapljive komponente koje se sastoje od masnih kiselina, alkohola, aldehida, estera, ketona, laktona, sumpornih spojeva, amina i drugih aktivnih tvari zaslužne su za stvaranje aromatskih karakteristika u siru. U radu su detaljno objašnjeni biokemijski procesi tijekom formiranja značajnih aromatskih spojeva koji utječu na ukupnu kvalitetu i senzorne karakteristike sira te je pružen pregled najnovijih metodologija za ekstrakciju i određivanje hlapljivih spojeva u siru.
Ključne riječi: sir; aromatski spojevi; GC-MS; metode ekstrakcije