1. Introduction
Formation of emulsion with achi ( Brachystegia eurycoma) and red palm oil used in the production of complementary food to increase its pro-vitamin A content resulted in 52.7% reduction of the pro-vitamin A content of red palm oil (Attaugwu and Uvere, 2017). This could be as a result of lipoxidase activity or the presence of chemical compounds in B. eurycoma that binds pro-vitamin A into a complex that cannot be quantified. Malting is known to reduce anti-nutrients in grains and pulses and could be a remedy to this problem (Osagie and Eka, 1998).
Complementary foods are foods other than breast milk introduced to an infant (6-12 months of age) to provide nutrients (Gibbs, 2010). Complementary feeding is needed in the diet of a child when breast milk is no longer enough to meet the nutritional needs of the infant. This covers the age between 6-24 months and is a very vulnerable period. It is the time when malnutrition starts in many infants, contributing significantly to the high prevalence of malnutrition in children under five years of age worldwide (Shrimpton et al., 2001). At about 6 months of age, an infant is ready for other foods (Naylor and Morrow, 2001). Their digestive system is mature enough to digest the starch, protein, and fat in a non-milk diet. Hence, the food must contain about 110 g of protein, 202 to 548 kcal of energy, 5000 mg of calcium, 275 mg of iron, 125 mg of zinc and 5000 µg of vitamin A to complement breast milk (Lutter and Dewey, 2003). Infants who do not receive enough complementary foods are malnourished and stunted as a result of deficiency in energy, protein and micro-nutrients such as iron, zinc, iodine and vitamin A (Brown, 1991).
Complementary foods are mostly produced from plant foods which include cereals such as wheat, rice, maize (Ikujelola and Adurotoye, 2014), legumes, such as soya beans, cowpea, bambara groundnut (Attaugwu et al ., 2016), tubers ,such as yam, cassava and potatoes (Ijarotimi and Keshinro, 2013). Cereals can be used individually or in combination with legumes to produce high energy protein formulations (FAO/WHO, 1994). Examples of cereal-legume formulations are maize and cowpea, and/or maize and bambara nut (Attaugwu et al., 2016). These complementary foods are produced using various house-hold technologies such as fermentation (Gernah et al., 2011; Wakil and Kazeem 2012; Ijarotimi and Keshinro 2013; Attaugwu et al ., 2016), malting (Gernah et al., 2011; Ikujelola and Adurotoye, 2014; Attaugwu et al., 2016; Attaugwu and Uvere, 2017), toasting, sun drying or combination of these methods. However, these plant based complementary foods are low in the required nutrient density, because of the formation of insoluble complexes between nutrients (minerals, proteins and vitamins) and some antinutrients (Wakil and Kazeem, 2012). The method of processing ( soaking, milling) may lead to futher loss in nutrient, hence the problem of micro nutrient deficiency is still persisting.
Generally, infants are born with low vitamin A stores ( Allen and Haskell, 2001) and are dependent on external sources, most importantly, breast milk. However, in settings where vitamin A deficiency and/or under nutrition is common, infants are likely to receive inadequate amounts of this vitamin from breast milk due to poor maternal nutritional status. In many countries, where vitamin A deficiency is a public health problem, vitamin A supplements are provided to children 6-59 months of age to reduce the risk of illness and death. A high dose of vitamin A should be given to children with measles, diarrhea, respiratory disease, chicken pox and other infections or who live in the vicinity of children with vitamin A deficiency. Reduction in the pro-vitamin A content could lead to vitamin deficiency and associated diseases/complications in infants if such blends are used in the fortification of complementary foods. Therefore, the consumption of fortified complementary diet is highly recommended, because the nutrient compositions of complementary foods in developing countries are inadequate (Ijarotimi and Keshinro, 2013), especially for iron, zinc, calcium, and vitamin A, which are difficult to meet from non-fortified complementary foods.
Fortification of these plant-based complementary foods with vitamin and mineral premix or animal supplements, such as milk, make the food expensive for low income earners (Anon, 2012). The best alternative is to adopt food-to-food fortification. In an effort to increase the pro-vitamin A content of formulated complementary food by fortification of foods using an emulsion formed with red palm oil and B. eurycoma, the pro-vitamin A content reduced from 1724.12 µg RE/kg to 615.350 µg RE/kg (Attaugwu and Uvere, 2017). This suggests that B. eurycoma may contain a compound whose presence reduces the vitamin A content of red palm oil. Malting may help to reduce some of the components in pulses, such as B. Eurycoma, which destroy or form complexes with pro-vitamin A in red palm oil (Anderson and Wolf, 1995).The use of such malted pulses in complementary food formulation may enhance the pro-vitamin A content and availability. Malting is known to reduce anti-nutrients in legumes. Hence, this present study was carried out to determine the effect of malting of achi, bambara groundnut and cowpea on the pro-vitamin A content and the stability of red palm oil emulsions formed with malts.
2. Materials and methods
Healthy mature seeds of bambara groundnut ( Voandzeia subterranean Thouars), cowpea ( Vigna unguiculata) and red palm oil were purchased from Ogige market, Nsukka, Nigeria, while achi (Brachystegia eurycoma) was purchased from Orie Urba market Nsukka.
Sample preliminary preparation
The maize, bambara groundnut and cowpea seeds were cleaned by winnowing and hand-sorting to remove non-viable (broken, insect-infested) seeds, stones, empty pods and other extraneous materials.
Sample processing
Malting of bambara groundnut, B. eurycoma and cowpea seeds
The pulses were malted by the 2-step wet-steep method described by Uvere et al. (2010). The root length was determined on a daily basis using a meter rule (Bhutta, 2006). The green malts were dried in a convection Gallenkamp oven (Model IH-150, Gallenkamp, UK) at 50oC for 12 hours. After that the seeds were cleaned of sprouts and hulls by abrasion between the palms and winnowed. Malting loss was calculated and the cleaned malts milled into flour using a Bentall attrition mill (Model 200 L090, E. H. Bentall, UK) and sieved using a traditional nylon cloth to obtain the malt flours. The flours were stored in polyethylene bags in a refrigerator at 4oC.
Emulsion formulation
The emulsions were formulated using the malted flours, red palm oil and water in the ratio of 1:1:2 (w/v/v).
Analysis
Emulsion stability was determined using the method of Neto et al . (2001), with some modifications.
The proximate composition of the samples was determined using the standard procedures of AOAC (2010). The crude protein content was calculated by multiplying the total nitrogen by the factor 6.25. The carbohydrate content was estimated by difference. The tannin content was estimated spectrophotometrically by the Folin-Denis method (Makkar et al., 1993). The phytate content was determined using the procedure described by Lolas and Markakas (1975). Oxalate was determined using the method of Oke (1969). The pro-vitamin A content of the samples was determined by the method of AOAC (2010).
Statistical analysis
Determinations were carried out in triplicate and the error reported as standard deviations from the mean. Mean values of data obtained were subjected to analysis of variance (ANOVA) and mean separation carried out using Duncan Multiple Range test. The Statistical Products for Service Solution (SPSS, version 20 computer software, was used to carry out the analysis. Significance was accepted at p<0.05 according to Steel and Torrie (1980).
3. Results
Malting of achi, bambara groundnut and cowpea
The root length and malting loss of achi (Brachystegia eurycoma), bambara groundnut, and cowpea malts produced over 96 hours are shown in Figures 1 and 2. The results showed that the root
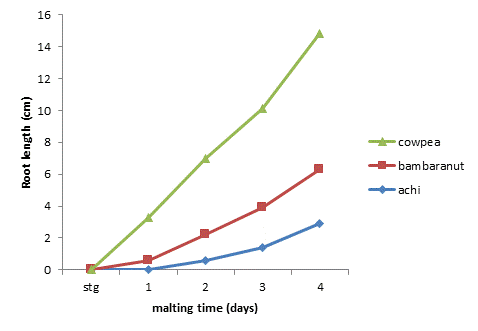
Figure 1. Time course variation of root length of achi, bambara groundnut and cowpea malts. out-of-steep grains (Stg)
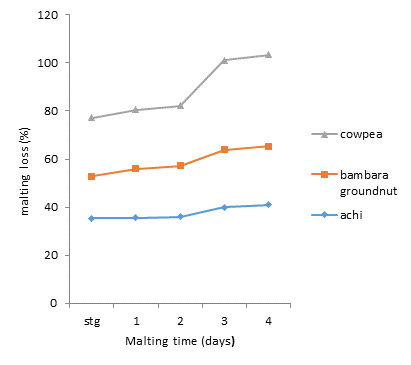
Figure 2. Time course variation of malting loss of achi, bambara groundnut and cowpea malts. out-of-steep grains (Stg)
length increased with germination time. The longest root length was observed on the fourth day of malting which suggests improved modification of the endosperm resulting from improved enzyme secretion and activities (Palmer and Bathgate, 1976), while on the first day it was the the lowest.
The root length was higher in cowpea malt compared to bambara groundnut and B. eurycoma and may be responsible for the higher malting loss of cowpea malt compared to bambara groundnut and B. eurycoma (Figure 2).
Proximate composition of the emulsions
The proximate composition of the red palm oil emulsions formed with malts of B.eurycoma, bambara groundnut and cowpea are presented in Table 1.The moisture content of the emulsions was in the range of 27.82 – 30.90% for achi malts, 36.20 – 37.99% for bambara groundnut malts and 30.50 – 35.73% for cowpea malts.
Samples Bb72 and C72 had the highest moisture content. This could be attributed to the slower utilization of sugar compared to its production by amylases. In sample BeC, however, increased moisture content could be attributed to the inherent moisture content of achi 10.25% (Ikegwu et al., 2010), compared to 9.23% for Bambara nut (Olaleye et al., 2013) and 5.3% for cowpea (Mune et al., 2013). Among these three samples, Bb72 had the highest moisture content, which implies that the amylolytic activity was the highest.
The protein content of the emulsions increased from 8.23 – 9.91% for achi malts, 18.02 -20.21% for bambara groundnut malts and 23.80 – 35.50% for cowpea malts. The highest values were found in Be96, Bb72 and C96. This is because malting breaks down protein-tannin complexes (Nout and Ngoddy 1997) leading to increased protein content. It could also be a result of mobilization of storage nitrogen of the seeds to produce high quality protein to aid the development of the young plant .TThese findings are in agreement with the reports of Attaugwu and Uvere (2017), who reported a 12.6% and 8.0% increase in protein content of malted cowpea and bambara groundnut, respectively, Malleshi et al. (1989), who reported significant increases in the protein content of weaning foods from sprouted sorghum and cowpea seeds, Kavitha and Parimalavalli (2014) ; Gernah et al. (2011), who also reported significant increases in the protein content of malted maize. C96 had the highest value, because malting had a higher effect on cowpea compared to achi and bambara groundnut.
The fat contents of the emulsions ranged from 7.00 – 7.20% for achi, 7.10 – 8.95% for bambara groundnut and 4.35 – 5.00% for cowpea malts. BeC, BbC and C96 had the highest value for the malts. This could be attributed to the inherent fat in the samples as a result of palm oil emulsification. BbC had the highest value compared to others, because bambara-ground nut has high fat content.
Table 1. Proximate composition (%) of the emulsions formed from the malts of bambara groundnut, B. eurycoma and cowpea
The ash content of the emulsions ranged from 0.8 – 1.19% for achi, 0.10 – 1.52% for bambara groundnut and 0.60 – 3.02% for cowpea malts. BeC, Bb72 and C96 had the highest ash content for the emulsions. This could be attributed to the migration of minerals from the bran to the endosperm of the grain during malting or due to the effect of malting on anti-nutrients leading to the release of bound minerals. Sokrab et al. (2012) has reported increases in both major and trace minerals during the germination of corn. The highest value was found in C96 and could be attributed to the highest malting loss by cowpea. Malting led to 16.56% and 16.31% increase in ash content of bambara groundnut and cowpea, respectively. A similar report of increase in ash content during malting has been reported by Gernah et al. (2011).
The crude fiber content of the emulsions ranged from 5.71 – 8.60% for achi, 1.91 – 3.21% for bambara groundnut and 1.20 – 2.93% for cowpea malts. The lowest values were found at Be96, Bb72 and C96. This could be as a result of malting as well as dehulling, which reduces the fiber content of the sample. The decrease may possibly have arisen from the action of hydrolytic enzymes present in the seeds and the mobilization of soluble nutrients into roots and shoots leading to the reduction of these nutrients. Similar results occurred in reports of Gernah et al. (2011); Kavitha and Parimalavalli (2014); Hahm et al. (2009). The carbohydrate content was calculated by difference and was in the range of 44.41 – 48.66% for achi malts , 30.76 – 34.16% for bambara groundnut malts and 21.02 – 34.00% for cowpea malts.
Pro-vitamin A contents of the red palm oil emulsions formed with the malted samples
The pro-vitamin A contents of the red palm oil emulsions formed with the malted samples are presented in Table 2. The pro-vitamin A contents of the emulsion increased from 33.30 to 400 µg RE/kg for achi malts, 66.67 to 333.33 µg RE/kg for bambara groundnut malts and 66.67 to 233.33 µg RE/kg for cowpea malts. The highest values were seen in the 96 hour malts. As malting time increased, the anti-nutrients that chelates or destroys pro-vitamin A, are destroyed (Anderson and Wolf, 1995). A similar result of increase in pro-vitamin A content of bambara groundnut and cowpea due to malting has been reported by Attaugwu and Uvere (2017).The increase in pro-vitamin A content of the emulsions was the highest in the emulsion formulated with the 96 hour malt of achi ( Brachystegia eurycoma), which had a pro-vitamin A content of 400.00 µg RE/kg, compared with that of 96 hour bambara groundnut containing pro-vitamin A of 333.33 µg RE/kg and 96 hour cowpea malts containing 233.33 µg RE/kg of pro-vitamin A. The malting has greater effect on the anti-nutrients of B. eurycoma compared to other samples.
Table 2. Pro-vitamin A content (µgRE/kg) of red palm oil emulsions formed with the malted samples
Anti-nutrient compositions of the emulsions
The anti-nutrients compositions of the red palm oil emulsions formed with malts from achi, bambara groundnut and cowpea are presented in Table 3.
The oxalate content decreased from 1.30 to 0.21 mg/100 g for achi malts, 2.90 to 0.34 mg/100 g for bambara groundnut malts and 1.60 to 0.40 mg/100 g for cowpea malts. This decrease in oxalate content of the samples could be attributed to leaching during steeping and reduction during the germination of the grains. The lowest value was found for Be96 (emulsion formed from 96 hour malt of achi), compared to Bb96 (emulsion formed from 96 hour malt of bambara groundnut) and C96 (emulsion formed from 96 hour malt of cowpea). The tannin contents of the emulsions decreased from 0.10 to 0.01 mg/100 g for achi malts, 0.12 to 0.03 mg/100 g for bambara groundnut malts and 0.05 to 0.02 mg/100 g for cowpea malts. This is also attributed to leaching and the activity of malt enzymes. Onyango et al. (2013) has reported a similar reduction in tannin content of red and white sorghum during malting.
The phytate contents of the emulsions decreased from 1.09 to 0.03 mg/100 g for achi malts , 4.26 to 1.04 mg/100 g for bambara groundnut malt and 2.8 to 0.40 mg/100 g for cowpea malts. This is attributed to losses during steeping and modification during the germination of the grain which reduces the anti-nutrients content of grain (Sokrab et al., 2012).The lowest phytate content was found in Be96, resulting in the higher effect of malting on Bb96, compared to Bb96 and C96.
Emulsion stability
The stability of the red palm oil emulsions formed with malts from achi, bambara groundnut and cowpea are presented in Table 4.
The emulsion stability determined over 3 hours indicates that out-of-steep malts, 24 hour malts and 48 hour malts has varying emulsion layers, but at 72 hour and 96 hour malts, the emulsion layers appear stable after 3 hours storage at 30oC. The results suggest that samples malted at 72 hour and 96 hour are stable, suggesting that malting resulted in the hydrolysis of oxalates, tannins and phytates-protein complexes to release proteins, which increased its capacity as emulsifiers. Proteins act as emulsifying agents, because of their amphoteric nature. Its molecules contain both hydrophilic (water-loving) and hydrophobic (water-hating) groups, which allow them to reduce the interfacial tension at the oil-water interface.
Table 3. Anti-nutrients contents (mg/100 g) of the formulated emulsions
Table 4. Stability of emulsions formed from malts of bambara groundnut, B. eurycoma and cowpea with red palm oil and water
Conclusion
Emulsification of red palm oil with 96 hour malt of achi ( Brachystegia eurycoma) led to the release of more vitamin A when compared to bambara groundnut and cowpea malt emulsions. The anti-nutrient compositions of the formulated emulsions decreased with malting time. The stability of the formulated emulsions was the highest with the malts of 72 hours and 96 hours. The best result was found to be in the emulsion formulated with 96 hour malt of achi. This implies that malting of the achi seeds may have reduced the components that bind pro-vitamin A in the unmalted samples. Hence, malted achi is recommended for food fortification of plant based complementary foods to increase their pro-vitamin A content.
Author Contributions: Peter Uvere originated the ideas and supervised the work, Stella Dioha carried out the laboratory work, and data collection, Roseline Attaugwu correlated the results and wrote the manuscript.
Funding: This research received no external funding.
Acknowledgements: We are grateful to the University of Management for providing the laboratory facilities used to carry out the work.
Conflicts of Interest: The authors declare no conflict of interest.