Introduction
Yoghurt is a dairy product obtained through fermentation with yoghurt culture consisting of strains Lactobacillus delbrueckii subsp. bulgaricus and Streptococcus thermophilus, and is a widely consumed food internationally due to its nutritional value, health benefits and digestibility (Nguyen et al., 2017). In recent years, there is significant demand for low fat-dairy products with the same properties as full-fat products due to rising of cardiovascular diseases, obesity, cancer and diabetes (Rudra et al., 2017). Thus, low fat yoghurt has become quite popular in the market (Nguyen et al., 2017). Milk fat in dairy products contributes to overall flavour, mouthfeel, body, and texture of the dairy products (Levy et al., 2022). However, the removal of fat in yoghurt leads to undesirable changes in physical and sensory properties such as low viscosity, weakened texture, syneresis, poor body and undesirable mouthfeel (Torres et al., 2018) that is not desired by consumers. Consequently, alternative processes such as microfluidization, thermosonication, high pressure processing and high-pressure homogenization have been proposed for implementation in the production of good quality reduced-fat yoghurts recently (Ciron et al., 2012; Oliveira et al., 2014; Ragab et al., 2021).
High-pressure homogenization is an emerging and relative new technology, which is used to improve quality characteristics of dairy products up to 400 MPa pressure (Loveday et al., 2013; Massoud et al., 2016). When considering conventional homogenization, 15-60 MPa pressure are usually applied in order to ensure homogeneous distribution of milk fat, improve the consistency and stability of the product, achieve whiter colour and reduce the amount of serum separated from the yoghurt (Krebs et al., 2021). High-pressure homogenization process affects the size of milk fat globules, proteins, and polysaccharides, but it also causes the modification of their functional properties. Furthermore, high-pressure homogenization leads to improved properties within yoghurt matrix due to smaller and more uniform fat globules with increased surface area that incorporate into the milk proteins, casein, and denatured whey during gel formation (Krebs et al., 2021; Loveday et al., 2013; Massoud et al., 2016). Additionally, shelf-life of the products is improved by high pressure homogenization which lowers the creaming rate (Serra et al., 2007).
In the literature reviews, it has been observed that there have been very limited studies with high-pressure homogenization, especially in the production of fermented milk products such as low-fat yoghurt. Krebs et al. (2021) stated that high pressure homogenization significantly affects the milk proteins and, thus results in positive changes of the gel microstructure, water holding capacity and textural properties of set-type and stirred yoghurts. On the contrary, Serra et al. (2008) stated that yoghurts from high pressure homogenized milk (up to 300 MPa pressure) had lower firmness and higher syneresis than control sample probably due to much lower β-lg denaturation in homogenized milks. In comparison to the conventional yoghurts, higher water holding capacity and firmness values for yoghurts manufactured from ultra-high pressure homogenization-treated milk have been reported Serra et al. (2009). Patrignani et al. (2007) also found that the homogenization pressure strongly affects some characteristics of fermented milks including coagulation time and rheological parameters. Consequently, the results of the several past studies about the effects of high pressure homogenization differ significantly and more research on this matter is needed. Therefore, the aim of this study was to evaluate the effect of high-pressure homogenization process up to 150 MPa on the acidification kinetic, rheological, textural, microstructural, and sensorial properties of low-fat yoghurts from milk containing 2% milk fat.
Materials and methods
Preparation of low fat milk and homogenization
Raw cow milk (6.71 pH, 11.1 % total solids, 3.6 % fat, 3.11 % protein, and 0.7 % ash) was directly obtained from a local dairy factory (Malkocoglu Dairy Co., Edirne, Turkey) and transported to laboratory at 4oC. To obtained low fat milk (6.59 pH, content 10.1 % total solids, 1.95 % fat, 3.03 % protein and 0.71 % ash), raw milk was skimmed at 55 °C using a cream separator (SMS Ltd. Co., Kayseri, Turkey) to approximately 0.2 % fat content and milk was standardized to 2 % fat content according to the Pearson square method. Then, low fat milk was treated by high pressure homogenizer (GEA Niro Soavi, Panda Plus 2000, Parma, Italy) with pressure set at 50, 100 and 150 MPa for one cycle. Homogenization of milk was done at 55 °C as the initial temperature, and the temperature of milk after homogenization was measured by a thermometer that was determined between 53 and 61 °C depending on homogenization pressure. A non-processed milk was set as the control sample.
After the high-pressure homogenization, the low fat milk samples were heat-treated at 90 °C for 5 min using a water bath (WBN 22, Memmert, Schwabach, Germany) and then cooled in an ice bath to fermentation temperature (45 °C). The processed milk samples were inoculated with YC-X11 starter culture containing Streptococcus thermophilus and L. delbrueckii subsp. bulgaricus (Peyma-Chr., Hansen’s Co., Istanbul, Turkey) and incubated at 43 °C until pH reached to 4.6±0.03. At the end of fermentation, yoghurt samples were cooled to room temperature, lids were closed, and they were kept in a refrigerate environment at +4 °C until instrumental testing.
Acidification activity
Acidification activity was determined by the changes in the pH during fermentation, which was monitored continuously by pH meter (Seven2Go, Mettler Toledo, Greifensee, Switzerland) until the pH 4.6. Maximum acidification rate ( Vmax ) was calculated as the time variation of pH ( dpH/dt) and expressed in absolute values. The time to reach the maximum acidification rate ( Tmax ), and time to complete the fermentation ( tf ) were determined as responses that characterized the kinetics of fermentation process.
Proximate analysis
Titratable acidity, pH, dry matter, fat, protein and ash content of milk and yoghurt samples were determined according to standard methods (AOAC, 2007). pH values of samples were measured at room temperature using a pH meter calibrated with standardized buffer solutions (pH 4.0, 7.0 and 10.0). Titratable acidity was determined by a titration method using 0.1 M NaOH and the results were expressed as lactic acid percentage. To convert nitrogen percentage to protein percentage, a multiplication factor of 6.38 was used.
Colour analysis
The colour properties of yoghurt samples were measured using a colorimeter (Minolta Chrome Meter, Tokyo, Japan) with CIELAB system using D65 light source. A white colour plate ( L*:97.81, a*: -0.19 and b*:1.88) was used as a standard background. L* (lightness), a* (green-red) and b* (blue-yellow) were determined by taking an average of six reading from each yoghurt sample. The colour difference (ΔE) and whiteness index (WI) were calculated by using Eq. 1 and 2.

where Δ L, Δ a, and Δ b refer the difference between each colour value of yoghurt samples and white colour plate.
Water holding capacity (WHC)
WHC of the yoghurt samples was determined using the method described by Harte et al. (2003) with a slight modification. Five grams of yoghurt samples were subjected to 20 min centrifugation at 3250 x g at 4 °C. The percentage of water holding capacity was calculated following equation (Eq. 3):

where: W1 refers the weight of whey after centrifugation and W2 refers the yoghurt weight.
Texture profile analysis (TPA)
TPA was done by using a TA.HD Plus Texture Analyser (Stable Micro Systems, Surrey, UK) equipped with a probe with back extrusion rig containing a compression disc of 45 mm diameter and extension bar having 5 kg load cell. The test was replicated three times at a pre-test speed of 1 mm/s, test speed of 2 mm/s and a depth of 20 mm into the samples. Samples were tested in their cups after the temperature was allowed to equilibrate at 20 °C. Yoghurt samples were evaluated for firmness, adhesiveness and cohesiveness values.
Rheological analysis
The rheological measurements of yoghurt samples were conducted by using a Haake Mars III rheometer (Thermo Scientific, Germany) equipped with a Peltier temperature controller and a cone-plate geometry with a plate of a 35 mm diameter and 2o cone angle. The samples poured into the cup after gently stirred in a standardized way and samples were held 2 min at 8 °C to equilibrate the temperature. Measurements were performed by recording shear stress against shear rate range of 1 - 100 s-1 at 8 °C. Ostwald de-Waele model was used to describe the flow behaviour by using the following equation (Eq. 4).
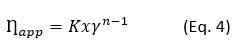
where ηapp, K, γ and n refer the apparent viscosity (Pa.s), the consistency index (Pa.sn), the shear rate (s−1) and the flow behaviour index (dimensionless), respectively.
Microstructure
The microstructural properties of yoghurt samples were evaluated using scanning electron microscope (Zeiss Evo LS 10, Oberkochen, Germany) at an accelerating voltage of 15 kV. Prior to analysis, yoghurt samples were frozen at -20 °C and freeze-dried at -50 °C at a constant low pressure of 0.13 mbar about 48 h. After the dried samples powdered and coated with a thin film of gold, the microstructures of the samples were analysed via SEM at a magnification of 5000X.
Sensory analysis
The sensory evaluation of yoghurt samples was carried out using a sensory evaluation scorecard modified by Bodyfelt et al. (1988). The evaluation was performed in duplicate by a trained panellist group of 10-15 people, including stuff and students (ages 20-55 years) from the Department of Food Engineering, Faculty of Engineering, Trakya University. Previous experience in evaluating products and ability to access and rate the sensory parameters differences were taken into account for selection of the panellists. The points required to be given according to the perceived errors in the sensory evaluation of the samples were given to the panellists in a separate chart and they were asked to score accordingly. The samples were randomly distributed in each sensory evaluation. For sensory evaluation, samples were presented in 200 g containers at 15 °C. In order to better perceive the aroma difference between the samples, the panellists made an evaluation in the presence of water. Taste-aroma and general acceptability were made out of 10 points, structure-texture and appearance-colour were made out of 5 points. The sensory properties were described numerically by a structured ten or five-point hedonic scale ranging from like extremely to dislike extremely.
Statistical analysis
Three independent productions of low-fat yoghurt were conducted, and analysis were carried out for each replicate. Data analysis were done using SPSS statistical package program version 21.0 (SPSS Inc., Chicago, IL, USA). The variance between the means was assessed by one-way ANOVA and the differences among the means were evaluated using Duncan test with a significance level of p<0.05. Principal component analysis was performed using XLSTAT version 2015 (Addinsoft USA, New York, USA) in order to visualize the effect of observations.
Results and discussion
Acidification activity and pH and titratable acidity values of yoghurts
The pH values during fermentation of milk untreated (control) and treated using high-pressure homogenization are shown in Figure 1 and the acidification kinetics were characterized by Vmax, Tmax and Tf (Table 1).
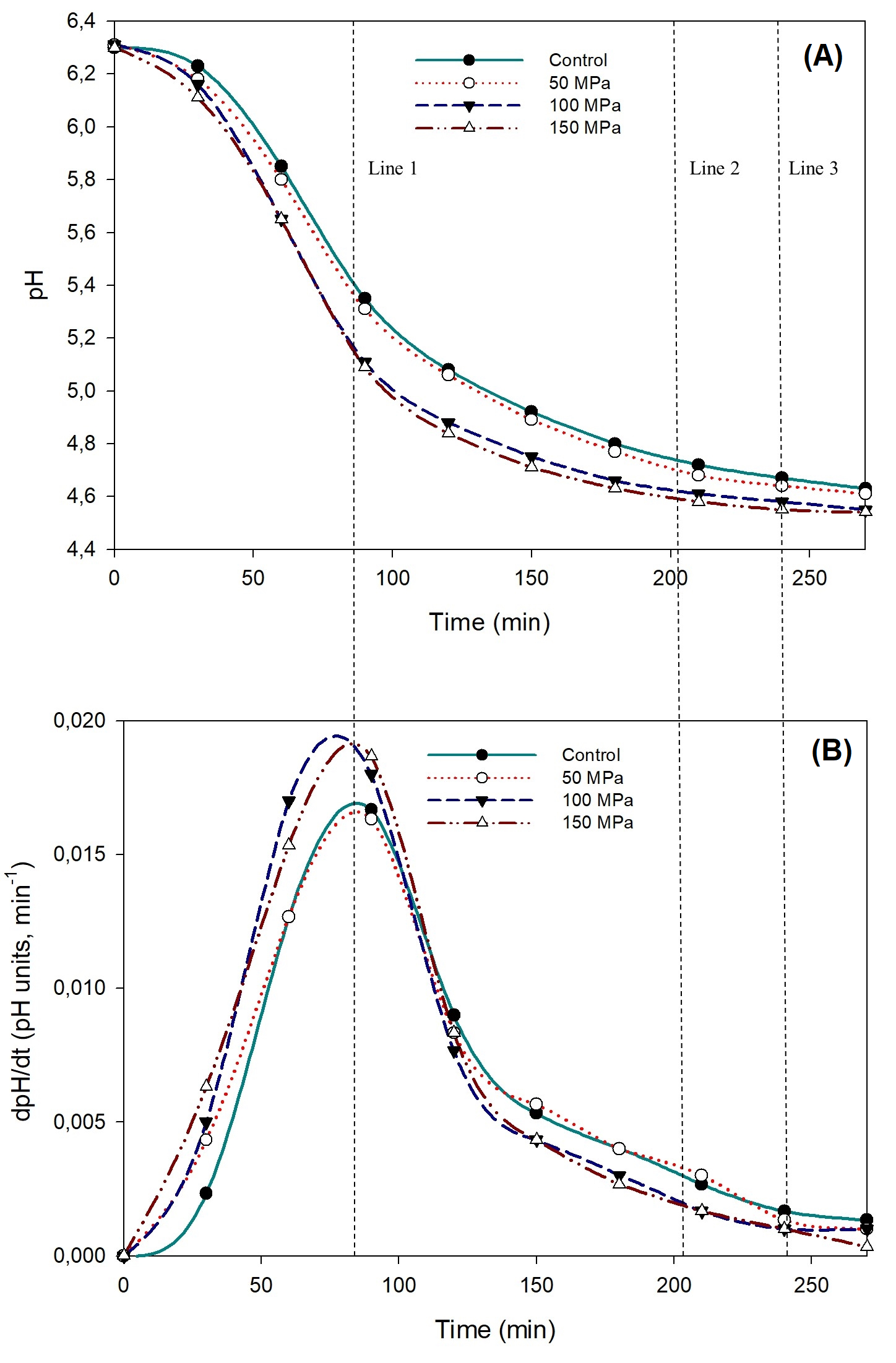
Figure 1. Fermentation kinetics through pH for low-fat yoghurt samples made from unhomogenized (control) and homogenized milk at 50, 100 and 150 MPa pressure
Table 1. Acidification kinetic parameters and physicochemical properties of low-fat yoghurt samples made from unhomogenised (control) and homogenized milk at 50, 100 and 150 MPa pressure
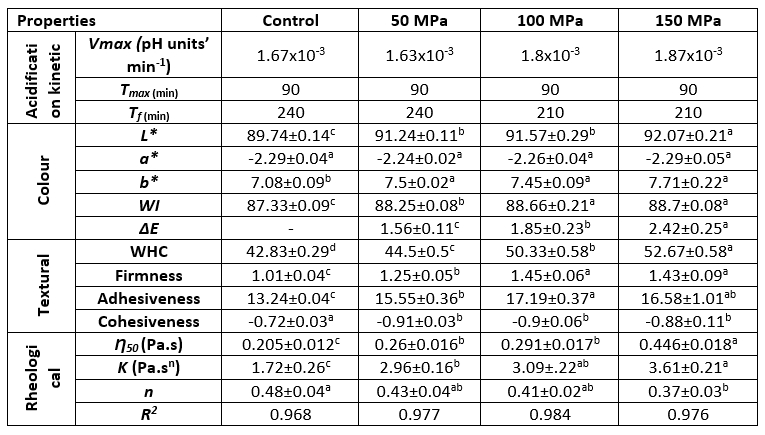
a, b, cMeans in the same row with different lowercase letters show significant differences between samples (p<0.05). Vmax: Maximum rate of acidification; Tmax: Time to reach Vmax; Tf: Time to reach pH 4.6; WI, whiteness index; ΔE, colour difference; WHC, water holding capacity; ηapp, apparent viscosity; K, consistency index; n, flow behavior index
Although the fermentation kinetics of yoghurts obtained from untreated milk and milk treated at 50 MPa was not influenced by homogenization process, the difference in fermentation kinetics of starter culture in samples after milk homogenized at 100 and 150 MPa was observed (Figure 1b). This observation corresponds to the results obtained by Serra et al. (2007) showing difference in the kinetics of acidification in high pressure homogenized milk compared to the control and by Lanciotti et al. (2004) showing accelerated decrease in pH by the increase in homogenization pressure that maximized the starter culture growth. On the contrary, Oliveira et al. (2014) stated that the fermentation kinetics is not affected by the dynamic high pressure.
The Vmax values of samples were determined in the range of 1.63 x10-3 - 1.87 x10-3 pH units min-1. While Vmax exhibited little reduction by applied homogenization at 50 MPa compared with control samples, it was increased by the increase of homogenization pressure. In addition, the starter culture in control samples has a longer initial adaptation (lag period) as shown in Figure 1b, which is characterized by a low acidification rate. After this period, acidification rate increased rapidly in a pH range between 6.23 and 5.09 (Figure 1b, line 1) and reached a maximum value of 1.87 x10-3 pH units min-1 for samples produced with milk homogenized at 150 MPa. After the pH values approximately 5.1, acidification rate decreased probably due to low metabolic activity of S. thermophilus (Galia et al., 2009).
According to the fermentation time shown in Figure 1b, although the time to reach maximum acidification rate ( Tmax ) was found similar for all samples, time to reach pH 4.5 ( Tf ) was significantly affected by homogenization process. Tf value of control and yoghurt sample produced milk after homogenized at 50 MPa was found similar as 240 min (Figure 1b, line 3). Homogenization process led to a decrease in the Tf value, and the shortest value was detected as 210 min for yoghurt samples produced after milk homogenization at 100 and 150 MPa pressure (Figure 1b, line 2). The reduction in the fermentation time of milk inoculated with yoghurt culture is probably due to changes in the distribution of soluble and insoluble calcium, phosphorus, and nitrogen resulting from the milk treatment (Patrignani et al., 2016).
Similar results were obtained by Nguyen et al. (2015) who stated that fermentation time for yoghurts was slightly shorter in homogenized milk samples, which could probably be explained by the better availability of nutrients released from the milk fat globule membrane that is disrupted by homogenization. In another study conducted by Serra et al. (2007) who found that the time reach pH 4.6 in control milk is longer than milks after homogenized at higher pressure than 200 MPa. Lanciotti et al. (2007) also reported the acidification time of L. pentosus and L. plantarum significantly reduced with the homogenization pressure level up to 150 MPa or two cycles at 50 MPa. However, Oliveira et al. (2014) reported that Tf did not change with the homogenization pressure. This difference in behaviour could be explained by milk composition, starter culture type and concentration as well as by the fermentation temperature employed in the manufacture of fermented milks.
At the end of the 240 min fermentation period for low-fat yoghurt production, pH value of yoghurt samples was found between 4.47 and 4.55. Although homogenization process clearly caused to drop the pH values compared control sample (p<0.05), a noticeable change in pH was not observed as a function of the applied pressure (p>0.05). On the other hand, an increase in titratable acidity was detected, but not significant (p>0.05).
Colour properties
Colour properties including L*, a*, b*, WI and ΔE values of yoghurt samples are shown in Table 1. Colour properties (except a* value) of low-fat yoghurt samples significantly influenced by milk homogenization (p<0.05). As expected, it was observed that an increase in homogenization pressure significantly increased the L* values of samples, and yoghurt obtained after milk homogenization at 150 MPa was whiter than other samples. The findings from this study are consistent with those from Hayes and Kelly (2003), showing an increment in L* value in homogenization treatment up to 200 MPa. This phenomenon could probably be explained by the increase in the number of fat globules in homogenized milk, which can diffract light more effectively (Amador-Espejo et al., 2014). Although homogenization treatment caused an increase in the b* values of samples, no statistically significant effect was observed among the pressure levels (p>0.05). The observed WI values of samples changed in accordance with L* values, which increased with increasing homogenization pressure. L* and b* values of milk homogenized at different pressures, significantly increased the colour difference ( ΔE) values of low-fat yoghurt samples (p<0.05), and the differences was visually obvious.
Water holding capacity (WHC) and textural properties
WHC, is one of the most important structural properties affecting yoghurt quality and shelf-life, is indicate the ability of the yoghurt structure to retain water, which must be as high as possible to obtain stable yoghurt gel network (Krebs et al., 2021; Levy et al., 2021). As observed in Table 1, control samples displayed the lowest (42.83 %) WHC value, while the highest WHC (52.67 %) was obtained for yoghurt produced after milk homogenization at 150 MPa. The increase in the homogenization pressure was accompanied by the progressive increase in the WHC values (p<0.05). Improving of WHC of yoghurt samples by using homogenized milk could be a result of increased particle number (casein micelles, fat globules etc.) produced during high pressure homogenization and the quantity of denatured whey proteins that may interact with one another (Ciron et al., 2010). According to Tamime and Deeth (1980) homogenization process leads to an increase in hydrophilicity and WHC due to casein fat globule membrane interaction and other protein-protein interactions, which is responsible for enhancing texture, including viscosity, firmness and water retention in yoghurt. In comparison to those made from conventionally treated milk, Serra et al. (2009) observed that syneresis of yoghurt decreased after homogenizing milk at pressures up to 200 MPa, and our results were similar to this observation. Similarly, Loveday et al. (2013) noted that acid gels with increased rigidity, breaking strength and improving WHC could be obtained by applying high pressure homogenization, due to the change in protein chemistry and interactions.
Texture profile analysis revealed that textural properties of yoghurt samples depend on applying homogenization pressure and the textural results of yoghurt gels are shown in Table 1. Firmness, adhesiveness and cohesiveness, which represents the gel networks strength, the force required to remove the material that adheres to a surface and the strength of the internal bonds that make up the texture of the yoghurt, respectively (Krebs et al., 2021; Najgebauer-Lejko et al., 2015), were monitored in order to complete the low-fat yoghurt characterization. In this study, it was observed that high pressure homogenized milk at pressures up to 100 MPa led to significant increase in firmness and adhesiveness of yoghurt samples (p<0.05), and then textural properties were not significantly affected by pressure (p>0.05). The cohesiveness of control sample (-0.72) was significantly different from samples produced after milk homogenization (p<0.05), however, no difference between pressure levels was obtained (p>0.05). The highest gel firmness in yoghurt produced after milk homogenization at 100 MPa is likely associated with the modification affected by high-pressure homogenization on casein-casein or casein-fat interactions, and the variation of the balance between insoluble and soluble forms of minerals such as calcium, phosphorus, and nitrogen (Lanciotti et al., 2004; Patrignani et al., 2007; Swelam, 2018). Our results agreed with the study by Serra et al. (2007) who showed that firmness of yoghurt samples increased by increasing homogenization pressure up to 300 MPa, which can be attributed to the whey protein denaturation, protein-protein interactions and fat–protein interactions. All of these events may result in enhanced particle interactions and the development of a gel network that is stable and capable of potent water absorption. Conversely, this tendency is in contrast with the results of yoghurts obtained by Krebs et al. (2021) and Patrignani et al. (2007), who noted that homogenization pressure is not impact firmness, and by Serra et al. (2008), who stated that yoghurts from high pressure homogenized milks have lower firmness. This difference in textural properties of yoghurts could be attributed by the type of milk used, system used for high-pressure homogenization, pressure applied before or after heat treatment (Ciron et al., 2010).
Rheological properties
The viscosities of low-fat yoghurt samples after fermentation are presented in Figure 2.
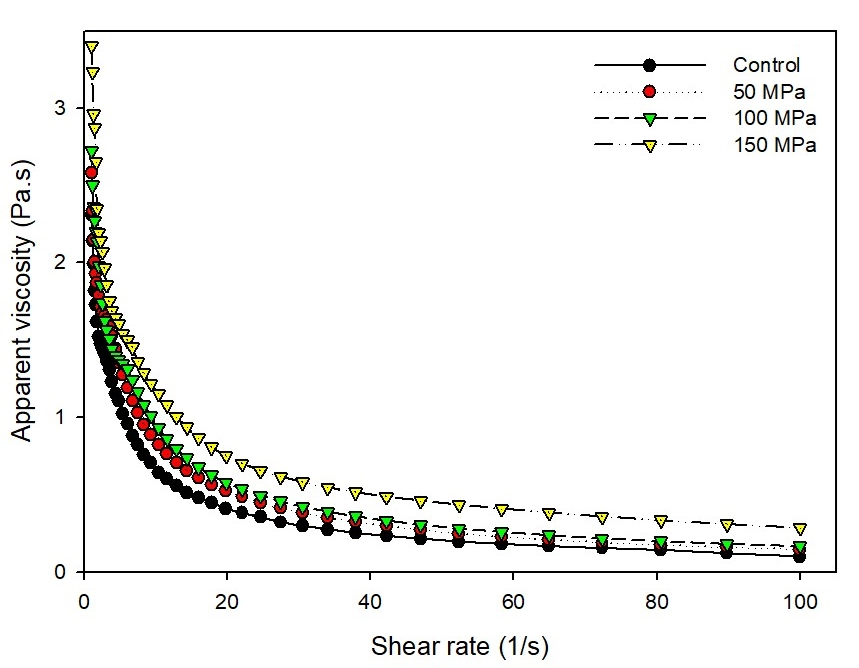
Figure 2. The flow behaviour of the low-fat yoghurt samples made from unhomogenized (control) and homogenized milk at 50, 100 and 150 MPa pressure
According to the flow curves of samples, viscosity decreased with increasing shear rates from 0.1 to 100 s-1, indicating all yoghurt samples exhibit shear thinning behaviour, regardless of the processing treatment, which is typical for non-Newtonian pseudoplastic materials (Torres et al., 2018). The viscosity values (Ƞ50) of yoghurt samples were recorded at the specified shear rate of 50 s-1, which has been suggested to have a good correlation with sensory perception analysis of thickness, stickiness and sliminess (Nguyen et al., 2017). The Ƞ50 values of yoghurt samples were determined between 0.205 and 0.446 Pa.s and significance difference were observed (p<0.05). The Ƞ50 values of low fat yoghurt samples obtained from milk treated with high-pressure homogenization process was found higher than control sample. Additionally, increasing homogenization pressure up to 150 MPa resulted in a considerable increase in Ƞ50 values (p<0.05) and the highest Ƞ50 value was determined for yoghurt sample obtained after milk homogenization at 150 MPa. This could be due to strong gel formation in the yoghurt sample treated higher homogenization pressure compared to other samples. The increment of viscosity values depend on homogenization pressure could be related with the production of insoluble co-aggregates with high molecular weight due to denaturation of whey protein during high pressure homogenization (Massoud et al., 2016). A similar observation was found by Needs et al. (2000) who stated that stronger gel network obtains with used high pressure homogenized bovine milk, which is probably attributed to greater whey proteins denaturation. In addition, particles in milk increases as a consequence of casein micelles partial disintegration during high pressure homogenization and could contribute the formation of networks, resulting in a finer network of smaller casein micelles in a three-dimensional yoghurt network structure (Serra et al., 2008).
For describing the flow curves of low-fat yoghurt samples, Ostwald de-Waele model was used and the regressed parameters of model with Ƞ50 values are shown in Table 1. Ostwald de-Waele model was successfully described the flow behaviour of low-fat yoghurt samples due to higher R2 values than 0.968. Values from yoghurt samples showed that homogenization pressure had a substantial impact on K and flow behaviour index ( n) values (p<0.05). K value of yoghurt samples increased from 1.72 to 3.61 Pa.sn by increasing homogenization pressure, while n values decreased from 0.48 to 0.37. This indicates more shear thinning behaviour with higher viscosity and higher yield stress of low fat yoghurt obtained after milk homogenization at 150 MPa pressure than that control sample. Similar results determined by Ciron et al. (2011) for yoghurt sample produced from milk homogenized by microfluidizer.
Microstructural properties
The microstructural properties of low-fat yoghurt samples were obtained by scanning electron microscopy (SEM) at magnification of 5000X (Figure 3).
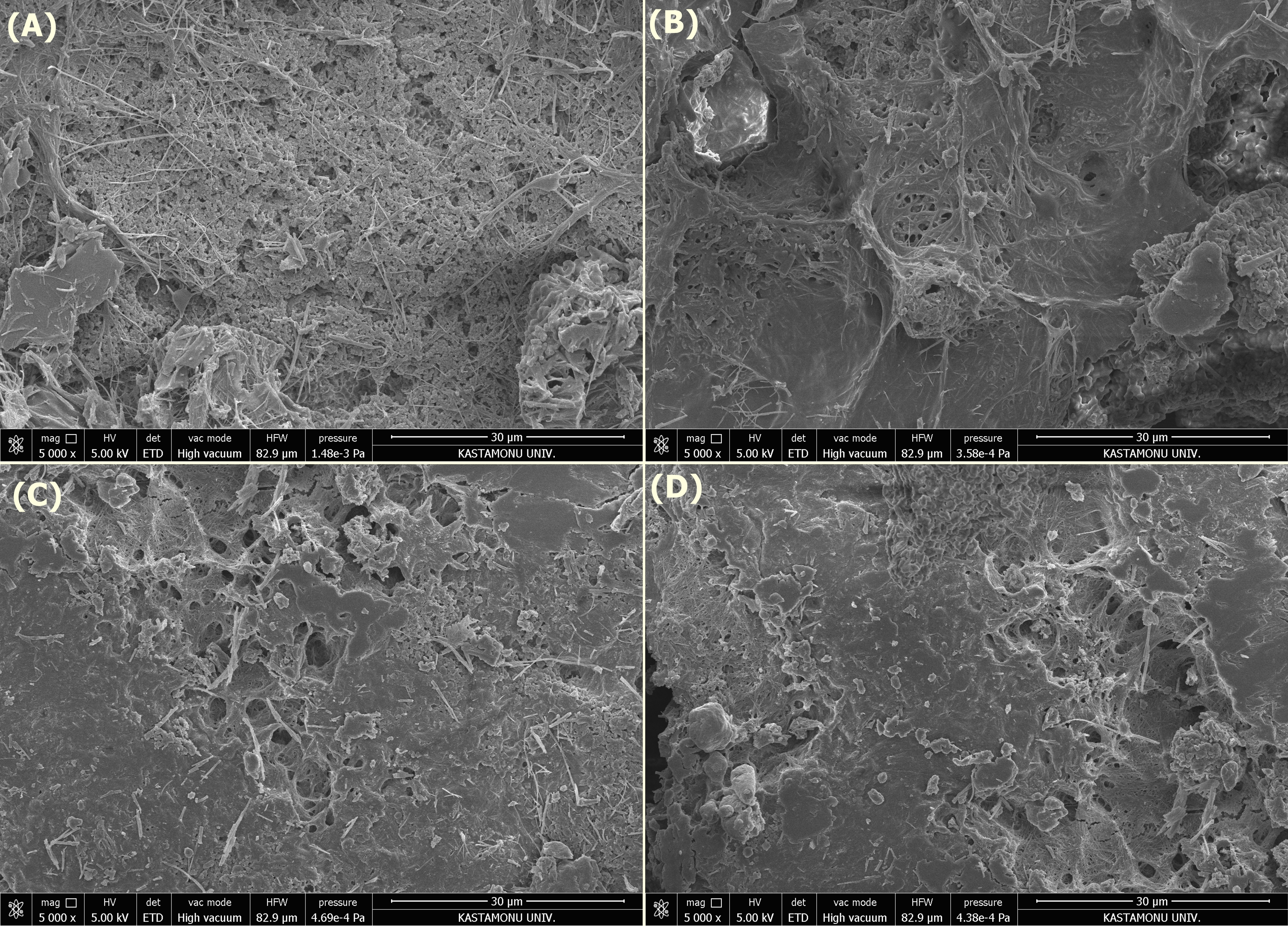
Figure 3. Microstructure of the low-fat yoghurt samples. A; control sample, B, C and D; low-fat yoghurt produced with homogenized milk at 50, 100 and 150 MPa, respectively. Images were captured at 5000x magnification
Noticeable differences between the microstructure of yoghurt samples were observed. Control sample displayed typical open porous and homogenously distributed casein gel network (Figure 3a), which is in agreement with the findings of previous studies (Khubber et al., 2021). Homogenization treatment with different pressure led to change the morphology of yoghurt samples. The protein network produced from homogenized milk showed fewer porous but heterogeneous microstructure and more dense protein network with compact structure compared to control sample, which may increase consistency of gel and improve water retention capacity (Oliveira et al., 2014). The thicker protein and compact structure observed in the microstructure of yoghurt samples made from homogenized milk are consistent with the textural properties. Large serum porous in the certain areas of the surface were observed by increasing homogenization pressure after 50 MPa (Figure 3c-d). Similar results were also reported in set yoghurt samples manufactured with homogenized milk (Ragab et al., 2021). On the other hand, although small aggregates observed in yoghurt samples from milk homogenized at 150 MPa, a marked difference in the microstructure and morphology did not observe between the samples from milk homogenized at 100 and 150 MPa.
Sensory properties
Sensory properties including taste-aroma, structure-texture, appearance-colour, and overall acceptability were evaluated after yoghurt manufacturing and the results are given in Figure 4.
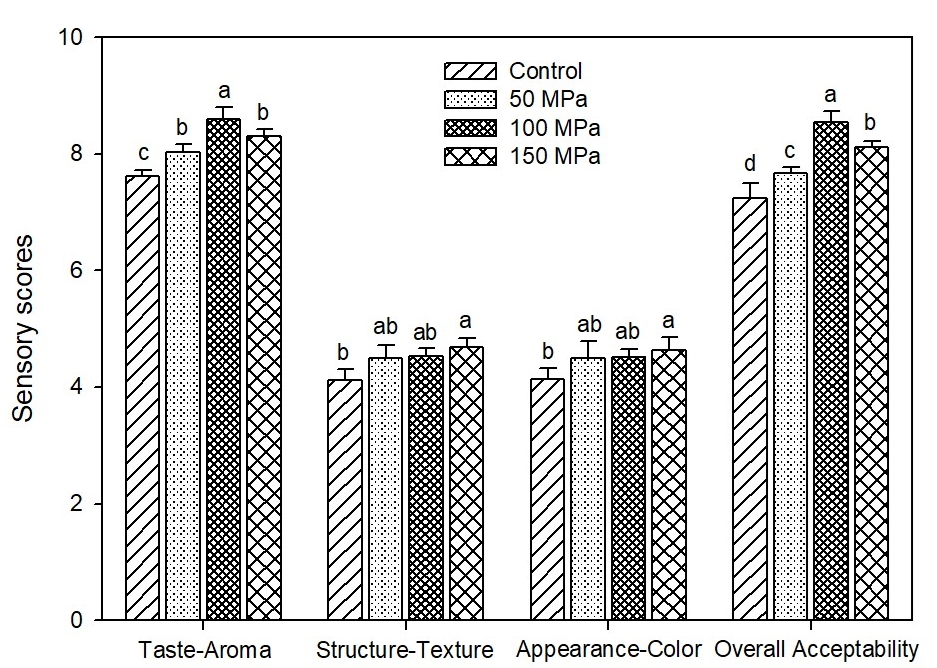
Figure 4. Sensory properties of the low-fat yoghurt samples made from unhomogenised (control) and homogenized milk at 50, 100 and 150 MPa pressure
High pressure homogenization process applied to milk led to a change in sensory ratings of yoghurt samples. However, this change was significant only for taste-aroma and overall acceptability (p<0.05). According to Figure 4, our results showed that high-pressure treatments up to 100 MPa were significantly effective for improving taste-aroma in yoghurt samples (p<0.05). However, it was negatively affected when pressure increased to 150 MPa, which is explained probably due to slightly sour taste as expressed by some panelists. The structure-texture scores of yoghurt samples increased depending on the homogenization process and homogenization pressure applied to milk (p<0.05). While a weak texture was detected in the control sample compared to yoghurt samples produced from high-pressure homogenized milk, a more creamy and colloidally uniform texture was observed in the samples obtained after milk homogenization. This improvement in the structure-texture of samples is consistent with the instrumental analysis results, this effect could be explained by strengthen the yoghurt gel structure resulting in the incorporation of homogenized fat globules into the gel network structure (Sodini et al., 2004). Similar results obtained by Ciron et al. (2011), who noted that improvements in textural properties of low-fat yoghurt achieves by microfluidization of milk, which is attributed by increasing interactions between milk proteins and fat globules because of changing in particle size and microstructure. The results in Figure 4 indicate that the mean scores for appearance-colour of low-fat yoghurts obtained after milk homogenization were significantly higher than control sample (p<0.05). As expected, reduction of fat globules by homogenization resulted whiter and smoother appearance of yoghurts made from homogenized milk, which is in line colour evaluation. As far as similar the assessed sensory attributes, overall acceptability increased by the homogenization process applied to milk. Amongst the low-fat yoghurts, the highest score was observed from samples after milk homogenized at 100 MPa, and the increasing pressure up to 150 MPa caused a significant decrease in overall acceptability (p<0.05), may be due to the low appreciation of the taste-aroma by panelists.
Principal component analysis
A principal component analysis was carried out to visualize the effect of high-pressure homogenization on the observations of low-fat yoghurt (Figure 5).
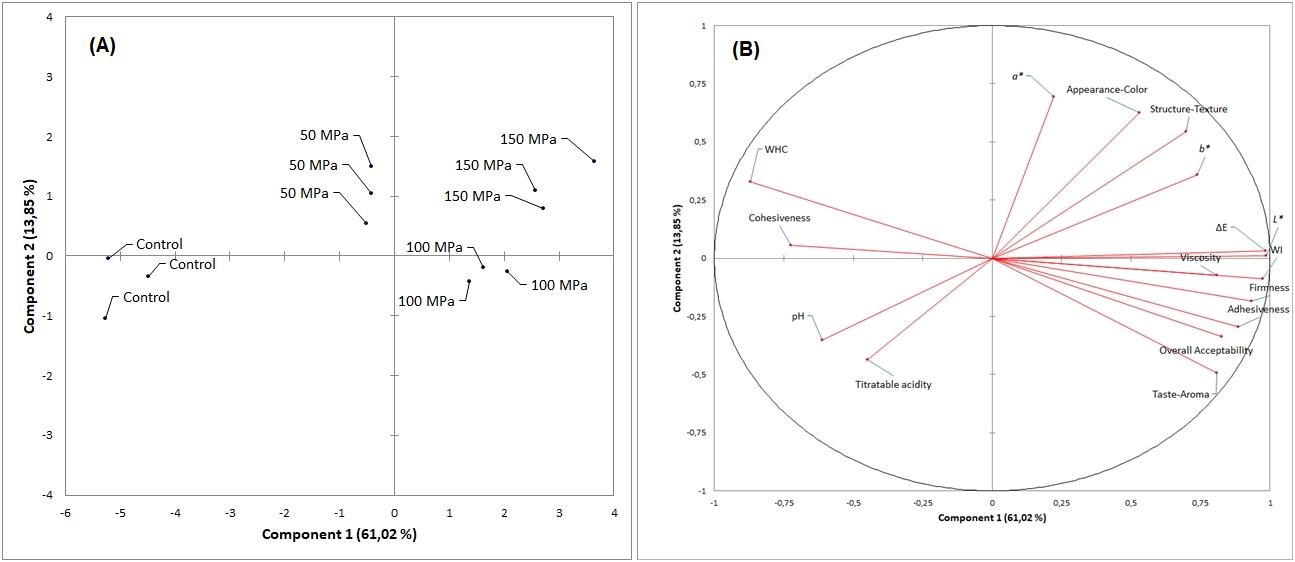
Figure 5. Principal component analysis. (A) Score plot and (B) loading plot of low-fat yoghurt samples made from unhomogenised (control) and homogenized milk at 50, 100 and 150 MPa pressure
Among the main components, the eigenvalues of the first two principal components (PC1 and PC2) were found to be greater than 1 (9.764 and 2.217, respectively) and these basic components with eigenvalues greater than 1 were taken into account in explaining the variation among the samples in terms of observations. PC1 and PC2 explained 61.02 % and 13.85 % of the variance, respectively, with 74.88 % of the total variation. As seen in score plot (Figure 5a), the control yoghurt and yoghurts from milk homogenized at 50 MPa are located on the negative side of PC1 and other samples are located on the positive side. The control sample was far separated from other yoghurt samples produced from homogenized milk, indicating it has distinct properties from the other groups. Similarly, homogenization at 50 MPa pressure was separated from 100 and 150 MPa pressure indicated that different pressures had various effects. The loading plot (Figure 5b) indicated that the attributes of WHC, L*, WI, ΔE, firmness, adhesiveness, viscosity, taste-aroma and general acceptability had a high loading (>0.8) in PC1. Concerning PC2, the attributes of a* and appearance-colour had a high loading (>0.6) (Table 2).
Table 2. Principal component factor loadings and their contributions from PCA of observations for low-fat yoghurt
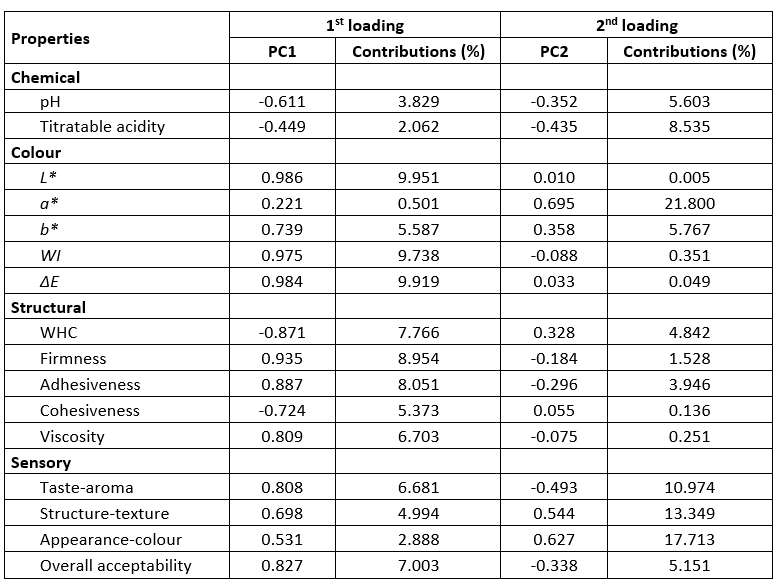
These results indicated that the most important variables for the PC1 were the colour and textural properties, which was affected by milk homogenization at 100 and 150 MPa. From the Figure 5b, appearance-colour was positively related to colour properties and structure-texture because of located on the same quadrant. On the other hand, general acceptability was closely related with viscosity and textural properties, suggesting these variables, which enhance significantly with the application of homogenization with the pressure higher that 50 MPa, are effective in sensory preference.
Conclusion
This study described the effect of high-pressure homogenization on the physicochemical, rheological, textural, microstructural and sensorial properties as well as acidification kinetics of low-fat yoghurt. The results demonstrated that the homogenization treatment after 50 MPa was altered the fermentation kinetics of starter culture and fermentation time decreased to 210 min by milk homogenization at 100 and 150 MPa. High-pressure homogenization led to increase the whiteness index and the colour difference among the samples was noticeable visually obvious. The processing of milk by high-pressure homogenization clearly contributed to increase the water holding capacity and textural properties as well as viscosity of low-fat yoghurts, suggesting that high pressure homogenization can significantly overcome the physical and structural problems of low-fat yoghurt. While sensory properties of yoghurt samples were enhanced by milk homogenization at high pressures, considering the general acceptability, yoghurt produced after milk homogenization at 100 MPa p was more acceptable. Consequently, high-pressure homogenization process at 100 MPa showed an important potential both to improving the yoghurt structure and reduce the fermentation time and thus costs at industrial levels for low fat yoghurt.
Acknowledgements
This research was supported by the Fund of Scientific Research Projects of Trakya University (TUBAP, Grant No: 2017/77).
Poboljšanje kinetike zakiseljavanja, strukturnih i senzorskih svojstava nemasnog jogurta primjenom visokotlačne homogenizacije
Sažetak
U ovom istraživanju ispitivan je utjecaj homogenizacije primjenom visokog tlaka pri 50, 100 i 150 MPa, na fizikalno-kemijska, reološka, mikrostrukturna i senzorska svojstva, kao i na teksturu te kinetiku zakiseljavanja nemasnog jogurta. Prema dobivenim rezultatima, fermentacija uzoraka jogurta proizvedenih iz mlijeka homogeniziranog pod visokim tlakom trajala je kraće u usporedbi s kontrolnim uzorkom. Homogenizacija mlijeka pri visokim tlakovima rezultirala je povećanjem kapaciteta zadržavanja vode s 42,83 na 52,67 % i čvrstoće koaguluma s 1,01 na 1,45 N. Homogenizacijom mlijeka povećala se i viskoznost (Ƞ50) uzoraka jogurta do 0,446 Pa.s, a svi uzorci jogurta su okarakterizirani kao pseudplastične ne-Newtnovske tekućine. Analiza mikrostruktura pokazala je da kontrolni uzorak ima tipičnu otvorenu poroznu gel-mrežu kazeina, dok su manje porozne i gušće proteinske mreže utvrđene u jogurtima od homogeniziranog mlijeka. Utvrđen je pozitivan učinak postupka homogenizacije mlijeka na senzorska svojstva jogurta. Analizom glavnih komponenti grafički je prikazan učinak tretmana homogenizacijom te je potvrđena pozitivna korelacija između strukturnih svojstava i ukupne prihvatljivosti jogurta. Među ispitivanim nemasnim jogurtima, homogenizacija mlijeka pri tlaku od 150 MPa imala je najveći potencijal za poboljšanje strukture jogurta, dok je tlak od 100 MPa rezultirao najprihvatljivijim senzorskim svojstvima.
Ključneriječi: nemasni jogurt; homogenizacija pod visokim pritiskom; kinetika fermentacije; reologija; mikrostruktura; senzorna svojstva