Introduction
Originating from the Middle East and the Balkans, yoghurt is one of the fermented dairy products made with starter cultures and is often preferred by consumers (Fisberg and Machado, 2015). In order to increase consumers' interest in healthy nutrition and to develop the functional food market, various types of yoghurt (plain, probiotic and fruity, etc.) are produced nowadays (Gahruie et al., 2015; Feng et al., 2019). In this context, yoghurt is a unique source of protein, minerals and vitamins to consumers (Gahruie et al., 2015). In addition to valuable nutritional properties, dairy products are poor sources of phenolic compounds, although they are highly demanded by consumers in nutrition. Therefore, plant-based supplements are used to improve the phenolic compound content of yoghurt and increase its antioxidant capacity. At the same time, yoghurt supplemented by fruit extracts or puree, appeals to consumers of all age groups (Turgut and Cakmakci, 2018). On the other hand, acetaldehyde, the characteristic aroma compound of yoghurt, can be masked by the addition of fruit (Terzioğlu and Bakirci, 2023). It is also reported that the addition of fruit sugar to yoghurt contributes to the formation of HMF, which is one of the advanced products of the Maillard reaction and proved to be a genotoxic compound (Terzioğlu et al., 2022). Being a rich source of potassium and dietary fibre, banana plays an important role in maintaining cardiovascular health (Falcomer et al., 2019). Kiwi, on the other hand, positively affects the immune system, digestive system and protection against oxidative stress with due to high content of minerals, vitamins, fibre, antioxidant substances and phenolic compounds (Terzioğlu and Bakirci, 2023). Kiwifruit has the content that can meet the daily need for vitamin C, but it is also very rich in actinidin (about 50% of soluble proteins), a cysteine protease. It has been reported that the enzyme actinidin can be used as a milk coagulant of plant origin in dairy products coagulated by acidic proteases (Zhang et al., 2017; Richardson et al., 2018). On the other hand, actinidin, an active protease, breaks down casein into bitter peptides (Katsaros et al., 2009; Kaur et al., 2022). However, it has also been found that kiwifruit is a source of various pigments such as carotenoids, chlorophyll and anthocyanins (Sun-Waterhouse et al., 2013). In line with the information provided, the present study aimed to exploit the antioxidant and pigmenting properties of kiwi on the one hand, and on the other hand, bananas, another fruit that appeals to a wide group of consumers, were used in combination with kiwi to suppress the formation of a bitter taste. All these aspects have been taken into consideration while planning this research and it was aimed to use different combinations of kiwifruit and banana puree to produce experimental yoghurt samples. For this purpose, volatile compounds, antioxidant activity, angiotensin converting enzyme (ACE) inhibitory activity, 5-hydroxymethylfurfural (HMF) content, reducing sugar content, microstructure and some physicochemical properties were investigated.
Materials and methods
Materials
Raw cow milk was obtained from Atatürk University Food and Livestock Application and Research Center (Erzurum, Türkiye). Starch and sugar were purchased from local shops in Erzurum, skim milk powder (SMP) was supplied from İzi Milk Company and starter culture was obtained from Peyma-Chr Hansen (İstanbul, Türkiye) were used in this study.
Preparation of the kiwi and banana purees
After peeling the ripe kiwi and banana fruits in the production of puree, they were homogenized with a culinary blender. Then, after adding sugar and starch, the fruit purees were taken into jars and pasteurized at 90±1 °C for 10 minutes.
Production of experimental yoghurt samples
The production flow chart of the experimental yoghurt samples with banana-kiwi addition used in this study is presented in Figure 1. In the production of stirred type yoghurt, yoghurts were cooled to 24-25 °C after incubation. Then, fruit puree was added to the yoghurts and yoghurt samples were stored at 4 °C.
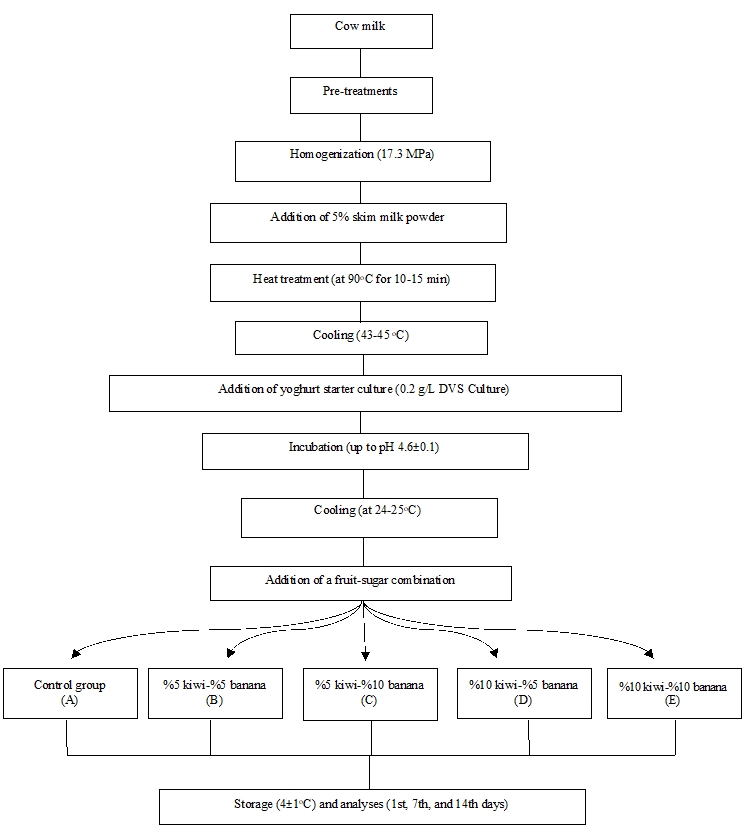
Figure 1. The production flow chart of the experimental yoghurt samples
Physicochemical analysis
Physicochemical analysis of raw milk, skim milk powder (SMP), and experimental yoghurt samples have been carried out according to the methods of Kurt et al. (2015).
Antioxidant activity
The DPPH method was used to determine the free radical scavenging activity of yoghurt samples. For this purpose, 50 μL yoghurt sample and 5 mL of 0.004 % DPPH methanolic solution were put in the 10 mL test tube. After waiting for 30 minutes, the absorbance (517 nm) was measured in a spectrophotometer. Results of antioxidant activity were calculated as mg TE/100 g using the curve obtained using Trolox (Arslaner et al., 2021). In the CUPRAC analysis, the method given by Apak et al. (2004) was taken as a reference. Accordingly, 1 mL of copper (II) chloride solution, 1 mL neocuproine solution, 1 mL of ammonium acetate buffer solution (pH=7.0) and 1 mL of pure water were added to the 100 μL sample and mixed. Then, after waiting for 1 hour, the absorbance (450 nm) was measured in a spectrophotometer (Optizen POP). Trolox was used as the standard in the analysis.
Total phenolic compounds
The method given by Arslaner et al. (2021) served as a basis for the determination of total phenolic compounds of yoghurt samples. For this purpose, yoghurt sample (0.1 mL), Folin-Ciocalteu reagent (1 mL), and distilled water (46 mL) were added into a volumetric flask and shaken for 3 minutes. Then, 3 mL of Na 2CO 3 (2 %) solution was added and the contents of the volumetric flask were shaken at regular intervals and left for 2 hours. Subsequently the absorbance was measured at a wavelength of 760 nm. All of the applied processes were also performed for standard gallic acid solutions.
Volatile compounds
Determination of volatile compounds of the yoghurt samples was carried out by modifying the method exhibited by Kavaz (2012). Yoghurt samples belonging to the 1st, 7th, and 14th days of the storage were weighed as 5 g each into a 20 mL glass headspace vial. Yoghurt samples were incubated at 80 °C with shaking for 30 minutes prior to injection to obtain readings in the GC-MS (GC-MS-QP2010, Shimadzu). Headspace injection mode and DB-WAX Ultra Inert (Agilent, 30 m, 0.25 mm, 0.25 μm) column were used to run volatile compounds in GC-MS. The column oven temperature of gas chromatography was initially at 40 °C for 2 minutes, then gradually increased to 200 °C at a rate of 5 °C/min. Helium was used as a carrier gas with a flow rate of 1.70 ml/min in the system.
ACE inhibitory activity
50 µL of yoghurt sample, 180 µL of HHL solution and 20 µL of ACE solution were mixed and incubated at 37 °C for 90 minutes. Afterwards, 1 M HCl (250 µL) was added and incubation was completed. Subsequently, ethyl acetate (1.7 mL) was added to the mixture and extraction was carried out. It was evaporated at 100 ºC for 15 minutes and after adding distilled water (1 mL) to the hippuric acid solution, absorbance (228 nm) was measured in a UV-spectrophotometer (Optizen POP) (Cushman and Cheung, 1971; Nakamura et al., 1995).
HMF content
The method given by Sert et al. (2016) was taken as a reference in determining the HMF content of the yoghurt samples. For this purpose, 5 mL oxalic acid was added to 5 mL of yoghurt and mixed. The mixture is soaked in a water bath for 1 hour, then cooled to 24-25 ºC. Then the mixture was filtered and 4 mL supernatant was taken and 1 mL 0.05 M thiobarbituric was added to it. After the mixture was kept in the water bath again for a while, the absorbance (443 nm) measurement was performed in the spectrophotometer (Optizen POP).
Reducing sugar analysis
Reducing sugar analysis was performed according to the dinitrosalicylic acid solution (DNS) method given by Teixeira et al. (2012). 5 mL Carrez I and 5 mL Carrez II solution were added to the yoghurt sample and filtered. Then the filtrate was completed to 100 mL with activated charcoal to be kept in the dark for 1 hour. The mixture was re-filtered and heated with a solution of 2 mL of filtrate, 6 mL of DNS for 5 min. After the mixture was cooled, the absorbance (540 nm) was measured in a spectrophotometer (Optizen POP).
Microstructure
Microstructure analysis of the experimental yoghurt samples was conducted using scanning electron microscopy (SEM; Quanta-Feg 250 FEI) according to the method exhibited by Akalın et al. (2012). In the microstructure analysis, a magnification degree of 1000x was used for the images.
Statistical analysis
Statistical analysis was performed according to a Randomized Complete Block Design factorial experiment. The obtained data were analysed by variance and correlation analyses on SPSS 20 Package software (SPSS Inc., Chicago, IL). The statistically significant groups were determined by Duncan’s Multiple Range Test. In addition, SIMCA-P + 14.1 (UMETRICS, Umea, Sweden) was used when performing Principal Component Analysis (PCA).
Results and discussion
Physicochemical analysis
The physicochemical properties of SMP, cow milk and the experimental yoghurt samples are presented in Table 1. The general composition of cow milk and SMP were suitable with the sources in the literature (Kamber and Harmankaya, 2019; Brodziak et al., 2021).
Table 1. Physicochemical analysis of the cow milk, SMP and experimental yoghurts
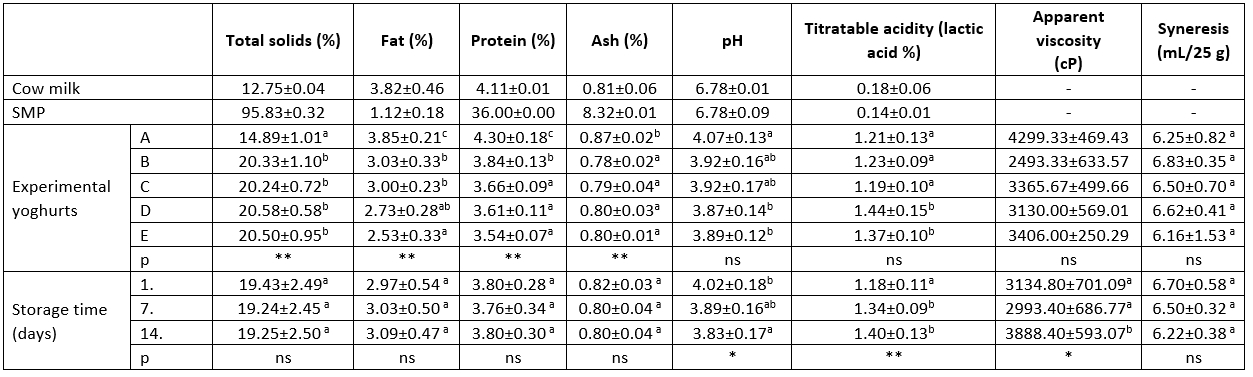
a-c: Different letters indicate significant differences in column
*: p<0.05, **: p<0.01, ns: p>0.05
SMP: Skim milk powder; sample A: control group, sample B: 5 % kiwi puree+5 % banana puree, sample C: 5 % kiwi puree+10 % banana puree, sample D: 10 % kiwi puree+5 % banana puree, sample E: 10 % kiwi puree+10 % banana puree.
The addition of kiwifruit and banana fruit to the experimental yoghurt samples had a statistically very significant effect (p<0.01) on total solids, fat, protein and ash content (Table 1), it was also determined that it had an insignificant effect (p>0.05) on the other physicochemical parameters. Experimental yoghurt samples with t added fruit showed an increase in total solids as expected, while a decrease was observed in fat and protein content. The storage period had a statistically very significant effect (p<0.01) on titratable acidity, as well as a statistically significant effect (p<0.05) on pH and apparent viscosity. During the storage period, a decrease in pH value and an increase in titratable acidity could be observed. Blassy et al. (2020) reported that in different fruit supplemented yoghurt samples the total solids content ranged from 15.11 % to 17.58 %, the pH value ranged from 4.13 to 4.70 and the apparent viscosity value ranged from 7024.52 mPas to 12.795,55 mPas.
Colour
The colour values ( L*, a* and b*) of experimental yoghurt samples are presented in Table 2. The addition of banana-kiwi had a statistically very significant effect (p<0.01) on L*, a*, and b* values while the storage period had a statistically significant effect (p<0.05) only on the a* value. The addition of banana-kiwi to yoghurt samples caused a decrease in the L* value, which is an indicator of darkness-brightness, compared to the control group. On the other hand, the increase at the ratio of banana in the fruit yoghurt samples decreased the L* value more than kiwi. The decrease in the L* value might be explained by the increasing in darkening of the colour due to of the absorption of the free water in the yoghurt by the fibre content of the fruit (García-Pérez et al., 2005).
The addition of different proportions of banana-kiwi caused a reduction in the a* value representing greener tones compared to the control group. The decrease in the a* value increased due to the increase in the kiwi fruit ratio, as expected. The addition of banana-kiwi at different proportions to the yoghurt samples caused an increase in the b* value which expresses yellowness. As expected, the b* value increased in parallel with the increase at the proportion of bananas. It has been reported that the deterioration of the stabilization of casein micelles due to the influence of temperature during the pasteurization process in addition to fruit addition caused the increase in the a* and b* values (Costa et al., 2015). Baria et al. (2020) reported that the L* value ranged from 63.54 to 77.51, the a* value from 4.04 to 10.33 and the b* value from -2.69 to 2.09 in the yoghurt samples they enriched with black carrots.
Table 2. L*, a* and b* values of the experimental yoghurts and their statistical evaluations
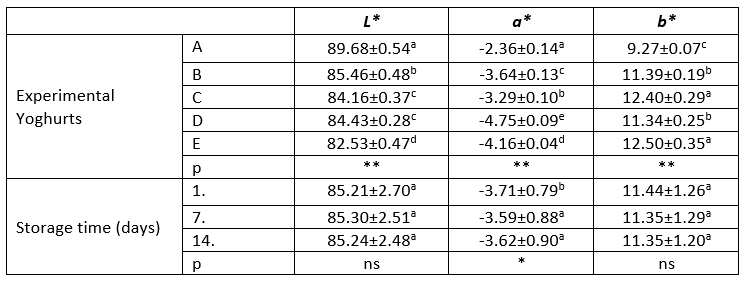
a-c: Different letters indicate significant differences in column
*: p<0.05, **: p<0.01, ns: p>0.05
Sample A: control group, sample B: 5 % kiwi puree+5 % banana puree, sample C: 5 % kiwi puree+10 % banana puree, sample D: 10 % kiwi puree+5 % banana puree, sample E: 10 % kiwi puree+10 % banana puree.
Volatile compounds
Volatile compounds play an important role in the formation of aroma in yoghurt. Among the volatile compounds, acetaldehyde, diacetyl, and acetoin are among the main components in the characteristic aroma of yoghurt (Cheng, 2010). These compounds are synthesized by classical yoghurt cultures ( Streptococcus thermophilus and Lactobacillus delbrueckii subsp. bulgaricus) during fermentation. Lactose, methionine and threonine are the precursor substances for the synthesis of acetaldehyde by yoghurt bacteria, while pyruvate, which is produced by lactose metabolism and citrate conversion, is used as the precursor substance for the synthesis of diacetyl and acetoin
These compounds formed in yoghurt vary according to the type of culture, type of milk, yoghurt production, and added supplements (Zhao et al., 2018). The acetaldehyde, acetoin, and diacetyl analyses results of the experimental yoghurt samples are presented in Table 3. Accordingly, the addition of banana-kiwi caused a statistically very significant effect (p<0.01) decrease in the content of acetaldehyde, acetoin, and diacetyl in the experimental yoghurt samples compared to the control group. The lowest content of acetaldehyde and diacetyl was determined in sample E with the highest amount of banana-kiwi added. In addition, considering the total fruit ratios of the C and D samples, the increase of kiwi component was more effective with respect to the content of the aforementioned volatile compounds compared to bananas (Table 3). It is believed that the decrease in protein, one of the total solid components, is effective in reducing the amounts of volatile compounds due to the addition of fruit. Indeed, similar results have already been reported by some authors (Sahingil and Hayaloglu, 2022; Terzioğlu and Bakirci, 2023).
Table 3. Volatile compounds of the experimental yoghurts
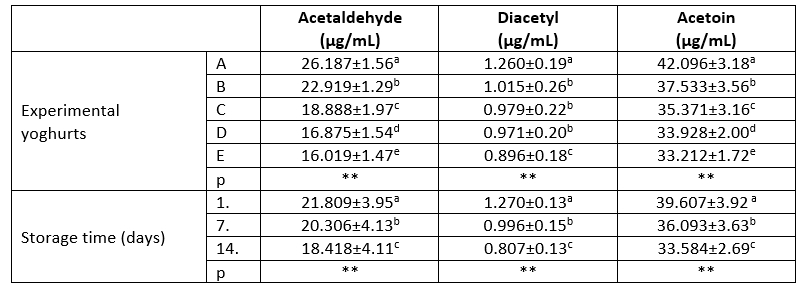
a-e: Different letters indicate significant differences in column
**: p<0.01
Sample A: control group, sample B: 5 % kiwi puree+5 % banana puree, sample C: 5 % kiwi puree+10 % banana puree, sample D: 10 % kiwi puree+5 % banana puree, sample E: 10 % kiwi puree+10 % banana puree
Antioxidant activity
Oxidation, which is a chain reaction, can be defined as the conversion of unsaturated fatty acids into various compounds such as aldehydes, ketones, and hydroxyl acids by decomposition in the presence of oxygen. There are various factors affecting oxidation, and since it is not possible to remove all of these factors from the environment, the use of antioxidant substances has become widespread. Various side effects of synthetic antioxidants have brought up the search for natural antioxidants. In this context, various foods can be used as an alternative to synthetic antioxidants due to their antioxidant compounds contain. Kiwi and banana contribute to the taste, aroma, and consistency of products such as yoghurt with their rich antioxidant content, while at the same time they come to the fore in preventing oxidation with their antioxidant compounds (Ekiz and Oz, 2019; El Azab and Mostafa, 2022). DPPH and CUPRAC analyses are among the most common methods used to determine antioxidant activity. Antioxidant activity (DPPH and CUPRAC) analysis results of the experimental yoghurt samples are presented in Table 4. It was observed that the addition of banana-kiwi and the storage period very significantly affected (p<0.01) the DPPH and CUPRAC values in the experimental yoghurt samples. DPPH values were significantly increased in yoghurt samples with different ratios of banana-kiwi added (20.43-40.33 mg TE/100 g) compared to control group (6.02 mg TE/100 g). In addition, CUPRAC values were significantly increased in yoghurt samples with different ratios of banana-kiwi added (13.58-33.86 mg TE/100 g) compared to control group (4.37 mg TE/100 g). It was determined that the addition of kiwi contributed more to the increase in antioxidant activity compared to banana, as expected. The amounts of DPPH and CUPRAC decreased with the storage period. Arslaner et al. (2021) determined that the DPPH values ranged from 21.54 mg TE/100 g to 26.73 mg TE/100 g and the CUPRAC values ranged from 12.50 mg TE/100 g to 15.03 mg TE/100 g in the yoghurt samples to which they added Hibiscus sabdariffa L. flower marmalade.
Total phenolic compounds
Phenolic compounds such as flavonoids, phenolic acids, lignans, and stilbenes, which are naturally found in fruits and vegetables in special, are secondary metabolism products. Phenolic compounds play an important role especially in preventing the occurrence of cancer, cardiovascular diseases, Alzheimer's disease, and intestinal disorders (Amerinasab et al., 2015). The addition of fruit to dairy products also contributes to the enrichment of deficient phenolic compounds. The phenolic compounds contained in kiwi and bananas transform yoghurt, which already has superior properties, into an entirely complete product (Zhu et al., 2021). TPC analysis results of the experimental yoghurt samples are presented in Table 4. It could be observed that the addition of banana-kiwi and the storage period had a statistically very significant effect (p<0.01) on TPC in the experimental yoghurt samples. The amount of TPC significantly increased in the yoghurt samples with different proportions of banana-kiwi added (17.08-37.52 mg GAE/100 g) compared to control group (5.19 mg GAE/100 g). It is believed that the reason for the high amount of TPC in the yoghurt samples with a higher kiwi content is due to the fact that kiwi contains phenolic compounds (7.7 mg/kg FW) at a higher rate compared to banana (3.0 mg/kg FW) (Park et al., 2015). During the storage period, the amount of TPC decreased. Terzioğlu et al. (2022) found that the amount of TPC in the yoghurt samples supplemented with pineapple puree ranged from 15.55 mg GAE/100 g to 30.53 mg GAE/100 g and that the amount of TPC increased with the addition of fruit.
Table 4. DPPH values, CUPRAC values, TPC amounts, ACE inhibitory activity, HMF content and reducing sugar content of the experimental yoghurts and their statistical evaluations
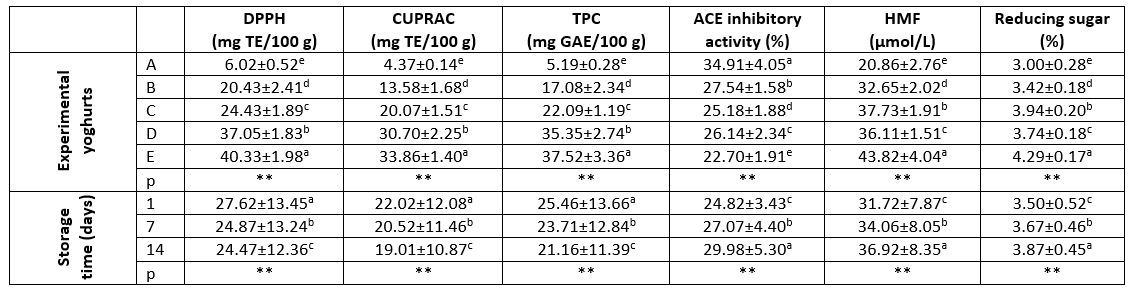
a-e: Different letters indicate significant differences in column
**: p<0.01
Sample A: control group, sample B: 5 % kiwi puree+5 % banana puree, sample C: 5 % kiwi puree+10 % banana puree, sample D: 10 % kiwi puree+5 % banana puree, sample E: 10 % kiwi puree+10 % banana puree
ACE inhibitory activity
Yoghurt is one of the important sources for the intake of bioactive compounds into the body. During fermentation in yoghurt, bioactive peptides with an inhibitory effect ACE are formed by the proteolytic activity of the starter cultures (Shori et al. 2020). On the other hand, many factors such as differences in the amino acid profile of milk, the use of different analytical methods, the use of different strains, the degree of proteolysis, the fermentation conditions and the storage conditions were found to be responsible for the differences in ACE inhibitor activity in yoghurt samples (Moschopoulou et al., 2018).
Results of the ACE inhibitory activity inexperimental yoghurt samples are given in Table 4. The addition of banana-kiwi and the storage period had a statistically very significant effect (p<0.01) on ACE inhibitory activity in the experimental yoghurt samples, with the highest ACE inhibitory activity observed in A sample (34.91 %), and the lowest in E sample (22.70 %), respectively. The addition of banana-kiwi at different ratio to yoghurt samples caused a decrease in ACE inhibitory activity. An increase in ACE inhibitor activity was observed during the storage period, depending on the proteolytic activity. Erkaya-Kotan (2020) reported that the ACE inhibitor activity of yoghurt samples supplemented with orange fibre varied between 32.52-58.50 % and increased during the storage period.
HMF and reducing sugar contents
HMF is the product of thermal degradation of sugars and is occurred in the advanced stages of the Maillard reaction, which is the reaction that occurs between the reducing sugars and their amino groups. It has been proven that HMF, which belongs to the group of furfurals, has genotoxic, cytotoxic and tumoral effects. HMF, which is one of the most important quality criteria for milk and milk products, is produced during heat treatment or storage in milk and milk products manufacture. The presence of a certain amount of HMF, which is toxic in milk and dairy products with a wide range of consumers, poses a risk to human health (Van den Oever and Mayer, 2021; Li et al., 2022). The HMF and reducing sugar contents of the experimental yoghurt samples are presented in Table 4. In the experimental yoghurt samples, banana-kiwi addition and storage period showed a statistically very significant (p<0.01) effect on the HMF contents of the yoghurt samples as follows – control sample A (20.86 μmol/L) < sample B (32.65 μmol/L) < sample D (36.11 μmol/L) < sample C (37.73 μmol/L) < sample E (43.82 μmol/L), respectively. Similar to the HMF content, the reducing sugar contents of the yoghurt samples followed the same pathway with control sample A (3.00 %) < sample B (3.42 %) < sample D (3.74 %) < sample C (3.94 %) < sample E (4.29 %), respectively. A large part of the sugar found in yoghurts with fruit supplements consists of glucose, lactose and fructose, and these sugars are called reducing sugars (Özdemir, 2021). In the experimental yoghurt samples, the addition of banana-kiwi caused an increase in the HMF content compared to the control group. However, when fruit was added, bananas were found to have a higher impact on the HMF content compared to kiwis, which is most probably related to the higher sugar content of bananas (Mohapatra et al., 2010; Satpal et al., 2021). It coul also be observed that the HMF content increased with the progress of storage in the experimental yoghurt samples (Table 4). Such results are in correspondence to previous findings of Li et al. (2022) who emphasized the effect of the fermentation process and the added components on the HMF content in milk and dairy products. On the other hand, the number of studies investigating HMF content in yoghurt samples is quite limited in the literature. Cui et al. (2020) detected HMF content up to 3.43 mg/kg in various yoghurt samples, Terzioğlu et al. (2022) reported that HMF content increased with fruit addition in yoghurt samples with pineapple addition and HMF content in yoghurt samples ranged from 17.16 to 39.04 μmol/L. The maximum detectable amount of HMF, a genotoxic and cytotoxic compound, in milk and dairy products has been reported by the European Food Safety Authority (EFSA) as 15 mg/kg (Hou et al., 2022). In this context, HMF content in the experimental yoghurt samples produced in the current research was below the maximum limit.
Microstructure
Microstructure is defined as the three-dimensional gel network arrangement of protein, fat globules, and other components in yoghurts, and is an important quality parameter related to the processing conditions, functional properties and the bioavailability of the components in the product (Nguyen et al., 2018). The microstructure of yoghurt formed during fermentation varies depending on factors such as heat treatment, milk type, storage period, protein concentration, shape, and size of protein particles (Akalın et al., 2012). Figure 2 consists of images of the microstructures of the yoghurt samples. There are significant differences between the microstructures of the control and the banana-kiwi added yoghurt samples. According to the obtained results, a more homogeneous structure was observed in the control group samples compared to the fruit-added yoghurt samples and it was determined that the serum pore diameters were smaller. as Also, as the ratio of banana and kiwi added to the fruit yoghurts increased, deeper pores were formed on the surface and the number of pores increased. At the same time, the addition of kiwi caused a more intense increase in the pore diameter compared to banana.

Sample A: control group, sample B: 5 % kiwi puree + 5 % banana puree, sample C: 5 % kiwi puree + 10 % banana puree, sample D: 10 % kiwi puree + 5 % banana puree, sample E: 10 % kiwi puree + 10 % banana puree
Figure 2. SEM micrographs of the experimental yoghurts
Correlation analysis and PCA
The correlation analysis of various characteristics of banana-kiwi supplementary yoghurt samples with the control group is presented in Figure 3.
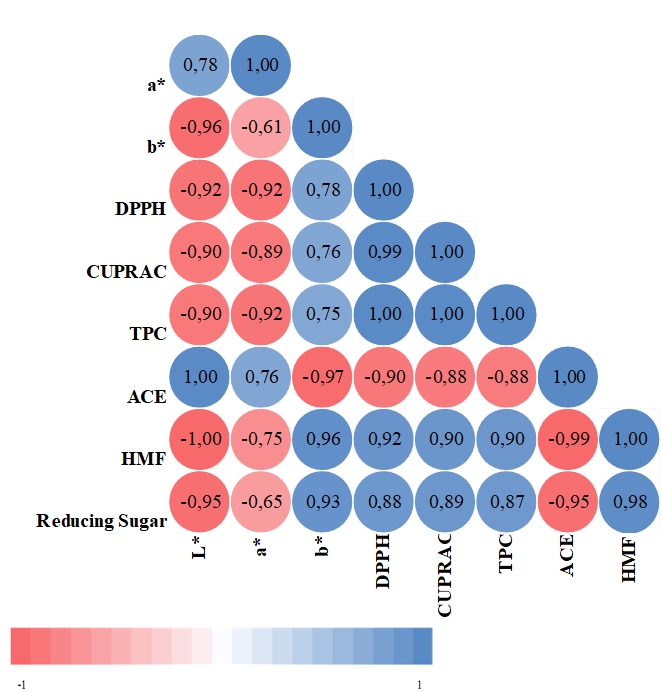
Figure 3. Correlation analysis
While a positive correlation was observed between L* value and ACE inhibitor activity (r=1.00, p<0.01), a negative correlation was found between the L* value and the b* value (r=-0.96, p<0.01), DPPH (r=-0.92, p<0.05), CUPRAC (r=-0.90, p<0.05), TPC (r=-0.90, p<0.05), HMF (r=-1.00, p<0.01) and reducing sugar (r=-0.95, p<0.05). A negative correlation was found between the a* value and the DPPH (r=-0.92, p<0.05), CUPRAC (r=0.89, p<0.05) and TPC (r=-0.92, p<0.05). There was a negative correlation between the b* value and ACE inhibitor activity (r=-0.97, p<0.01), and a positive correlation between the b* value and HMF (r=0.96, p<0.01) and reducing sugar (r=0.93, p<0.05). While the negative correlation between DPPH and ACE (r=-0.90, p<0.05) was determined, positive correlation was found between DPPH and CUPRAC (r=0.99, p<0.01), TPC (r=1.00, p<0.01), HMF (r=0.92, p<0.05) and reducing sugar (r=0.88, p<0.05). A positive correlation was found between CUPRAC and TPC (r=1.00 p<0.01), HMF (r=0.90, p<0.05) and reducing sugar (r=0.89, p<0.05). There was also a positive correlation between TPC and HMF (r=0.90, p<0.05). A negative correlation was determined between ACE inhibitor activity and HMF (r=-0.99, p<0.01) and reducing sugar (r=-0.95, p<0.05). A positive correlation was found between HMF and reducing sugar (r=0.98, p<0.01).
In the examination of various properties in experimental yoghurt samples, PCA was performed to better observe the differences between the samples. In this respect, score scatter plot (Figure 4A), dendrogram (Figure 4B), loading scatter plot (Figure 4C) and biplot (Figure 4D) graphs of the experimental yoghurt samples are presented.
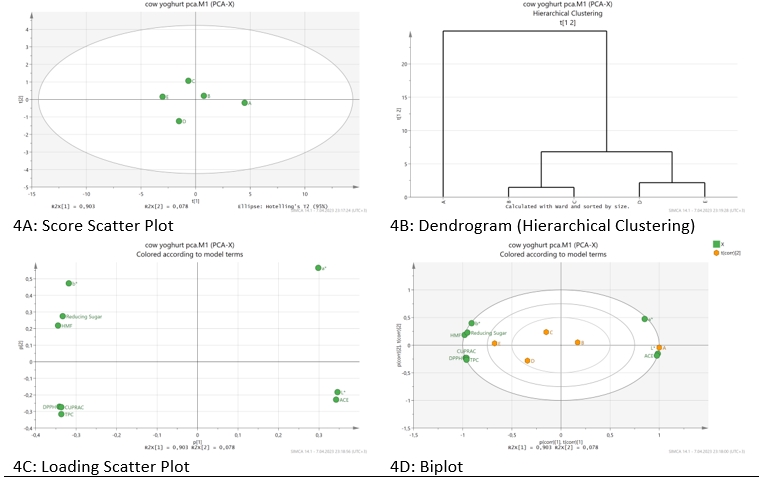
Figure 4. Principal component analysis
The first two basic components (PC1=90.3 % and PC2=7.8 %) explained 98.1 % of the variance. As can be seen from Figure 4A and Figure 4B, the experimental yoghurt samples were divided into 3 groups. There are sample A in group I, samples B and C in group II, and samples D and E in group III. As can be seen from Figure 4C, the upper right part of the parcel has an a* value, and the lower right part has an L* value and ACE inhibitor activity. However, it was determined that sample A had higher values in terms of L* value and ACE inhibitory activity compared to other samples (Figure 4D). On the other hand, in the upper left part of the parcel, the b* value, reducing sugar and HMF, and in the upper left part, DPPH, CUPRAC and TPC are clustered. Compared to other samples, sample E exhibited superior properties in terms of b* value, reducing sugar, HMF, DPPH, CUPRAC and TPC parameters (Figure 4D). HMF has been reported to be associated with the decomposition reaction of reducing sugar (Agrib et al., 2017), and the current research has also found parallels between the contents of HMF and reducing sugar. Zor et al. (2022) reported that phenolic compounds were directly related to antioxidant activity in both correlation analysis and PCA.
Conclusion
Considering all of the obtained results, using kiwi and banana fruits together and in different proportions improved physicochemical, biochemical and microstructure properties of experimental yoghurts. Indeed, when fruit yoghurts and control group yoghurts were compared in terms of antioxidant activity and phenolic compounds, it could be observed that DPPH values increased approximately 7 times and CUPRAC values and phenolic compounds approximately 8 times after the addition of fruit. On the other hand, the content of volatile compounds that provide the characteristic aroma of the yoghurt and ACE inhibitory activity decreased by the addition of fruit. However, it was found that adding banana-kiwi to experimental yoghurts in different ratios and extending the storage time increased the content of HMF and reducing sugars. The addition of fruit to the yoghurt samples resulted in a more porous microstructure characterised by deeper pores. On the other hand, more comprehensive studies are needed in order to recommend fruit yoghurts to consumers so that they can consume fruit yoghurt samples with higher nutritional value and more functional qualities.
Hlapljivi spojevi, antioksidacijska aktivnost, ACE inhibitorna aktivnost, udio HMF i mikrostruktura voćnih jogurta
Sažetak
U ovom radu su proizvedeni tekući voćni jogurti s dodatkom dva različita voćna pirea (kivi i banana). Svim uzorcima su određivana fizikalno-kemijska svojstva, udio hlapljivih spojeva, antioksidacijska aktivnost primjenom 2,2-difenilpilhidrazil (DPPH) i CUPRAC metoda, udio ukupnih fenola (TPC), sposobnost inhibicije angiotensin konvertirajućeg enzima (ACE), udio 5-hidroksimetilfurfurala (HMF), udio reducirajućih šećera i mikrostruktura. Dodatak voćnog pirea značajno je (p<0.01) utjecao na udjele ukupne suhe tvari, masti, proteina, pepela, parametre boje (vrijednosti L*, a*, b*), udio hlapljivih spojeva, vrijednosti antioksidacijske aktivnosti određene metodama DPPH i CUPRAC, udio TPC, stupanj ACE inhibicijske aktivnosti, udio HMF-a i reducirajućeg šećera, kao i na i ukupnu senzorsku prihvatljivost jogurta, dok nije utvrđen značajan (p>0.05) učinak na pH vrijednost, titracijsku kiselost, prividnu viskoznost i sinerezu. Trajanje skladištenja je vrlo značajno (p<0.01) utjecalo na titracijsku kiselost, vrijednosti antioksidacijske aktivnosti određene metodama DPPH i CUPRAC, udio TPC, stupanj ACE inhibicijske aktivnosti, udio HMF-a, smanjenje udjela reducirajućih šećera i ukupnu prihvatljivost, dok je značajno (p<0.05) utjecalo na pH vrijednost, prividnu viskoznost i a*. Iako je dodavanje banane i kivija relativno potisnulo karakteristični okus eksperimentalnih uzoraka jogurta, poboljšao je vrijednosti antioksidacijske aktivnosti određene metodama DPPH i CUPRAC, udio TPC. S druge strane, otkriveno je da dodavanje banane-kivija povećava sadržaj HMF, toksičnog spoja. Jogurt se može preporučiti kao važan izvor bioaktivnih spojeva s ACE inhibitornom aktivnošću. Prema rezultatima mikrostrukturne analize, u uzorcima kontrolne skupine utvrđena je homogenija struktura u usporedbi s uzorcima jogurta s dodanim voćem i utvrđeno je da su promjeri pora u serumu manji.
Ključne riječi: hlapljivi spojevi; antioksidacijska aktivnost; ACE inhibitorna aktivnost; sadržaj HMF; mikrostruktura