Introduction
Lactic acid bacteria (LAB) strains from human milk are capable of producing potential therapeutic biomolecules. Previously isolated four strains of Levilactobacillus brevis MB1, MB2, MB13, and MB20, are capable of producing S-proteins, as demonstrated after proteomic analysis and trypsinization followed by liquid chromatography-mass spectrometry (LC-MS) and Mascot search (Banić et al., 2022). Surface (S)-layer proteins (S-proteins) are two-dimensional, paracrystalline, monomolecular crystalline structures of surface (glyco)proteins that cover the cell surface of hundreds of different species of almost all taxonomic groups of Gram-positive and Gram-negative bacteria and archaea in the form of a layer (S-layer) (Assandri et al., 2023). As the outermost layer covering the surface of the cell, the S-protein layer is in direct contact with its microenvironment, and the role of S-proteins can be studied from two different approaches - their role in the adhesion of bacteria to various surfaces and substrates and their role as a mechanical barrier in a harmful environment (Gerbino et al., 2015). The expression of S-proteins in probiotic bacteria is a rare but desirable trait, as it has been shown to play an important role in the expression of the probiotic properties of the producer strain. It protects the cells from unfavourable environmental conditions, but also influences many cell surface properties, including surface recognition, auto aggregation and co-aggregation, and adhesion to various epithelial and subepithelial structures (Banić et al., 2018; Butorac et al., 2020; Novak et al., 2021; Banić et al., 2022). Probiotics not only have great potential for broad biotechnological application (Novak et al., 2022), but also have many health benefits, such as prevention of gastrointestinal tract (GIT) diseases and allergies, antidepressant, anticancer and immunomodulatory activities (Zommiti et al., 2020). However, their activity is impaired by the methods of powder production, storage and unfavourable conditions in the digestive tract (Vivek et al., 2023). One of the techniques for administering probiotics is microencapsulation in protective capsules that form a physical barrier to improve the survival and bioavailability of the probiotics (Razavi et al., 2021). Alginate is widely used as a carrier for the delivery of probiotics in microcapsules and has attracted attention due to its excellent physicochemical and mechanical properties, including simple structure, simple raw materials, low toxicity, mild processing, and easy matrix formation around the bacteria (Wang et al., 2022). Furthermore, in order to prolong the survival time of the encapsulated probiotics during storage and in the human GIT, prebiotic substrates that are indigestible or only partially digestible in the GIT can be added to stimulate the growth of the microbiota and probiotics in the human gut (Misra et al., 2022). There are numerous studies that report microencapsulation of probiotics in dairy products. For instance, Phoem et al. (2019) encapsulated L. plantarum TISTR1465 with Eleutherin Americana oligosaccharide extract using the extrusion technique demonstrated to be more effective than the free cells after exposure to the GIT and refrigerated storage in yoghurt. Also, Kavas et al. (2022) determined that administration of microencapsulated probiotic culture mixture ( Lacticaseibacillus casei + Bifidobacterium longum) in white goat cheese production had a positive effect on the amount of unsaturated fatty acids. Although microencapsulation of probiotics provides higher viability and stability during the storage and consumption of dairy products (El Kadri et al., 2018), it can alter textural and rheological properties (Li et al., 2020; Ningtyas et al., 2019). Therefore, it is important to choose appropriate encapsulation technique and coating materials for maintainig viability and food properties.
The aim of this work was therefore to investigate the role of S-proteins in enhancing the probiotic potential of the producer strains, particularly in relation to the better ability to survive simulated GIT conditions and better adhesion to Caco-2 cells, but also the protective effect of microencapsulation of L. brevis strains in alginate, with and without the addition of the prebiotic substrates fructooligosaccharides (FOS) and galactooligosaccharides (GOS), under unfavourable conditions during freeze-drying, passage through simulated GIT conditions and effect on viable cells stability during one-month storage.
Materials and methods
Bacterial strains and culture conditions
The S-layer-producing strains Levilactobacillus brevis MB1, MB2, MB13 and MB20, isolated from human milk, were maintained frozen at -80 °C in MRS (de Man Rogosa Sharpe; Difco, Detroit, MI, USA) broth supplemented with 15 % (by volume) glycerol (Alkaloid, Skopje, North Macedonia). All strains used in this study are part of the Culture collection of the Laboratory for Antibiotic, Enzyme, Probiotic and Starter Cultures Technology, University of Zagreb Faculty of Food Technology and Biotechnology. Prior to each experiment, all strains were sub cultured twice in a suitable medium under the growth conditions listed in Table 1.
Table 1. Bacterial strains used in this study
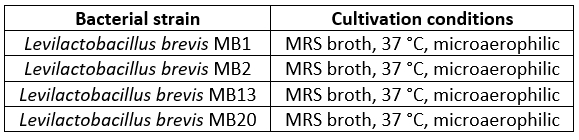
*microaerophilic conditions were created using Anaerocult A system (Merck, Darmstadt, Germany)
Treatment of Levilactobacillus brevis cells with guanidine hydrochloride
In order to remove S-proteins present on the surface of L. brevis MB1, MB2, MB13 and MB20, cells were harvested after overnight incubation, washed, resuspended in 3 M guanidine hydrochloride (Sigma-Aldrich, St. Louis, MO, USA) and incubated for 1 h with shaking at room temperature. Cells were then washed in distilled water to remove residual guanidine hydrochloride thus obtaining S-layer-depleted L. brevis strains (S-). Untreated L. brevis cells (S+) were prepared for the experiments in the same way, except that distilled water was used instead of guanidine hydrochloride. To confirm S-layer removal from the treated bacterial cells, protein extracts were subjected to Sodium Dodecyl Sulphate Polyacrylamide Gel Electrophoresis (SDS-PAGE) following protocol by Barukčić Jurina et al. (2024). Electrophoretic separation of proteins was performed using a power-supply PowerPac HC™ (Biorad, Hercules, CA, USA) at a constant voltage of 150 V, along with the ProSieve QuadColor Protein Marker 4.6-315 kDa (Lonza, Morristown, NJ, USA).
Survival of S-layer-producing Levilactobacillus brevis strains in simulated gastrointestinal tract (GIT) conditions
To study the survival of L. brevis strains MB1, MB2, MB13 and MB20 during incubation under simulated GIT conditions, simulated gastric and small intestinal juices were prepared as described by Kos et al. (2000). Simulated gastric juice was prepared by suspending pepsin (3 g/L; Sigma-Aldrich, Merck, St. Louis, MO, USA) in a sterile sodium chloride solution (0.5 %; Kemika, Zagreb, Croatia) and pH was adjusted with hydrochloric acid (Kemika, Zagreb, Croatia). Simulated small intestinal juice was prepared by suspending pancreatin (1 g/L; Fluka Biochemica, Buchs, Switzerland) and bile salts (1.5 g/L; Difco, Detroit, MI, USA). Overnight cultures of L. brevis strains were harvested by centrifugation, washed twice and resuspended in simulated gastric juice (pH = 2) and incubated for 2 h before cell viability was determined. After incubation in simulated gastric juice, the cells were harvested by centrifugation, resuspended in simulated small intestinal juice (pH = 8) and incubated for 4 h before cell viability was determined. The viable cell count was determined after 24 h of anaerobic incubation at 37 °C.
In vitro adhesion of S-layer-producing Levilactobacillus brevis strains to Caco-2 cell line
In vitro adhesion of L. brevis strains MB1, MB2, MB13, and MB20 to the Caco-2 cell line was performed as previously described by Butorac et al. (2021), with modifications. In brief, Caco-2 monolayers were seeded in 24-well tissue culture plates (Falcon®, Durham, USA) until confluent monolayers were obtained and then rinsed three times with phosphate-buffered saline (PBS, pH = 7.4). Subsequently, 1 mL of the prepared L. brevis bacterial suspension (approx. 109 CFU/mL) was added to each well and incubated for 1 h at 37 °C in an atmosphere of 5 % CO2. The monolayers were then washed three times with PBS to remove non-adherent bacterial cells. The total number of adhered bacterial cells in each well was counted after the Caco-2 cells were lysed with 0.05 % (by volume) Triton X-100 (AppliChem, Darmstadt, Germany) at 37 °C for 10 min. To determine the number of viable bacteria, adhered to the Caco-2 cells, appropriate dilutions in physiological solution were inoculated onto MRS agar plates and colony count was performed. Adhesion to the Caco-2 cell line was calculated using the following equation:
Δlog(CFU/mL) = log(CFU/mL)before adhesion - log(CFU/mL)after adhesion
Microencapsulation of S-layer-producing Levilactobacillus brevis strains
Microencapsulation of L. brevis strains MB1, MB2, MB13, and MB20 was performed according to the procedure described in Butorac et al. (2021) with some modifications. Overnight L. brevis cultures were pelleted by centrifugation at 4200 rpm for 10 min, washed twice and suspended in 2 % ( m/V) sodium alginate (Fluka, Buchs, Switzerland) and 2 % ( m/V) sodium alginate with addition of prebiotic substrates, fructooligosaccharides (FOS; Sigma-Aldrich, St. Louis, MO, USA) or galactooligosaccharides (GOS; Biosynth, Staad, Switzerland), at a concentration of 5 % ( m/V). Using a syringe and a needle (23 G), the mixture of bacterial cells and alginate or alginate+FOS or alginate+GOS was gradually dripped into a 1 % calcium chloride solution (Gram-mol, Zagreb, Croatia) while stirring on a magnetic stirrer, forming microcapsules. The beads were left on a magnetic stirrer for 1 hour to solidify. They were then washed twice with saline and the number of microencapsulated cells after release from the microcapsules was determined after treatment with 2 % ( m/V) Na-citrate (Kemika, Zagreb, Croatia) (Figure 1).
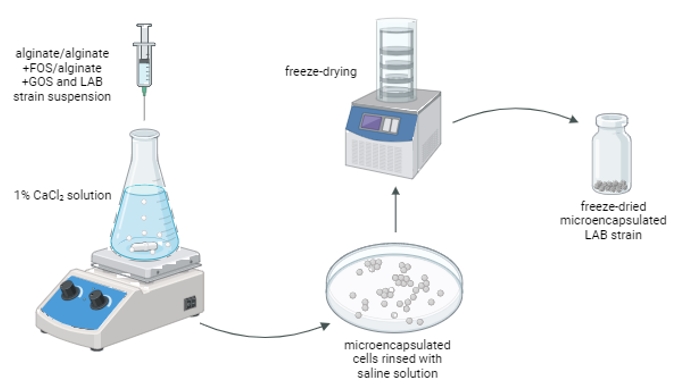
Figure 1. Schematic representation of the microencapsulation method (image created with BioRender.com)
In addition, the microcapsules were suspended in 10 % ( m/V) skimmed milk (Fluka, Buchs, Germany), frozen at -80 °C and freeze-dried in the Alpha 1-2 LD plus freeze dryer (Martin Christ, Osterode, Germany). The freeze-dried microencapsulated cells were examined for their viability after 1 month of storage at 4 °C, while the non-encapsulated (free) cells served as a control. Subsequently, the freeze-dried microencapsulated cells were exposed to simulated GIT conditions as described above.
The data obtained on the number of viable cells after freeze-drying, GIT transition and 1-month storage is expressed as cell mortality - a logarithmic reduction of colony forming units per gram of freeze-dried cell powder [Δlog(CFU/g)].
Statistical analysis
All experiments were repeated three times and the results were expressed as the mean of three independent values ± standard deviation. Statistical significance was determined by one-way analysis of variance (ANOVA). Pairwise differences between group means were determined using Tukey’s honestly significant difference test for post-analysis of variance pairwise comparisons (https://www.statskingdom.com/index.html, accessed on 11 February 2024) (Statistics Kingdom, 2017). Images were created using GraphPad Prism v.9.4.1 (GraphPad Software, USA). Differences between samples were considered significant at p<0.05.
Results and discussion
The role of S-layer proteins of Levilactobacillus brevis strains in simulated GIT conditions survival
Cell surface proteins profiling of LAB strains isolated from human milk revealed the presence of S-proteins in four strains, manifested by a characteristic band of about 50 kDa after SDS-PAGE, and gene encoding for S-proteins production were detected after whole genome sequencing of Levilactobacillus brevis strains MB1, MB2, MB13, and MB20 (Accession No. SAMN22155538, SAMN22155539, SAMN22155540 and SAMN22155541, respectively; BioProject No. PRJNA388578). In addition, the expression of S-proteins, after trypsinization of the protein bands cut from the polyacrylamide gel, was also confirmed by LC-MS after Mascot search (Banić et al., 2022).
These four L. brevis strains were used in an experiment investigating the protective role of S-layer proteins in the survival under unfavourable GIT conditions. This included a two-hour exposure to simulated gastric juice (pH = 2) followed by a four-hour exposure to simulated small intestinal juice (pH = 8). When comparing the viability of cells that were not treated with guanidine hydrochloride, a positive role of S-proteins was observed, as the number of live cells (CFU/mL) was significantly higher in untreated strains than in the same strains from which the S-layer was removed (Figure 2). S-proteins of L. brevis MB13 and MB20 strains had the greatest impact on survival in the GIT, as the strains without S-layer did not survive the harmful conditions of simulated small intestine juice. Our results are consistent with those of other researchers, such as Abramov et al. (2020) who demonstrated that Lactobacillus crispatus 2029 cells unable to produce Slp2 reduced the survival in simulated gastric and intestinal juices by 2–3 logs. According to previous results, L. brevis D6 strain, an S-protein producer, showed better ability to survive under simulated GIT conditions than the L. plantarum D13 without S-layer, which served as a negative control (Uroić et al., 2016), and as the Δlog(CFU/mL) of the L. brevis SF9B strain with S-layer was significantly lower than that of the same strain but without S-layer (Banić et al., 2018).
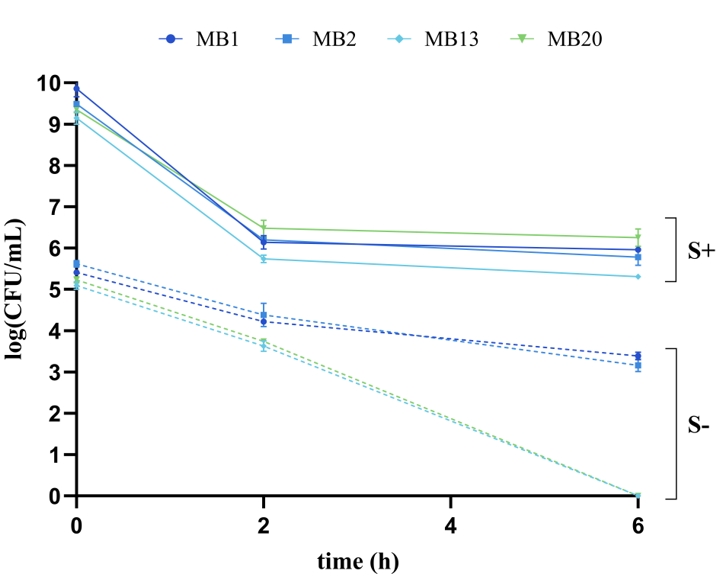
Figure 2. Viability of Levilactobacillus brevis MB1, MB2, MB13, and MB20 cells , with (S+) or without (S-) S-layer proteins, under simulated GIT conditions after incubation in simulated gastric juice (t = 2 h) and after incubation in simulated small intestine juice with direct transition (t = 4 h)
The role of S-proteins in ability of Levilactobacillus brevis to adhere to Caco-2 cell line
The ability to adhere to the host's intestinal surface is one of the main criteria in the selection of potential probiotic bacteria, as it leads to colonization and thus stimulation of the immune system as well as stimulation of the intestinal barrier and metabolic functions (Wang et al., 2022). In addition, probiotic bacteria have a potential protective role against enteropathogens through various mechanisms, which include the production of antimicrobial compounds, competition for binding sites and the resulting reduction in the adhesion of pathogenic strains (Monteagudo-Mera et al., 2019). Therefore, the adhesion ability of L. brevis strains MB1, MB2, MB13 and MB20, which possess S-layer on their surface, as well as strains in which the S-layer was removed by chemical treatment with guanidine hydrochloride, was tested on Caco-2 cells, since they serve as an intestinal epithelial barrier model (Figure 3). The results showed a positive role of S-layer proteins in adhesion ability, as the Δlog(CFU/mL) values of all tested L. brevis strains were higher when the cells were treated with guanidine hydrochloride, meaning that fewer L. brevis cells with the S-layer removed were able to bind to the Caco-2 cell line compared to the same strains expressing S-proteins on their surface. This effect was particularly evident in the L. brevis MB1 and MB20 strains, as the difference between the non-depleted and depleted strains was highest (p<0.0001). Our results are consistent with the evidence for the importance of S-proteins for the adhesion properties of the producer strain. Similar results were obtained by Klotz (2020), who demonstrated that S-layer deletion mutants of Lactobacillus acidophilus NCFM exhibited a reduced ability to bind to the Caco-2 cell line, as well as adhesion to extracellular matrices and mucin in vitro.
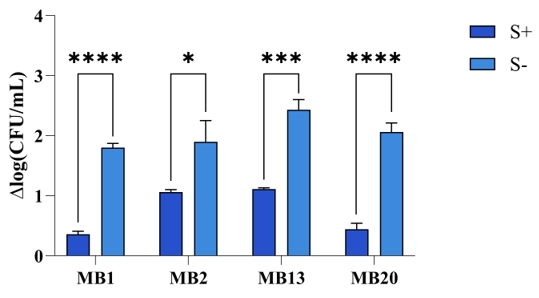
Figure 3. Adhesion of Levilactobacillus brevis strains MB1, MB2, MB13, and MB20, with (S+) or without (S-) S-layer proteins, to the Caco-2 cell line. Lower Δlog(CFU/mL) values indicate better adhesive properties of the strains tested. *Significantly different (*p<0.05, ***p<0.001, ****p<0.0001)
Microencapsulation of S-layer-producing Levilactobacillus brevis strains in alginate, with the addition of FOS and GOS as prebiotics, and their role in survival under adverse conditions
In recent decades, modern and innovative microencapsulation methods have been developed, leading to the production of a wide range of functional probiotic microcapsules. Successful methods used in the microencapsulation of probiotics include various physical and chemical principles, such as spray drying, spray cooling, spray freeze drying, freeze drying, lyophilisation, electro spraying, layer-by-layer (LbL) method, fluidized bed drying, extrusion, vibrating nozzles technology, emulsification and coacervation (Pech-Canul et al., 2020). The extrusion technique is a popular method due to its simplicity, low cost on a small scale, easy handling and mild formulation conditions that ensure the survival of bacterial cell (Frakolaki et al., 2021). A hydrocolloid solution (e.g. alginate) is prepared, microorganisms (probiotics) are added and the cell suspension is pressed through a needle with gentle mixing into a solution with polyvalent cations (Figure 1). For microencapsulation in alginate polymer, a calcium chloride solution is usually used, in which calcium alginate beads are formed in which bacterial cells are enclosed. Concentration ranges of 1-2 % alginate and 0.05-1.5 M calcium chloride are common (Pech-Canul et al., 2020). In addition to the classic carriers, the addition of oligosaccharides such as β-glucan and FOS has been shown to improve the survival of probiotics. Prebiotics are not degraded by enzymes in the GIT, but can be utilised by bifidobacteria, LAB and other desirable bacterial species in the colon (Liao et al., 2019).
In order to protect the LAB, producers of potential therapeutic biomolecules, and thus increase their probiotic potential, one of the objectives of this work was to carry out the microencapsulation of L. brevis strains in alginate, alginate with the addition of GOS and alginate with the addition of FOS by the extrusion method (Figure 4).
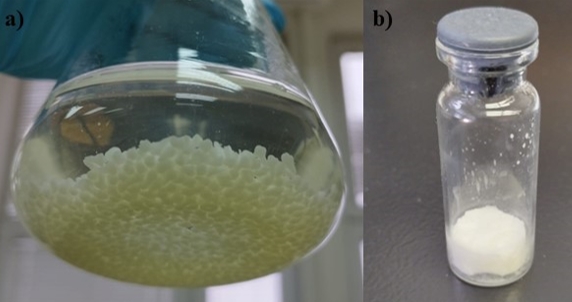
Figure 4. Microencapsulated Levilactobacillus brevis MB1 cells in alginate after dipping into a 1 % calcium chloride solution (a) and freeze-dried capsules (b)
The efficacy of the microencapsulation process of strains producing S-proteins was also tested (Table 2). The efficiency of microencapsulation of strains in alginate with the addition of GOS was highest for all strains, except for strain MB1, for which there was no significant difference between the different compositions of microcapsules. According to previously published studies, the efficiency of microencapsulation by extrusion is between 89.2 % and 98.8 %, but results with a lower efficiency of 30.6 % to 45.5 % have also been published (How et al., 2022).
Table 2. Efficiency of the microencapsulation (encapsulation yield, EY) of Levilactobacillus brevis MB1, MB2, MB13, and MB20 cells in alginate, with or without addition of fructooligosaccharides (FOS) or galactooligosaccharides (GOS)
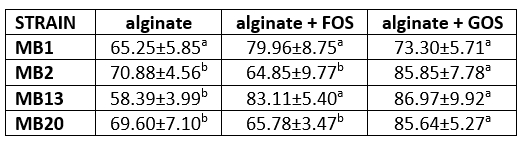
Different letters in superscript indicate statistically significant difference among the data across the same row (p<0.05)
When probiotic preparations are administered orally, the conditions for their survival are harsh, so that the number of live probiotic bacteria decreases by 106 CFU/g preparation after only 5 min of incubation in simulated gastric juice (Dodoo et al., 2017). Many probiotics in commercial products belong to the genus Lactobacillus or Bifidobacterium, which are sensitive to unfavourable conditions in the stomach and intestines. Therefore, it is necessary to develop effective methods to improve the stability of probiotics in food and during their passage through the human GIT. Microencapsulation has been proposed as an effective method to protect probiotics from degradation under such unfavourable conditions (Sipailiene and Petraityte, 2018; Razavi et al., 2021). Probiotic delivery system should be designed to release the probiotics in the colon so that they can have a beneficial impact on the health of the host. In addition, probiotics should be able to adhere to and colonize the colonic mucosa, otherwise they will not survive to have positive effects on host health (Bosnea et al., 2017 Wang et al., 2022). An effective microencapsulation system should maintain the stability of the probiotics during storage, protect them in the upper part of the GIT and release them in the colon so that the probiotics can adhere to and colonize the gut (Anselmo et al., 2016; Cassani et al., 2020).
Freeze-drying is one of the most suitable methods for drying sensitive materials such as microorganisms, and it also has good dissolution/suspension properties of dried materials. Despite the advantages of freeze-drying, there might be a significant loss of probiotic survival during the drying process and storage period due to changes in the membrane lipids and sensitive proteins of the bacterial cell caused by the low temperatures during the freezing phase, the formation of ice crystals and the evaporation rate of these crystals (Gul and Atalar, 2018). Skim milk was used as a lyoprotective agent in the freeze-drying of microcapsule samples containing probiotics, as it has also been shown in our previous research to stabilize the cell membrane and provide the cell with a protective layer (Butorac et al., 2021). The results (Figure 5) show that cell mortality [Δlog(CFU/g)] was significantly lower for MB1 and MB20 strains compared to alginate/alginate+FOS/free cells. Free cells of the MB2 strain had a significantly higher cell mortality rate than microencapsulated cells. As for MB13 strain, cell mortality was significantly lower with alginate+GOS and alginate+FOS compared to alginate alone and free cells. Overall, microencapsulation of L. brevis strains in alginate with the addition of GOS proved to be the best method for protecting cells during freeze-drying for all strains tested. Microencapsulation of the Limosilactobacillus fermentum D12 strain in alginate significantly improved the ability of the tested strain to survive the freeze-drying process as well as survival under simulated GIT conditions compared to the non-encapsulated strain (Butorac et al., 2021). Raddatz et al. (2020) demonstrated that other microencapsulation methods (emulsification) with the addition of various prebiotic substrates (hi-maize, inulin, and rice bran) can also improve survival in the freeze-drying process, but also under other unfavourable conditions.
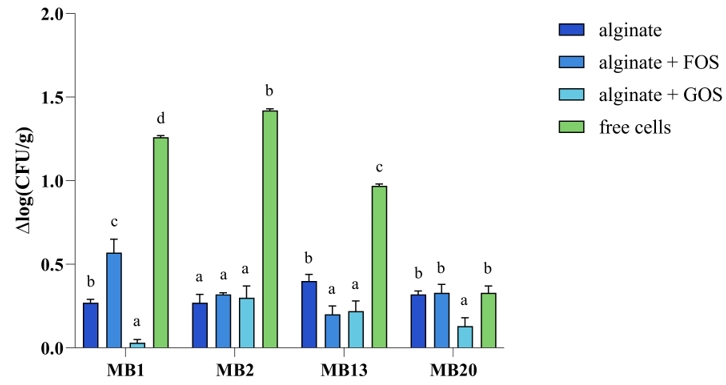
Figure 5. Cell mortality [Δlog(CFU/g)] of free and microencapsulated Levilactobacillus brevis MB1, MB2, MB13, and MB20 cells in alginate, with or without addition of FOS (fructooligosaccharides) or GOS (galactooligosaccharides), after freeze-drying
After oral administration, probiotic bacteria are confronted with extremely low pH values in the stomach, and in the colon there is competition for nutrients and adhesion sites on the mucosa, the action of the host's immune system, all of which can affect the concentration of live microorganisms in situ (Butorac et al., 2021). Therefore, the ability of freeze-dried microencapsulated S-protein-producing L. brevis strains to survive simulated GIT conditions was evaluated (Figure 6). Microencapsulation in alginate with the addition of GOS proved to be the best method to protect the cells of all tested L. brevis strains, as a statistically significant difference was found when examining cell mortality [Δlog(CFU/g)], compared to other microencapsulation methods as well as to free cells. Our results confirmed that microencapsulation techniques improve survival in the GIT, thus contributing to probiotic delivery to the target site of action, which was confirmed by other authors (Butorac et al., 2021; Pupa et al., 2021; Barajas-Alvarez et al., 2022).
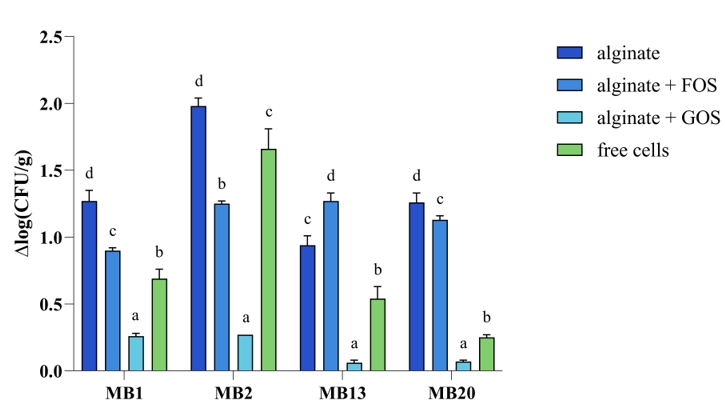
Figure 6. Cell mortality [Δlog(CFU/g)] of free and microencapsulated Levilactobacillus brevis MB1, MB2, MB13, and MB20 cells in alginate, with or without addition of FOS (fructooligosaccharides) or GOS (galactooligosaccharides), after exposure to simulated GIT conditions
The survival of lyophilized microencapsulated L. brevis strains stored at 4 °C for one month was also monitored. The results show that cell mortality [Δlog(CFU/g)] after one month of storage is significantly lower when the L. brevis MB1 and MB2 cells are microencapsulated in alginate with the addition of GOS, compared to alginate with or without the addition of FOS and the non-encapsulated free cells (Figure 7). As for the other two strains, L. brevis MB13 and MB20, the addition of GOS was also successful, but the addition of FOS had a similar positive effect on maintaining cell viability.
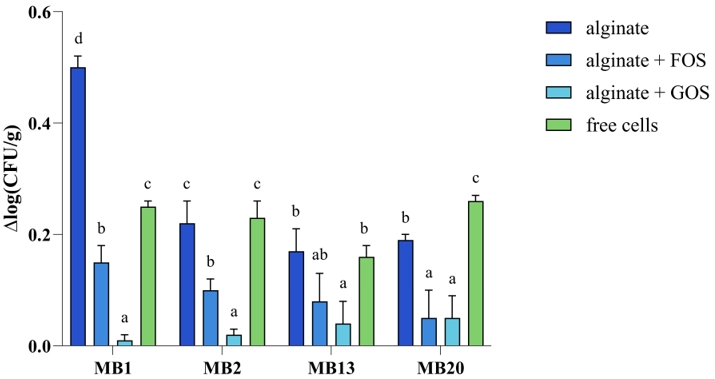
Figure 7. Cell mortality [Δlog(CFU/g)] of free and microencapsulated Levilactobacillus brevis MB1, MB2, MB13, and MB20 cells in alginate, with or without addition of FOS (fructooligosaccharides) or GOS (galactooligosaccharides), after one month of storage
Since potential prebiotic substrates were used in the microencapsulation process, these matrices, in addition to their protective effect, also play an important role as potential carbon and energy sources for the intestinal microbiota and for the probiotic strains in the microcapsules themselves, which are indigestible and are only metabolized by the metabolic activity of the microbiota in the intestinal tract, producing short-chain fatty acids whose beneficial effects on host health have been demonstrated in numerous studies (Markowiak-Kopeć and Śliżewska, 2020; Robles-Vera et al., 2020; Cheng et al., 2022).
Conclusions
The S-proteins located on the surface of L. brevis strains MB1, MB2, MB13 and MB20 showed a protective role in survival under simulated GIT conditions and positive influence on adhesion to the Caco-2 epithelial cells, thus demonstrating an additional competitive advantage of S-protein-producing bacteria in the selection of probiotic strains. From the results of the encapsulated and free cells of the tested strains of L. brevis after freeze-drying and passing through simulated GIT conditions, it can be concluded that the final number of live cells in all samples was above 108 CFU/g, so that the freeze-dried preparations of both free and encapsulated probiotic cells met the demanding technological criteria for achieving a probiotic effect (> 108 CFU/g). The results of a higher number of encapsulated cells surviving freeze-drying than free cells can be attributed to the protective effect of the alginate, GOS and FOS as protective and prebiotic agents.
Funding
This work was funded by the Croatian Science Foundation through the projects IP-2014-09-7009 and IP-2019-04-2237. The authors also acknowledge the financial support from the University of Zagreb, Croatia. The authors are also grateful for the equipment provided with financial support from the project “Bioprospecting of the Adriatic Sea” (KK.01.1.1.01.0002), granted to the Scientific Centre of Excellence for Marine Bioprospecting (BioProCro) at the Ruđer Bošković Institute, Zagreb, Croatia. Also, PhD student Nina Čuljak was a recipient of FEMS Research and Training Grant (FEMS-GO-2021-034) for early career scientists.