Introduction
Cancer is a complex group of diseases that occur due to the uncontrolled growth and spread of abnormal cells in the body (Desai et al., 2023). These abnormal cells can divide and multiply rapidly, leading to the formation of tumors or malignant masses that can interfere with the normal functioning of organs and tissues. The tumors formed by these cells can invade surrounding tissues and organs, and in some cases, they can travel through the bloodstream or lymphatic system to other parts of the body and form new tumors. Cancer can affect any part of the body, including the lungs, liver, colon, breasts, skin, and many others. Furthermore, cancer can develop due to a wide range of complex factors. It is often caused by genetic mutations that occur within cells, as well as environmental factors and lifestyle choices (Liu et al., 2022). Some of the most common risk factors associated with cancer include tobacco use, exposure to certain chemicals and substances, radiation exposure, a family history of cancer, certain infections, and aging (Meijers and de Boer, 2019). All these factors can contribute to the development of cancer by damaging DNA and other genetic material within cells, leading to abnormal growth and division of cells that can eventually become cancerous. Cancer symptoms can vary depending on the type of cancer and the stage of the disease. Some common symptoms of cancer include fatigue, which is often persistent and unexplained, as well as unexplained weight loss, which may occur rapidly or over a longer period. Pain is also a common symptom of cancer, and it can occur in various parts of the body, depending on where the cancer is located. Changes in the skin, such as darkening, yellowing, or redness, may also be a sign of cancer. Additionally, persistent coughing or difficulty swallowing may indicate the presence of cancer in the lungs or throat. Cancer diagnosis involves multiple stages of evaluation, beginning with a comprehensive medical history assessment, including an analysis of a patient's risk factors and symptoms (Nooreldeen and Bach, 2021). A physical examination follows, where medical professionals look for signs of cancer such as lumps or abnormal growths. Imaging tests, like CT (computed tomography) scans, MRIs (magnetic resonance imaging), and PET (positron emission tomography) scans, help visualize the inside of the body and detect any abnormalities. Laboratory tests, including blood tests and biopsies, help identify cancer cells and determine their type and stage. Various treatment options are available for cancer, including surgery, which is often the first choice for removing tumors. Chemotherapy involves the use of drugs to kill cancer cells, while radiation therapy uses high-energy radiation to destroy cancer cells. Immunotherapy and targeted therapy are newer promising approaches that have been proposed. Hormone therapy is used to treat certain types of cancer that are hormone sensitive. The choice of the treatment depends on several factors, including the type and the stage of cancer, the patient's overall health, and their personal preferences. Preventive measures can significantly reduce the risk of developing cancer. A healthy lifestyle, including a balanced diet and regular exercise, is one of the most effective ways to prevent cancer. It is important to note that there is no specific "anticancer food" that can guarantee cancer prevention. However, maintaining healthy and balanced diet rich in certain nutrients can contribute to overall health and potentially decrease the risk of specific types of cancer. Therefore, adopting a dietary approach that prioritizes nutrient-dense foods may be beneficial in promoting optimal health and reducing the likelihood of developing cancer. Several studies have examined the relationship between milk and its potential role in cancer prevention (Badawy et al., 2018; El-Gawad et al., 2021; El-Maksoud et al., 2021; Mahmoudi et al., 2023). Milk and other dairy products are known to be rich in essential nutrients such as calcium, vitamin D, proteins, and milk fat, all of which are crucial for overall health and well-being. However, it's important to note that the specific impact of milk on cancer risk is complex and may vary depending on the type of cancer. Some studies have suggested that certain components found in milk, like calcium and vitamin D, may have protective effects against colorectal cancer. Additionally, the anticancer effect of milk fatty acids was the subject of analysis in numerous scientific papers (Narayanan et al., 2015; Zhang et al., 2022; Day et al., 2022; Poonam et al., 2020; Chhikara et al., 2011; Lappano et al., 2017; Chhikara et al., 2010; Zeng et al., 2008; To et al., 2020; Zhu et al., 2021; Yu et al., 2023; Effenberger et al., 2010; Xu et al., 2019; Hardy et al., 2003; Khan et al., 2013; Liu et al., 2009; Beesley et al., 1993; Venn-Watson et al., 2020; Hasi et al., 2019; Kumari et al., 2012; Nath et al., 2022; and Kaplan et al., 2013).
Fatty acids (FA) are carboxylic acids comprising an aliphatic chain, which may be either saturated or unsaturated. They constitute a significant constituent of lipids, contributing up to 70 % by weight in certain species. Fatty acids are typically not present in their isolated form; instead, they exist primarily as three main classes of esters, including triglycerides, phospholipids, and cholesteryl esters. Fatty acids play significant roles in signal-transduction pathways, cellular fuel sources, protein modification, and energy storage. Also, FA represents fundamental structural components of cells. Fatty acids can be categorized in many ways, such as by length, saturation versus unsaturation, even versus odd carbon content, and linear versus branched. Their classification based on length includes short-chain fatty acids (SCFAs), medium-chain fatty acids (MCFAs), long-chain fatty acids (LCFAs), and very long-chain fatty acids (VLCFAs). Saturated fatty acids are devoid of C=C double bonds, and they have the formula CH3(CH2) nCOOH, where n varies. Conversely, unsaturated fatty acids comprise one or more C=C double bonds. These bonds can produce either cis or trans isomers. Studies have shown that dietary polyunsaturated fatty acids (PUFAs) can play a significant role in inhibiting tumor development (Selvaraj, 2017). PUFAs are essential fatty acids that cannot be synthesized by the human body and must be obtained through diet. They are known to have anti-inflammatory properties, which can help to prevent the growth and spread of cancer cells. In addition to PUFAs, short-chain fatty acids (SCFAs) have also been identified as having the potential to boost the efficacy of immunotherapy (Westheim et al., 2022). SCFAs are produced by the gut microbiota and are known to modulate the immunogenicity of tumor cells. They can also have a direct effect on the immune system in the context of cancer by regulating the function of immune cells.
In light of the multiple accusations and widely accepted notion that saturated fatty acids present in milk and dairy products could be detrimental to one's health, this study aims to provide a comprehensive overview of the anti-cancer properties of medium and long-chain saturated fatty acids, as well as to compare their concentration in the milk of three distinct domestic animal species, namely cattle, goats, and horses. The research aims to provide a detailed analysis of the fatty acids' potential to prevent cancer, in order to better understand their benefits and drawbacks in the context of human health.
Materials and methods
Experimental design
In this research, milk samples were collected from three different animal species in Slovenia and Croatia, namely Simmental cows, Saanen goats, and Lipizzaner mares. During the experiment, all animals were clinically healthy. The milk samples were analysed for fatty acid profiles. The contents of five MCFAs (Caprylic, Pelargonic, Capric, Undecylic, and Lauric), and ten LCFAs (Myristic, Pentadecylic, Palmitic, Margaric, Stearic, Nonadecylic, Arachidic, Behenic, Tricosylic, and Lignoceric) have been determined.
In July, cow's milk was collected from 15 Simmental cows held in an outdoor system in Slovenia. Simmental cows were pastured at mountain Velika Planina, at an altitude of 1400-1600 m, and milked twice a day. The cows were divided into three groups, based on their lactation stage (I. calving - 100 days of lactation; II. 100-200 days of lactation; III. 200-300 days of lactation) and two groups based on their parity (I. primiparous cows; II. cows in second and higher lactation). Milk samples were taken from each cow during milking, and transported to the laboratory for analysis of fatty acid profiles.
In September, goat's milk was collected from 30 Saanen goats, held in a farm with machine milking in Slovenia. The goats were milked twice a day and fed hay ad libitum, which was spread in the manger twice a day. Additionally, the goats were given a fodder mixture at the milking parlor, which they consumed at each milking. The animals were divided into three groups based on their lactation stage; after weaning in the 4th to 10th week of lactation (I.), in the 11th to 20th week (II.), and over the 20th week of lactation (III). All goats had already weaned kids. Milk samples were taken from each goat during milking and transported to the laboratory for analysis of fatty acid profiles.
In June and September, mare's milk was collected from 16 Lipizzaner mares reared on a state stud farm in eastern Croatia. The mares were on pasture with meadow grass, and in addition to grazing, they received 4.5 kg of oats daily and hay ad libitum. The mares were hand-milked once a day in the morning 3 h after the physical separation from their foals. The mares were divided into two groups based on their lactation stage (in the fourth and sixth months of lactation) and two groups based on their parity, with Lipizzaner mares classified as younger (from first to third lactation) and older (over third lactation). Milk samples were taken from each mare during milking and transported to the laboratory for analysis of fatty acid profiles.
Gas chromatography (GC)
A gas chromatograph (Agilent 8860; Agilent Technologies. Inc., Santa Clara, CA, USA) equipped with a flame ionization detector (FID) and ALS was used to analyze fatty acid methyl esters. The injector temperature was set at 200 °C and the detector temperature at 240 °C. Chromatography was completed using a capillary column DB-23 (Agilent Technologies, Santa Clara, CA, USA), with a length of 60 m, an inner diameter of the column of 0.25mm, and an active layer thickness of 0.25 μm. The initial column temperature was 150 °C for 2 minutes, then increased to 230 °C by heating at 5 °C/min and held at that temperature for 20 minutes. Hydrogen was used as the carrier gas with a flow rate of 1 mL/min. The computer program OpenLAB CDS ChemStation Workstation VL was utilized for the collection and processing of results. Identification of fatty acids was completed through a comparison of retention times with methyl standards (Sigma Aldrich Chemie, GmbH and Supelco, St. Louis, MI, USA). Nonadecanoic acid methyl ester (C19:0) was used for quantification. The percentage of each fatty acid relative to the total fatty acids was calculated to determine the fatty acid composition.
Statistical analysis
To prepare and analyse the data, SAS software (SAS, 2019) was used. To assess the effect of an animal's species (cattle, goat, and horse) on the values of medium and long chain saturated fatty acids in milk, a GLM (Generalized Linear Model) procedure in SAS was applied, utilizing least-square means. The applied statistical model (1) was as follows:
yijklm = µ + Li + Pj + Mk + Sl + eijklm (1)
where:
yijklmn = estimated saturated fatty acid (SFA)
µ = intercept;
Li = fixed effect of lactation stage i;
Pj = fixed effect of parity j;
Mk = fixed effect of sampling month k;
Sl = fixed effect of animal’s specie l;
eijklm = residual.
To determine the significance of differences between estimated LSMeans, Scheffe's method of multiple comparisons in the SAS GLM procedure was applied.
Results and discussion
The results of this analysis of the content of saturated fatty acids in milk from three different animal species - cattle, goats, and horses, have been presented in Tables 1 and 2 for medium-chain fatty acids (MCFAs) and long-chain fatty acids (LCFAs), respectively. The study revealed that the concentration of caprylic MCFA did not show any statistically significant (p>0.05) difference with respect to the animal species. However, the concentration of caprylic MCFA in goat milk (2.686 g/100 g of total FA) was observed to be several times higher than that in mare's milk (0.463 g/100 g of total FA). Furthermore, the content of pelargonic acid was found to be higher in goats’ milk (0.776±0.227 g/100 g of total FA) as compared to mare's milk (0.479±0.176 g/100 g of total FA). The analysis of capric acid showed statistically significantly (p<0.01) higher values in cow's milk (10.209±1.397 g/100 g of total FA) when compared to goat's milk (5.935±0.867 g/100 g of total FA) and mare's milk (4.659±0.769 g/100 g of total FA).
Kompan and Komprej (2012) have reported that goats' milk contains significantly higher amounts of medium-chain fatty acids (MCFAs) than cows' milk, specifically for caprylic (C8:0) and pelargonic (C9:0) fatty acids. Furthermore, according to a study by Kompan and Komprej (2012), the unique flavour and health benefits of goat milk are largely attributed to the predominance of three medium-chain fatty acids - caproic, caprylic, and capric. Interestingly, these fatty acids were named after goats because of their abundance in goats' milk. However, cows' milk also contains MCFAs, albeit in lower proportions than goat milk. Nevertheless, the presence of MCFAs in cow milk also contributes to its flavor and various health benefits.
Caproic acid (hexanoic acid), caprylic acid (octanoic acid), and capric acid (decanoic acid) are medium-chain fatty acids that have been found to have anti-cancer effects (Narayanan et al., 2015; Negm et al., 2022). Recent studies have revealed the agonistic behavior of caproic, caprylic, and capric acid with calcitriol, in regulating inflammatory mediators in colon cancer cells (Narayanan et al., 2015; Negm et al., 2022). In this study, a colorectal cancer cell line was induced using LPS/TNF-α, and the inflammation and metastatic mediators (IL-1β, IL-6, IL-17) were evaluated in colon cancer cells supplemented with capric acid and calcitriol. The mRNA and protein expression of MMP-2, NF-κB, and COX-2 were also quantified. Zymogram analysis confirmed the significant reduction in MMP-2 expression with combination treatment. These findings highlight the anti-inflammatory and anti-metastatic potential of capric acid and calcitriol in individual exposure and in a combination of human colon cancer cell lines. According to a study conducted by Narayanan et al. (2015), medium-chain fatty acids are known to possess remarkable anti-cancer properties. These properties have been found to significantly reduce the viability of cancer cells by up to 90 % compared to control groups. The fatty acids work by regulating the genes responsible for controlling cell cycles, thereby slowing down the rate of cell division. Additionally, they stimulate the genes responsible for programmed cell death (apoptosis), which leads to a reduction in the overall number of cancer cells. The results of this study show that medium-chain fatty acids have the potential to be used as a therapeutic for several types of cancer, including colorectal, skin, and breast cancer.
Similarly, like Kompan and Komprej (2012), Rodríguez-Alcalá et al. (2017) reported a higher value of caprylic acid in goats than in cows’ milk, that is they found that cow's milk contained 1.17 g/100 g total fatty acids of caprylic acid, while goat's milk had 2.73 g/100 g total fatty acids of the same acid. Rodríguez-Alcalá et al. (2017) also reported that the content of capric acid (C10:0) in goats' milk (9.97 g/100 g of total FA) was significantly (p>0.05) higher than in cows' milk (2.48 g/100 g of total FA), which is not in agreement with the results of this research. Mollica et al. (2021), emphasised that in contrast to ruminant milk, equid milk is characterised by a unique lipid composition with a particularly high percentage of total polyunsaturated fatty acids. Furthermore, Jastrzębska et al. (2017) noted that mare's milk is rich in monounsaturated fatty acids. The findings of this research suggest that caprylic and pelargonic acid present in goats' and mare's milk, respectively, along with capric acid found in milk of all three species, with the highest concentration in cows' milk, hold great promise as a potential therapy for various types of cancer, such as colorectal, skin, and breast cancer. These fatty acids have shown remarkable anti-cancer properties in several studies (Narayanan et al., 2015; Zhang et al., 2022), thus highlighting their potential as a beneficial nutritional supplement for cancer patients.
Pelargonic acid, also known as nonanoic acid, is a nine-carbon fatty acid that has recently garnered attention for its potential as an effective delivery vehicle for anticancer drugs. A study conducted by Zhang et al. (2022) examined the efficacy of an amphiphilic conjugate of carboxymethyl xylan-nonanoic acid (CX-NA) as a drug encapsulator. The study found that CX-NA was an efficient encapsulator of the model drug, 10-hydroxy camptothecin (HCPT), and that the drug-loaded micelles demonstrated excellent stability and permeability in the intestinal environment. The study also revealed the mechanisms of delivery vehicle for anticancer drugs by which HCPT was taken up by cells through clathrin-mediated endocytosis and that an intestinal in situ absorption study further confirmed that the CX-NA vehicle could enhance HPCT transport across intestinal epithelial cells in colonic tissues. Additionally, the formulation showed excellent anti-tumor activity in vitro and improved bioavailability by 3.4 times in vivo when compared with free HCPT.
Table 1. LsMeans of medium-chain fatty acids ( MCFAs) regarding the animal’s specie (g/100 g of total FA)
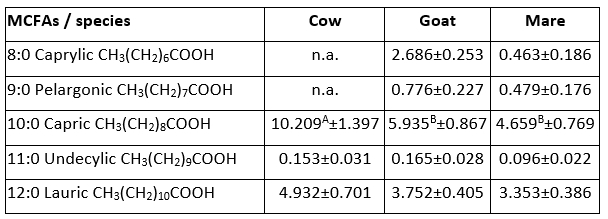
*MCFAs - medium-chain fatty acids; LsMeans in the same row marked with different letters (A,B) differ statistically highly significant (p<0.01)
The content of undecylic acid was highest in the milk of goats, with an average value of 0.165±0.028 g/100 g of total FA. In contrast, the lowest value of undecylic acid was observed in mare's milk, with an average value of 0.096±0.022 g/100 g of total FA. Another medium-chain fatty acid, lauric acid, was found to have the highest content in cow's milk, with an average value of 4.932±0.701 g/100 g of total FA. However, the milk of goats and mares also had similar values of lauric acid, with an average of 3.752±0.405 and 3.353±0.386 g/100 g of total FA, respectively. Furthermore, the findings of this study contradict those of another study conducted by Rodríguez-Alcalá et al. (2017), which found a higher content of lauric acid in the milk of goats (4.99 g/100 g of total FA) than in cows' milk (2.99 g/100 g of total FA). According to recent research conducted by Day et al. (2022), it has been found that undecylic acid exhibits anti-cancer properties and can be effective against several types of cancer. Additionally, other studies such as the one conducted by Poonam and team in 2020, have shown that lauric acid can induce apoptosis in cancer cells of diverse types, including leukemia (Chhikara et al., 2011), breast cancer, and endometrial cancer cells (Lappano et al., 2017). It has also been observed that goat milk has the highest concentration of undecylic acid, making it a potential source of this compound for those looking to incorporate it into their diet. Consumption of goat milk and its products may offer a beneficial effect on several types of cancer. On the other hand, cow milk has been found to contain high levels of lauric acid, which can have a positive impact on individuals with leukemia, breast cancer, and endometrial cancer when introduced into their diet.
Undecylenic acid, a widely used topical antifungal agent, has shown promising potential as an effective antitumor agent in recent research by Day et al. (2022). The study investigated a new formulation of undecylenic acid, called GS-1, which contains L-Arginine and was found to cause concentration-dependent death of tumor cells. The primary cytotoxic component of GS-1 is undecylenic acid, which triggers caspase-dependent cell death and reduces mitochondrial membrane potential, leading to a pro-apoptotic mechanism of action. Intriguingly, GS-1 was found to localize intracellularly to lipid droplets. These findings suggest that GS-1 has significant pro-apoptotic antitumor activity in vitro, and the innovative fatty acid solubilization platform contributes to the reemerging field of fatty acids as potential anti-cancer therapeutics.
Lauric acid (dodecanoic) is a saturated medium-chain fatty acid that has been shown to improve the quality of life in breast cancer patients undergoing chemotherapy. Recent research conducted by Lappano et al. (2017) sheds light on how LA triggers antiproliferative and pro-apoptotic effects in both breast and endometrial cancer cells. Their findings indicate that LA increases reactive oxygen species levels, stimulates the phosphorylation of EGFR (Epidermal Growth Factor Receptor), ERK (Extracellular Signal-Regulated Kinases), and c-Jun (c-Jun is a component of the AP-1 (Activator Protein-1) transcription factor complex), and induces the expression of c-fos. The EGFR/ERK/c-Jun signaling pathway is a complex mechanism that can play a vital role in the regulation of PD-L1 (Programmed Death-Ligand 1) expression - a protein that has been found to be crucial in the immune escape of tumors. Recent research studies have shown that the signaling pathway can be activated in response to glutamine deprivation in renal cancer cells, leading to an increase in PD-L1 expression levels. Specifically, in a study conducted by Ma et al. (2020), it was observed that the deprivation of glutamine in renal cancer cells resulted in the activation of the EGFR/ERK/c-Jun signaling pathway, which subsequently led to an upregulation of PD-L1 expression. Furthermore, other studies have also highlighted the role of EFEMP2 (EGF containing fibulin-like extracellular matrix protein 2) in the upregulation of PD-L1 expression through the same pathway in ovarian cancer cells. Specifically, a study conducted by Shen et al. (2023) found that EFEMP2 could activate the EGFR/ERK/c-Jun signaling pathway in ovarian cancer cells, leading to an increase in PD-L1 expression levels. Lappano et al. (2017) stated that LA also promotes stress fiber formation via the Rho-associated kinase-mediated pathway, which is crucial in the morphological changes associated with apoptotic cell death. Assiri et al. (2023) explored the potential of lauric acid-based hydrazones as anticancer and antioxidant agents. They synthesized various compounds and evaluated their activity, finding that these compounds were able to inhibit the oxidative chain reactions that lead to the production of reactive oxygen species, making them potential antioxidants. Among the synthesized compounds, (E)-N′-(4-fluorobenzylidene) dodecanehydrazide (FBDH) showed the highest anticancer activity, suggesting that it could be developed as a novel anticancer agent.
The variability in the concentration of ten LCFAs (myristic, pentadecylic, palmitic, margaric, stearic, nonadecylic, arachidic, behenic, tricosylic, and lignoceric) regarding the animal species is presented in Table 4. Although a statistically significant (p<0.01) difference was determined only for stearic acid, the composition of milk from different animal species has been found to differ in terms of fatty acid content.
Myristic acid (tetradecanoic) is a common saturated long-chain fatty acid that plays an essential role in myristoylation. This process is essential for membrane association and protein-protein interactions that are vital for cellular function and signaling. The pharmaceutical industry utilizes myristic acid as a precursor in the synthesis of various drugs, making it a crucial intermediate compound in drug development. The study conducted by Zeng et al. (2008) revealed that saturated fatty acids (SFAs), such as myristic acid, palmitic acid, and stearic acid, have a direct impact on the cellular response to DNA damage. These SFAs were found to compromise the induction of p21 and Bax expression, both of which are crucial proteins in the response to double-stranded breaks and single-stranded DNA. The p21 protein is an essential cyclin-dependent kinase inhibitor, while Bax is a pro-apoptotic protein that plays a crucial role in regulating cell cycle and apoptosis. Furthermore, this study discovered that the inhibition or knockdown of fatty acid synthase (FASN), which is an enzyme responsible for catalyzing the synthesis of long-chain fatty acids, enhanced the induction of p21 and Bax. This finding suggested that the synthesis of SFAs may play a crucial role in the cellular response to DNA damage.
Margaric acid (heptadecanoic), is a type of saturated long-chain fatty acid. This fatty acid has been the subject of various studies due to its potential health benefits, especially concerning cancer. According to research conducted by Effenberger et al. (2010), margaric acid has shown promising effects in the treatment of human leukemia, melanoma, breast, and cervix carcinomas. A recent study by Xu et al. (2019) indicates that margaric acid may also be beneficial in the treatment of lung cancer.
Regarding the myristic acid content, the highest amount was found in mares' milk (11.847±1.494 g/100 g of total FA), followed by cows' milk (11.373±2.714 g/100 g of total FA), and goats' milk (8.386±1.567 g/100 g of total FA). Research by Chhikara et al. (2010) and Zeng et al. (2008) has shown that myristic acid can have a beneficial effect on human leukemia, indicating that mares' and cows' milk may be beneficial for those suffering from leukemia. Similarly, pentadecylic acid content was found to be highest in goats' milk (2.900±0.362 g/100 g of total FA), followed by mares' milk (2.070±0.357 g/100 g of total FA), and cows' milk (1.131±0.648 g/100 g of total FA). Studies by To et al. (2020) have suggested that pentadecylic acid may have an anticancer effect on human breast carcinoma, indicating that goats' and mares' milk may be advantageous for those suffering from breast cancer.
Pentadecylic acid (pentadecanoic acid) is a saturated long-chain fatty acid with potential health benefits, including anticancer properties. Recent research (To et al., 2020) on the anticancer effects of pentadecylic acid has revealed its selective cytotoxic effects in human breast carcinoma MCF-7 stem-like cells, which are characterised by greater mobility, invasiveness, and cancer stem cell properties as compared to the parental MCF-7 cells. These findings suggest that pentadecylic acid may have a promising role in targeting cancer stem cells, which play a crucial role in tumor growth and metastasis.
The highest content of palmitic acid was found in cows' milk (24.785±1.593 g/100 g of total FA), followed by goats' milk (23.968±0.890 g/100 g of total FA), and mares' milk (23.639±0.877 g/100 g of total FA). Studies by Zhu et al. (2021) and Yu et al. (2023) have shown that palmitic acid may have beneficial effects for those suffering from prostate and gastric cancers. The margaric acid content was the highest in cows' milk (0.679±0.064 g/100 g of total FA) and the lowest in goats' milk (0.526±0.041 g/100 g of total FA). Studies by Effenberger et al. (2010) and Xu et al. (2019) have suggested that margaric acid may have a detrimental effect on human leukemia, melanoma, breast and cervix carcinomas, as well as lung cancer.
Table 2. LsMeans of long-chain fatty acids (LCFAs) regarding the animal’s breed (g/100 g of total FA)
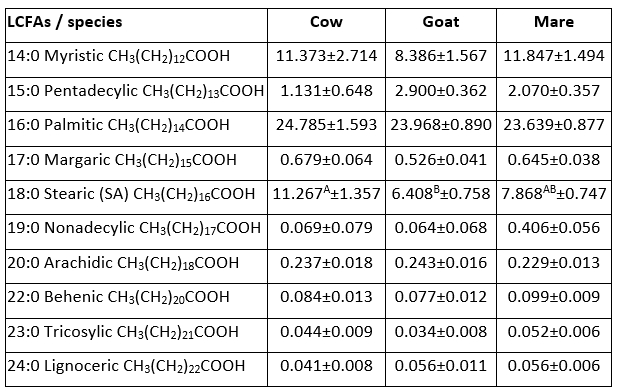
* LCFAs - long-chain fatty acids; LsMeans in the same row marked with different letters (A, B) differ statistically highly significant (p < 0.01)
This study revealed that cows' milk has the statistically significant (p<0.01) highest content of stearic acid (C16:0) amounting 11.267±1.357 g/100 g of total FA, whereas goats' milk showed the lowest content of 6.408±0.758 g/100 g of total FA. Rodríguez-Alcalá et al. (2017) obtained similar results reporting the content of 10.51 g/100 g of total FA of stearic acid in cows and 8.88 g/100 g of total FA in goat milk. The research conducted by Hardy et al. (2003) and Liu et al. (2009) has confirmed the importance of stearic acid, which has been found to inhibit the growth of breast cancer cells and induce breast cancer cell apoptosis. Liu et al. (2009) also noted the anticancer effect of stearic acid in a case of human leukemia.
Stearic acid (octadecanoic), a long-chain saturated fatty acid, has been identified as a substance with anti-cancer properties. The mechanisms through which it acts against cancer include inhibition of cancer cell growth, induction of apoptosis of breast cancer cells, and arrest of breast cancer cell cycle (Khan et al., 2013). Additionally, stearic acid has been encapsulated in solid lipid nanoparticles and studied for its anticancer and antidepressant effects (Khan et al., 2022). The cytotoxic analysis of naked and drug-encapsulated solid lipid nanoparticles on the U-87 cell line indicated that diosgenin encapsulation in nanoparticles slightly decreases toxicity. Stearic acid has also been found to inhibit the human cervical cancer (HOG-1) cell growth and DNA synthesis in a dose-dependent manner (Beesley et al., 1993). Hardy et al. (2003) found that stearic acid can have an anticancer effect on breast cancer. Similarly, Liu et al. (2009) reported that stearic acid can also have an anticancer effect on human leukemia. These findings suggest that stearic acid holds potential as a therapeutic agent for cancer.
The content of nonadecylic acid (C18:0) was the highest in the milk of mares with a value of 0.406±0.056 g/100 g of total FA, while goats' milk showed the lowest value of 0.064±0.068 g/100 g of total FA.
Nonadecylic acid (nonadecanoic), is a type of saturated faty acid with an odd number of carbon atoms. It can be found in insignificant amounts in dairy products, certain fish, and plants. According to Venn-Watson et al. (2020), higher levels of two specific odd-chain saturated fatty acids, pentadecanoic acid (C15:0) and heptadecanoic acid (C17:0), are associated with a lower risk of cardiometabolic diseases, and increased intake of these fatty acids has been linked to a lower mortality rate.
The content of arachidic acid (C20:0) was relatively similar in all the species, with goats showing slightly higher values of 0.243±0.016 g/100 g of total FA and mares showing slightly lower values of 0.229 ± 0.013 g/100 g of total FA. The content of behenic acid (C22:0) was also similar in all the species with mares showing the highest value of 0.099±0.009 g/100 g of total FA and goats showing the lowest value of 0.077±0.012 g/100 g of total FA. Arachidic acid has been found to possess antioxidant and antineoplastic properties (Hasi et al., 2019). Kumari et al. (2012) reported that arachidic acid has anti-inflammatory and anti-cancer effects.
Arachidic acid (eicosanoic), is a type of saturated long-chain fatty acid that contains a 20-carbon chain. Although arachidic acid itself is not usually associated with anticancer properties, recent research has been conducted on the potential anticancer effects of fatty acids and their derivatives (Hasi et al., 2019). This study analysed the roots of Hibiscus sabdariffa and found out that a methanolic extract, which had arachidic acid as a major component, exhibited promising antioxidant and antineoplastic efficacy against Ehrlich ascites carcinoma cells. This was achieved by inducing apoptosis, a programmed cell death process, which is commonly used to kill cancer cells. These findings suggest that arachidic acid may have a potential as a therapeutic agent against cancer.
Nath et al. (2022) emphasized that behenic acid has the potential to decrease cancer prevalence, whereas Kaplan et al. (2013) suggested that behenic acid could be used as an indicator of glial tumors. The levels of tricosylic (C23:0) and lignoceric (24:0) acids were found to be relatively low across all the studied milk samples. The amount of tricosylic ranged from 0.034±0.008 g/100 g of total FA in goats to 0.052±0.006 g/100 g of total FA in mares, while the levels of lignoceric varied from 0.041±0.008 g/100 g of total FA in cows to 0.056±0.006 g/100 g of total FA in goats and mares.
Behenic acid (docosanoic acid), is a saturated long-chain fatty acid comprising a 22-carbon chain. Although it is not commonly recognized for its anticancer properties, behenic acid has been examined for its potential health advantages, including its capacity to decrease inflammation, enhance cholesterol levels, and maintain healthy skin. Nath et al. (2022) have suggested that it could aid in reducing the likelihood formation of specific types of cancer, improve cognitive function, and lower the risk of heart disease. Kaplan et al. (2013) reported that elevated levels of behenic acid have been observed in individuals diagnosed with low-grade glial tumors. This observation is valuable as it implies the persistence of tissue integrity and resistance. Behenic acid levels may serve as a prognostic indicator in the case of glial tumors.
Heneicosylic acid (heneicosanoic), is a type of saturated long-chain fatty acid composed of a 21-carbon chain. According to Kumari et al. (2012), this acid has shown potential biological properties including anti-inflammatory, anti-cancer, and anti-microbial activities, making it a promising candidate for therapeutic applications. It has been found to exhibit inhibitory effects on the growth of various bacterial and fungal strains, which may be useful in the development of novel antibiotics. The anti-inflammatory and anti-cancer properties of heneicosanoic acid suggest that it could be a potential treatment for a range of diseases.
This study has identified two distinct groups of long-chain fatty acids (LCFAs) in milk based on their concentrations in the overall FA profile. The first group, found to have the highest concentration are myristic, palmitic, and stearic acid, ranging from 6.408 to 24.785 g/100 g of total FA across all three species. These findings are consistent with previous research done by Rodríguez-Alcalá et al. (2017). The high levels of LCFAs in this group have been linked to potential anti-cancer properties, particularly in the prevention and treatment of human leukemia, prostate, gastric, and breast cancer, as supported by various studies including those of Hardy et al. (2003), Liu et al. (2009), Zeng et al. (2008), Chhikara et al. (2010), Zhu et al. (2021), and Yu et al. (2023). On the other hand, the second group are LCFAs with the lowest concentration in the overall FA profile across all three species. This group consists of tricosolyc and lignoceric acids, with a range of 0.034 to 0.056 g/100 g of total FA.
Palmitic acid (hexadecanoic) is a saturated long-chain fatty acid commonly found in dairy products and palm oil. Recent research has explored its potential anti-tumor properties, which appear to occur through multiple mechanisms (Zhu et al., 2021; Yu et al., 2023). Palmitic acid (PA) can promote cell death in tumor cells by generating reactive oxygen species (ROS) and activating the mitochondrial pathway. Additionally, it can interfere with the cancer cell cycle, leading to cell cycle arrest, particularly in the G1 phase. PA has also been shown to inhibit cell migration, invasion, and angiogenesis, which are critical processes for tumor growth and metastasis. Finally, PA has the potential to enhance the efficacy of chemotherapy drugs while reducing adverse reactions.
Ruminants' fermentative capacity can vary depending on the type of ruminant species and the substrate fermented. In addition, the milk of cows, goats, and mares differs in the total fatty acid (FA) profile, including the content of medium and long-chain fatty acids. Some studies have highlighted that the FA composition of milk varies among different species and is strongly influenced by animal nutrition (Miller, 1987; Kompan and Komprej, 2012; Gantner et al., 2015; Jastrzębska et al., 2017; Rodríguez-Alcalá et al., 2017; Mollica et al., 2021). Furthermore, according to Linn (1988), milk fat content is affected by several factors, including breed/genetics, environment/management, health/physiology, and nutrition. These factors influence the composition of fatty acids in milk, as approximately 50 % of the fatty acids in milk are synthesized in the mammary gland (short-chain acids). The remaining 50 % are derived directly from blood (long-chain acids), while intermediate-chain acids arise from both sources.
Conclusions
The obtained results suggest that the concentration of medium and long-chain saturated fatty acids in milk varies depending on the animal species. Specifically, goats' milk was found to have a high concentration of caprylic acid, which has been linked to potential benefits in the prevention of colorectal, skin, and breast cancer in humans. On the other hand, cows' milk was found to have a high concentration of capric and lauric acid, both of which have been associated with potential beneficial effects against several types of cancer. The study also revealed that all three milk types (cows, goats, and mares) had high concentrations of long-chain fatty acids (LCFAs), specifically myristic, palmitic, and stearic acid, with cows' milk having the highest value. These fatty acids have been linked to potential beneficial effects in the prevention of various types of cancer in humans, including leukemia, prostate cancer, gastric cancer, and breast cancer.
Acknowledgements and funding
Research and dissemination were supported by the Fund for Bilateral Relations within the Financial Mechanism of the European Economic Area and Norwegian Financial Mechanism for the period 2014-2021 (Grant number: 04-UBS-U-0031/23-14).
References
Effenberger, K., Breyer, S., Schobert, R. (2010): Modulation of doxorubicin activity in cancer cells by conjugation with fatty acyl and terpenyl hydrazones. European Journal of Medicinal Chemistry 45 (5), 1947-1954. https://doi.org/10.1016/j.ejmech.2010.01.037
El-Gawad, A.M.A., El-Hassan, D.G.A., Aboul-Enein, A.M., Abdelgayed, S.S., Aly, S.A., Esmat, G., Mostafa, A.A., Bakr, M.H., Ali, R.A., Ayoub, M.A. (2021): Anticancer activity of milk fat rich in conjugated linoleic acid against Ehrlich ascites carcinoma cells in female Swiss albino mice. Veterinary World 14 (3), 696-708. https://doi.org/10.14202/vetworld.2021.696-708