Introduction
Genus Juniperus L. is the second most diverse genus of coniferous plants, as well as the most numerous genus of the Cupressaceae family, with 53 species distributed across broad regions of Northern Hemisphere (Farjon 2010). The initial diversification, which occurred during the Eocene in Europe (Allen and Armstrong 2008), continues today in three separate, though climatologically similar, arid areas of the Mediterranean, northern Mexico and southwestern USA, as well as in central Asia (Mazur 2021). The genus is characterized by high demands for light and pioneering character, often enabling juniper species to thrive in a climate where few woody plants survive (Sanchez-Salguero and Camarero 2020). Seeds of this wind-pollinated genus are dispersed by frugivores, mostly birds and small mammals (Santos et al. 1999). Taxonomically complex (Mazur 2021), the genus is easily recognized by fleshy cones with merged seed scales, as well as the diversity of leaf forms, with species having either needles or scaly leaves, or even both, depending on the age of the plant (Adams 2014a). In addition, the majority of junipers are characterized by sexual dimorphism, i.e., the presence of exclusively male or female cones on an individual plant (Nuñez et al. 2015).
Dioecy, the characteristic of a species that has distinct male and female individuals, even though relatively rare among plants, is present in 65% of contemporary gymnosperm species, and is a dominant sexual system in eight families (Walas et al. 2018). Individuals of dioecious plant species often differ in a range of ecological, morphological, and physiological traits (Hultine et al. 2016). This is most commonly expressed through the larger floral displays of male individuals, as well as through larger or more abundant flowers, or cones when gymnosperms are considered. Additionally, secondary sexual traits unrelated to sexual organs can also be used to differentiate the sexes, including shoot structuring, leaf morphology and physiology, water use efficiency and susceptibility to pests and herbivores (Moore and Pannell 2011). These changes in secondary traits reflect sex-specific adaptations to different resource needs, related to the timing and cost of male and female reproduction (Hultine et al. 2016). Additionally, spatial segregation of the sexes may cause physiological and morphological adaptations to different environmental conditions, further emphasizing dimorphic characters (Garbarino et al. 2015, Hultine et al. 2016).
In addition to extreme variability across environmental gradients, such as light availability (Coble and Cavaleri 2014), precipitation (Meier and Leuschner 2008) or temperature (Royer et al. 2009), leaf phenotypic traits have shown to be very variable between male and female individuals of dioecious plants as well (Li et al. 2007, Midgley 2010, Rabska et al. 2020). For many dioecious species, there is a general assumption that females are faced with an increased demand for higher reproductive investment, due to both flowering and fruit/cone bearing, fewer resources being allocated to other processes, such as growth or defense (Nicotra et al. 2003, Korgiopoulou et al. 2019). Therefore, differences in resource trade-offs and eco-physiological optima between sexes may directly influence vegetative growth (Moore and Pannell 2011) and leaf morphology (Ashman 2005, Midgley 2010, Rabska et al. 2020). Sex-specific adaptations to resource uptake, allocation and utilization, designed to meet the costs of reproduction, may be greatly influenced by increasingly pronounced climate change (Hultine et al. 2016), especially in the Mediterranean region.
Junipers, as species tolerant of drought and xeric conditions (Cano Ortiz et al. 2021), are common members of the Mediterranean landscape. Two of those species, commonly found throughout the Mediterranean basin, have until recently been considered synonymous: J. oxycedrus L. and J. deltoides R.P.Adams. The genetic separation of the two species was revealed using nrDNA sequencing, RAPDs (Adams 2004, Adams et al. 2005), simple sequence repeats, SSRs (Boratyński et al. 2014) and further confirmed by the analysis of needle terpenoids and morphology (Rajčević et al. 2013). When observing both species, differences in needle morphology and overall shape can be noted. Apart from having longer and narrower needles, J. oxycedrus is characterized by a tapered needle base. On the other hand, needle sides in J. deltoides are parallel or obtuse, giving them a triangular or deltoid appearance (Adams 2014b). An additional distinguishing character between the two species is a rounded crown shape in J. oxycedrus, as opposed to a pyramidal crown shape in J. deltoides. In addition, the cone scales of J. oxycedrus are smooth and do not protrude, whereas the cones of J. deltoides have protruding scales (Adams 2014b). Although both are widely distributed throughout their natural range, each covers a particular part of the Mediterranean basin, J. oxycedrus the western (from Northern Africa through Portugal to Italy), and J. deltoides the eastern (from Italy to Türkiye). Due to its central position in the Mediterranean region, the Apennine distribution of species is not entirely clear as it is likely that they are sympatric in some regions (Adams 2014b). Growing in the same, unfavorable xeric environments, on inaccessible limestone or siliceous terrain (Cano Ortiz et al. 2021) and commonly affected by wildfires, both of these species are under intensive anthropological influences (Rupprecht et al. 2011). With climate change affecting the Mediterranean basin more severely (Lange 2020), it is likely that some populations of both species will be under severe pressure, making it necessary to further explore their diversity, and resulting adaptability.
Although extensive previous research has been conducted on morphology (Klimko et al. 2004, 2007, Adams 2014b, Brus et al. 2011, 2016, Roma-Marzio et al. 2017), ecology (Muñoz-Reinoso 2003, Cano Ortiz et al. 2021), genetics (Boratyński et al. 2014, Adams et al. 2015), distribution models (Rupprecht et al. 2011) and studies on essential oils (Rajčević et al. 2013, Roma-Marzio et al. 2017, Semerdjieva et al. 2019), intersexual morphological differences between these two species are less well-known (Brus et al. 2011, 2016, Semerdjieva et al. 2019) but could provide insight into the adaptability of the species.
Therefore, in this research, morphometric analysis was performed on selected populations of J. oxycedrus from the western, and on populations of J. deltoides from the eastern Mediterranean, to test the degree of morphological distinctiveness among the taxa and sexes. Furthermore, phenotypic variation within and among populations was analyzed.
Material and sampling
Plant material used in this study was derived from 60 individuals from three natural populations of J. oxycedrus originating from the Provence-Alpes-Côte d'Azur region in southern France and 60 individuals from three natural populations of J. deltoides from the north-eastern coast of the Adriatic Sea. Each population included samples from 10 male and 10 female individuals. Both studied regions are characterized by a Mediterranean climate, with mild winters, dry and hot summers, and high insolation rates (Lionello et al. 2006). Juniperus oxycedrus populations are, compared to J. deltoides populations, located further inland and are found in areas of lower precipitation. In addition,the two study sites share very similar annual temperature values. Western Mediterranean populations representing J. oxycedrus included populations La Beaucet (P1), Grand Vallat (P2) and Les Plantades (P3) located in France. Juniperus deltoides populations in the Eastern Mediterranean included the island populations Cres (P4) and Krk (P5), as well as the coastal population Pula (P6), located in the Northern Adriatic region (Tab. 1, On-line Suppl. Fig. 1).
Tab. 1. Sampling sites, geographic coordinates, and multivariate diversity index (MDI) for six studied Juniperus oxycedrus and J. deltoides populations. Values followed by the same letters are not significantly different at P > 0.05 according to Wilcoxon Rank Sum test.
Needle and cone samples for morphometric analyses were collected during September and October of 2021. Great attention was paid to sampling only sexually mature individuals, in order to determine accurately the sex of the plant by the presence of cones or male strobili, or their residue on the branches (Brus et al. 2016). Several short shoots were cut from each individual on the sunlit side of the canopy, from which 20 random, fully developed and undamaged, one-year-old needles were selected from the central part of the shoot. Needles were first pressed and then glued to white paper, revealing the underside of the needles. Thus prepared, needles were measured and stored in the herbarium at the Faculty of Forestry and Wood Technology of the University of Zagreb (DEND). In addition, 20 mature cones were collected from female individuals. The number of scales was determined, and the cones were measured. The cones were then carefully opened, and seeds were extracted and counted. Out of the total seed count, 20 seeds per individual were randomly selected and measured. In total, 120 individuals, 2400 needles, 1200 cones and 1200 seeds were analyzed.
Studied phenotypic traits
Needle phenotypic traits were measured using the WinFolia program (WinFoliaTM 2001) with an accuracy of 0.1 mm. Measurement involved six traits related to needle size: needle area (NA); needle length (NL); maximum needle width (MNW); needle length, measured from the needle base to the point of maximum needle width (PMNW); and needle width at 50% (NW50) and 90% (NW90) of needle length; and two angles describing needle base NA10 and NA25, representing angles closed by the main needle vein and the line connecting the needle base to a set point on the needle margin, at 10% and 25% of total needle length, respectively.
Cone length (CL), cone width (CW) and seed thickness (ST) were measured using digital caliper (Alpha Tools®, Bahag AG, Germany), with an accuracy of 0.01 mm. The number of cone scales (NCS) and the number of seeds (NS) were counted manually, while seed length (SL) and seed width (SW) were determined using the WinSeedle program (WinSeedleTM 2011).
Statistical analysis
Pearson correlation coefficients were calculated among all needle and cone traits including all studied individuals using the CORR procedure in SAS v9.3 (SAS Institute 2011).
The phenotypic diversity of needles and cones was analyzed using descriptive statistics parameters, including arithmetic mean, standard deviation, minimum and maximum values, and coefficient of variation (%).
Six traits related to needle morphology and seven traits related to cones were subjected to analysis of variance using the MIXED procedure in SAS (SAS Institute 2011). For needle-related traits, the model included the effect of taxon (T), sex (S) and their interaction (T × S), population within taxon (P(T)) and population × sex interaction within taxon (P × S(T)) as fixed effects and individual (I) within taxon, population and sex (I(T × P × S)) as a random effect. For cone-related traits, the model included the effect of taxon (T) and population within taxon (P(T)) as fixed effects and individual (I) within taxon and population (I(T × P)) as a random effect. Partitioned F-tests (SLICE option) were conducted to examine the significance of the population × sex interaction within each taxon and each population (only in the case of needle-related traits) and of populations within each taxon (in the case of all traits).
Principal component analysis (PCA) was performed using the PRINCOMP procedure in SAS (SAS Institute 2011). The biplot was constructed by two principal components showing analyzed individuals and traits.
Morphological differentiation was assessed by calculating the Euclidean distances between all pairs of individuals based on the scores of the first two principal components (PCs) considering needle and cone traits. The average Euclidean distances were calculated for each population and taxon and used as a multivariate diversity index (MDI) of a population and taxon. The Kruskal-Wallis test (among all populations) and the Wilcoxon rank sum test (between all possible population pairs) were performed using the NPAR1WAY procedure in SAS (SAS Institute 2011), as was the Kruskal-Wallis test between studied taxa.
Discriminant analysis (DA) was performed to determine which of the needle and cone traits were most useful in maximizing discrimination between the studied taxa. The STEPDISC, DISCRIM and CANDISC procedures in SAS (SAS Institute 2011) were used for this purpose.
Results
Correlations
In order to determine how specific needle and cone variables correlated with each other, Pearson’s correlation coefficients were calculated. When observing correlations among needle traits (On-line Suppl. Tab. 1), statistically significant correlations ( P < 0.05) were determined among all of the studied traits except NA10 and MNW and NW50, as well as between NA25 and MNW, and NW50 and NW90. Of those, negative correlations were found only between traits related to needle base angles (NA10 and NA25) and NA, and NL and PMNW. Pearson's correlation coefficient value ( r) was above 0.7 in nine cases, and below -0.7 in two cases. Among analyzed cone traits, statistically significant correlations were found between all traits, except NCS and NS, and SW and ST, in addition to NS, which was correlated only with ST (On-line Suppl. Tab. 2). Eight pairs had the r value above 0.7. Furthermore, positive correlations were also found between all of the needle traits related to its size and all of the cone traits except NS, which was only correlated to NW90 (On-line Suppl. Tab. 3). Needle traits related to its shape were either not significantly or negatively correlated with cone traits. The trait NA10 was negatively correlated with CL, CW, SL, SW and ST, while NA25 was correlated only with ST.
Needle phenotypic traits
The results of morphological analyses of needles can be observed at the species, sex, and population levels. Observing the differences in needle dimensions and shape between the species, it is clear that J. oxycedrus has, on average, larger (0.19 mm2), longer (1.56 mm) and wider (0.17 mm) needles, compared to the smaller (0.12 mm2), shorter (1.17 mm) and narrower (0.14 mm) needles in J. deltioides (Tab. 2). In both species the most variable needle phenotypic traits were PMNW (CV = 49.18 and 76.02%, respectively) and NW90 (CV = 33.88 and 44.87%), while the least variable were MNW (CV = 15.51 and 19.03%) and NW50 (CV = 18.81 and 17.78%). In general, J. deltoides showed greater variability, with CVs ranging from 17.78 to 76.02%, than J. oxycedrus with a range from 15.51 to 49.18%.
Tab. 2. Descriptive statistics for analyzed needle traits for Juniperus oxycedrus and J. deltoides. X̄ – arithmetic mean; CV – coefficient of variation (%). Needle morphometric traits acronyms: NA – needle area; NL – needle length; MNW – maximum needle width; PMNW – needle length, measured from the needle base to the point of maximum needle width; NW50 – needle width at 50% of needle length; NW90 – needle width at 90% of needle length; NA10 – angle closed by the main needle vein and the line connecting the needle base to a set point on the needle margin at 10% of total needle length; NA25 – angle closed by the main needle vein and the line connecting the needle base to a set point on the needle margin at 25% of total needle length.
Sexual dimorphism was detected for the majority of measured needle traits, particularly in J. oxycedrus, where needles from female individuals displayed larger values for seven out of eight phenotypic traits (all traits except NA10). The distinction between the sexes was less pronounced in J. deltoides, where females had higher values in four of the measured phenotypic traits (NA, NL, PMNW and NW50), males in two (NA10 and NA25), while no difference was noted between two traits (MNW and NW90). In addition, male individuals of J. oxycedrus proved to be noticeably more variable than the females, having greater CV values of seven out of eight measured needle traits (all except NA). On the other hand, the degree of variability in J. deltoides was evenly distributed between males and females (Tab. 2).
Observing phenotypic characteristics of J. deltoides on a population level, it is evident that all three studied populations have the same mean value for NA (0.12 mm2) (On-line Suppl. Tab. 4). However, there are differences according to other measured needle traits. For example, the population Pula (P6) had the highest mean values for the five measured needle traits, mainly those related to the needle width (MNW, NW50 and NW90) and the shape of the base (NA10 and NA25). On the other hand, the population with the majority of the lowest values was Krk (P5) with six minimum mean values (MNW, PMNW, NW50, NW90, NA10, NA25). However, the latter population had the longest needles. According to the obtained CV values, the most variable population in terms of needle phenotypic traits was Krk (P5), with five maximal CV values (MNW, PMNW, NW90, NA10, NA25), while NA and NL were the most variable in population Pula (P6). The lowest variability was detected equally frequently in populations Cres (P4) (NA, NL, NA10, NA25) and Pula (P6) (MNW, PMNW, NW50, NW90). Coefficients of variability among sexes within studied populations of J. deltoides were greatest in population Krk (P5), where both male and female individuals had the majority of the maximum CV values for five and eight traits, respectively. On the other hand, the least variable males and females, needle-morphology-wise, were found in population Pula (P6), with the lowest values for six and four traits, respectively.
In J. oxycedrus, the biggest and the widest needles were characteristic of the population Les Plantades (P3), which had the highest mean values for NA, MNW, PMNW, NW50 and NW90. Needles of J. oxycedrus were the smallest and the shortest in population Grand Vallat (P2) and the narrowest in Le Beaucet (P1), while these two populations shared the lowest values for MNW, NW50 and NW90. The longest needles were typical of the population Le Beaucet (P1), while needle base angles were largest in the population Grand Vallat (P2). When traits’ variability is considered, the most variable population according to the obtained CV values was Grand Vallat (P2), with five maximum values (NA, NL, MNW, PMNW, NA10), followed by population Le Beaucet (P1) with three (NW50, NW90, NA25). According to coefficients of variability, the least variable population was Les Plantades (P3) with six minimal CV values (MNW, PMNW, NW50, NW90, NA10, NA25). Females had the largest needles in the population Les Plantades (P3) and the smallest in Grand Vallat (P2). Needles of male individuals were the longest and largest in the population Le Beaucet (P1), as opposed to the smallest and the shortest needles found in Les Plantades (P3), albeit the needles in P3 were the widest.
Cone phenotypic traits
Generally, when J. oxycedrus, is compared to J. deltoides, it excels in all studied characteristics of cones, from their dimensions, through the number of scales, to the dimensions and number of seeds (Tab. 3). On average, cones of J. oxycedrus were 2.09 mm longer and 1.13 mm wider, while seeds were 1.21 mm longer, 1.30 mm wider and 0.75 mm thicker than those of J. deltoides. In addition, dimensions of cones and seeds were less variable in J. oxycedrus, with coefficient of variation ranging from 11.21 to 11.79% and from 10.54 to 18.67%, respectively, compared to those of J. deltoides with CV values ranging from 13.66 to 14.37% for cone, and from 14.28 to 19.45% for seed dimensions. On the other hand, NCS and NS were less variable in J. deltoides, with CV values of 14.52 and 20.46%, respectively. Overall, the most variable cone trait in both species was NS, with CV values of 20.46 in J. deltoides and 30.88% in J. oxycedrus.
Tab. 3. Descriptive statistics for analyzed cone traits for Juniperus oxycedrus and J. deltoides. X̄ – arithmetic mean; CV – coefficient of variation (%). Cone morphometric traits acronyms: CL – cone length; CW – cone width; NCS – number of cone scales; NS – number of seeds; SL – seed length; SW – seed width; ST – seed thickness.
Observing individual populations of J. deltoides (On-line Suppl. Tab. 5), the largest cones and seeds, as well as the highest number of seeds, was present in population Cres (P4). Contrarily, the smallest cones were present in population Pula (P6), while the lowest number of seeds was detected in population Krk (P5). In addition, the lowest number of cone scales was detected in population Krk (P5), and the highest in population Pula (P6). The most variable population, according to cone traits, was Pula (P6), with five out of seven maximum CV values (CL, CW, NCS, SL, SW), while the least variable was population Cres (P4), with four of such traits (CW, NS, SW, ST). All of the measured cones in population Krk (P5) had three cone scales, resulting in a CV value of 0.00%.
In J. oxycedrus populations (On-line Suppl. Tab. 5), Les Plantades (P3) had the largest cones with the most cone scales and seeds, as well as the longest seeds. On the other hand, the smallest cone and seed dimensions were characteristic of the population Grand Vallat (P2). The highest number of cone scales was present in the population Les Plantades (P3), and the lowest one in the population Le Beaucet (P1). In general, the most variable was the population Le Beaucet (P1), with five maximum CV values (CW, NS, SL, SW and ST), as opposed to the least variable population of Les Plantades (P3), with four minimum CV values (CW, NS, SL, ST). The number of cone scales was the same for all of the measured cones in the population Le Beaucet (P1), generating a CV value of 0.00%.
Analysis of variance
As expected, the analysis conducted showed statistically significant differences in needle morphology between the two species, according to all of the measured needle traits (Tab. 4). Furthermore, significant differences in all traits, except MNW, were found between the sexes. However, sex differentiation was far less pronounced on the within-taxon level, with only three statistically significant differences (NL, NA10, NA25). Significant differentiation was also revealed on the within-population level, for all measured needle traits, except NA. Additionally, differences between populations and sexes within each taxon were found to be statistically significant only in MNW, NW50 and NW90.
Tab. 4. Results of the General Linear Model (GLM) for needle traits. Needle morphometric traits acronyms: NA – needle area; NL – needle length; MNW – maximum needle width; PMNW – needle length, measured from the needle base to the point of maximum needle width; NW50 – needle width at 50% of needle length; NW90 – needle width at 90% of needle length; NA10 – angle closed by the main needle vein and the line connecting the needle base to a set point on the needle margin at 10% of total needle length; NA25 – angle closed by the main needle vein and the line connecting the needle base to a set point on the needle margin at 25% of total needle length. df – degrees of freedom; F – F-test in GLM; P – significance of GLM's F-test.
Results clearly indicate a different number of distinguishable traits between the two sexes of both species, with sexes in J. oxycedrus distinguished by all of the measured needle traits on a statistically significant level, as opposed to sexes in J. deltoides, which were only differentiated by NL, PMNW, NA10 and NA25 (On-line Suppl. Tab. 6).
The population with the most significant differences between the sexes was P6 (NA, NL, PMNW, NW90, NA10, NA25), followed by P1 (MNW, NW50, NW90, NA25). Furthermore, sexes in populations P2 and P3 were distinguished by the lowest number of traits, MNW and NA and NL, respectively. Considering differences between populations within each taxon, it is clear that differentiation is more intensely pronounced in J. oxycedrus, with populations differing in all of the measured needle traits. On the other hand, populations of J. deltoides differ only in NA10 and NA25 (On-line Suppl. Tab. 6).
Cones of J. oxycedrus and J. deltoides differed significantly in all of the analyzed cone traits, except NS (Tab. 5). However, populations within each taxon were significantly differentiated only by traits NCS, SL and SW. Interestingly, cones of J. deltoides showed statistically significant differences only in SL, while cones of J. oxycedrus had four such traits (CL, CW, NCS, SW) (On-line Suppl. Tab. 7). Number of seeds demonstrated no significant variation, neither among taxa and populations nor within taxa.
Tab. 5. Results of the General Linear Model (GLM) for cone traits. Cone morphometric traits acronyms: CL – cone length; CW – cone width; NCS – number of cone scales; NS – number of seeds; SL – seed length; SW – seed width; ST – seed thickness. df – degrees of freedom; F – F-test in GLM; P – significance of GLM's F-test.
Principal component analysis
Principal component analysis (PCA) based on eight needle phenotypic traits in two juniper taxa populations revealed that the first two principal components had an eigenvalue greater than one and accounted for 89.59% of the total variation (Fig. 1A). Strong positive correlations ( r > 0.70) were observed between the first principal component (PC1) and the needle area (NA), position of maximal needle length (PMNW), needle length (NL), needle width at 50% (NW50) and 90% (NW90) of needle length. For the same principal component, a strong negative correlation was observed only for one phenotypic trait – needle angle at 10% of needle length (NA10). The second principal component (PC2) was strongly positively correlated with the needle angle at 25% of needle length (NA25). Biplot revealed a clear grouping of the individuals according to their respective taxa (Fig. 1A). Within taxon, significant overlapping has been demonstrated. In addition, males and females of each population overlapped with one another, i.e., demonstrated the same grouping trends. The slight divergence between males and females is only noticeable in population P1, with males partially separating.
The second PCA included seven cone phenotypic traits of the juniper species studied. The first two principal components had an eigenvalue greater than one and described 76.54% of the total variation (Fig. 1B). The first principal component (PC1) was in a strong positive correlation with five out of seven measured cone phenotypic traits (CL, SL, SW, CW, ST), and explained 61.87% of the total variability. No negative correlations were determined between the first principal component and cone traits. On the other hand, the second principal component (PC2), which accounted for 14.67% of total variability, was in a strong negative correlation with seed thickness (ST) and seed width (SW). Pronounced positive correlations were found with the number of cone scales (NCS) and the number of seeds (NS). The constructed biplot demonstrates clear clustering of individuals by their respective taxa (Fig. 1B). Interestingly, some individuals of J. deltoides have grouped with J. oxycedrus, mainly from populations P4 and P6. On a population level, a greater level of divergence was shown, compared to needles, especially in P1 and P3.
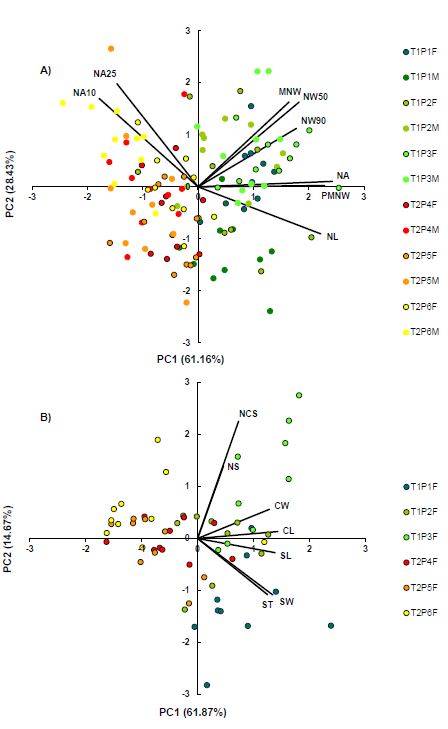
Fig. 1. Results of the principal component (PC) analysis based on (A) eight needle and (B) seven cone morphometric traits in studied Juniperus oxycedrus and J. deltoides populations. Needle morphometric traits acronyms: NA – needle area; NL – needle length; MNW – maximum needle width; PMNW – needle length, measured from the needle base to the point of maximum needle width; NW50 – needle width at 50% of needle length; NW90 – needle width at 90% of needle length; NA10 – angle closed by the main needle vein and the line connecting the needle base to a set point on the needle margin at 10% of total needle length; NA25 – angle closed by the main needle vein and the line connecting the needle base to a set point on the needle margin at 25% of total needle length. Cone morphometric traits acronyms: CL – cone length; CW – cone width; NCS – number of cone scales; NS – number of seeds; SL – seed length; SW – seed width; ST – seed thickness. T1 – J. oxycedrus; T2 – J. deltoides. F – female; M – male. Populations: P1 – Le Beaucet; P2 – Grand Vallat; P3 – Les Plantades; P4 – Cres; P5 – Krk; P6 – Pula.
Multivariate diversity index
To explore the diversity of individuals within the studied populations and taxa multivariate diversity index (MDI) was used. Statistically significant differences were confirmed for both the needles and the cones, on both the intrapopulation and the intrataxon levels. When considering the diversity of individuals within each taxon, based on the morphology of both needles and cones, J. oxycedrus showed to be more diverse, demonstrating higher MDI values than those of J. deltoides, 1.506 and 1.772, as opposed to 1.385 and 1.110, respectively. On the population level, both J. oxycedrus and J. deltoides demonstrated MDI values within a similar range of data, i.e., populations of both species were similarly diverse. For needles, MDI values in J. oxycedrus populations ranged from 1.172 to 1.551, whereas J. deltoides ranged from 1.200 to 1.447. Similar results were obtained for cones as well, with respective species’ populations ranges of 1.070 to 1.501, and 0.909 to 1.236 (Tab. 1).
Discriminant analysis (DA)
According to conducted discriminant analysis (DA), the needle traits that separated the two species the best were MNW, NW90, NW50, NA10 and NA25. Individuals of J. oxycedrus were characterized by wider needles, while individuals of J. deltoides had a wider needle base. Canonical discriminant analysis based on five traits showed that the first CV explained 100% of the variation between taxa. This is demonstrated also in the diagram (Fig. 2A), where a clear distinction between the two species along the first discriminant axis is visible. Significant overlap was detected on both population and sex levels, with no clear structuring. After the cross-validation, the discriminant function correctly classified 94.17% plants into their respective taxon. Individuals of J. oxycedrus were correctly classified in 91.67% cases, while J. deltoides showed even greater correct classification of 96.67%.
Cone traits that best distinguished species according to discriminant analysis were CL, SW and CW. All of the three mentioned traits showed greater values in J. oxycedrus. Canonical discriminant analysis based on three traits showed that the first CV explained 100% of the variation between taxa. As shown in the diagram (Fig. 2B), the two species were clearly separated by cone phenotypic traits as well, with negligible overlapping. However, no structuring was detected on the population level. Discriminant function correctly classified 93.10% plants into their respective taxon.
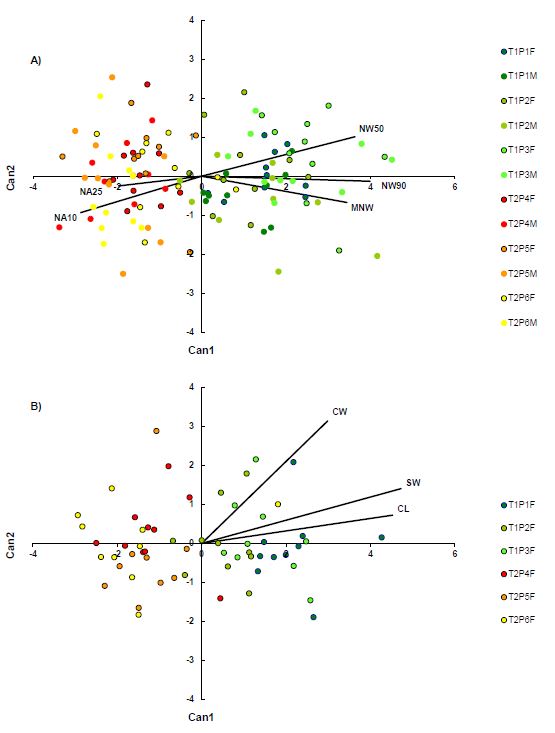
Fig. 2. The first two canonical variates of the canonical discriminant analysis (Can1 and Can2) of three Juniperus oxycedrus and three J. deltoides populations based on (A) five needle and (B) three cone morphometric traits. Each individual shrub is indicated by a small circle. Needle morphometric traits acronyms: MNW – maximum needle width; NW50 – needle width at 50% of needle length; NW90 – needle width at 90% of needle length; NA10 – angle closed by the main needle vein and the line connecting the needle base to a set point on the needle margin at 10% of total needle length; NA25 – angle closed by the main needle vein and the line connecting the needle base to a set point on the needle margin at 25% of total needle length. Cone morphometric traits acronyms: CL – cone length; CW – cone width; SW – seed width. T1 – J. oxycedrus; T2 – J. deltoides. F – female; M – male. Populations: P1 – Le Beaucet; P2 – Grand Vallat; P3 – Les Plantades; P4 – Cres; P5 – Krk; P6 – Pula.
Discussion
As expected, morphological differentiation between J. oxycedrus and J. deltoides was evident in all of the studied needle and cone traits, which further confirms the existence of the two separate species, first described by Adams (2004). Compared to J. oxycedrus, J. deltoides tend to have shorter and wider needles, with deltoid base shape, as well as visible cone scales with protruded tips and glaucous powder cover (Adams 2014b). Although the morphological differences between these two allopatric, cryptic species are very small and seemingly imperceptible, their differentiation is widely accepted and has been confirmed by genetics (Adams et al. 2003, 2005, Mao et al. 2010, Boratyński et al. 2014, Adams et al. 2015) and essential oil research (Adams et al. 2003, 2005, Rajčević et al. 2013). The divergence time between the two species has been estimated at approximately 8–10 Mya, which suggests a separate colonization of the western and eastern parts of the Mediterranean (Mao et al. 2010). Namely, during the late Miocene, the formation of the Alps occurred, causing the ancestral taxa to separate into two groups.
Average values of the needle and cone dimensions of J. deltoides, even though slightly lower, did not differ significantly from the previously reported values (Klimko et al. 2007, Brus et al. 2011, Adams 2014b, Brus et al. 2016, Roma-Marzio et al. 2017). However, contrary to mentioned previous reports, we determined narrower needles in J. deltoides. On the other hand, the opposite has been determined for J. oxycedrus, where obtained needle and cone dimensions slightly positively deviate from prior reported values (Adams 2014b, Roma-Marzio et al. 2017). In both species, seed dimensions were somewhat larger than those reported by Roma-Marzio et al. (2017).
Overall, needles and cones of J. deltoides were shown to have higher coefficients of variability than those of J. oxycedrus. Similar results were obtained by Klimko et al. (2007), who investigated the variability of J. oxycedrus s.l. throughout the Mediterranean. Although their study included a larger number of populations, they did not cover specific regions of southeastern France and northern Adriatic, unlike in this research. However, since the results are congruent, it is reasonable to assume the same trend would be present throughout the entire distribution range of the two species. Nevertheless, Boratyński et al. (2014) reported greater variability in J. oxycedrus, supported by a higher level of genetic diversity of Juniperus species in the western Mediterranean, as opposed to the eastern Mediterranean. This result is in accordance with the MDI values obtained in our research, which indicate higher overall variability of J. oxycedrus as well. The loss of genetic diversity in the east after the emergence of the Alps could be due to processes like the founder effects resulting from repeated climatic oscillations (Zhang et al. 2005), microevolutionary changes arising from different environmental pressures and limited gene flow caused by range fragmentation (Boratyński et al. 2014).
In this study, significant sex-based differences in needle morphometric traits were observed in all of the measured traits except maximal needle width. In both species, greater needle area and length were characteristic of the female individuals. The same pattern was previously confirmed in another dioecious conifer species, Taxus baccata L. (Iszkuło et al. 2009, Nowak-Dyjeta et al. 2017, Stefanović et al. 2017), as well as in some angiosperm species like Simmondsia chinensis (Link) C.K.Schneid. (Kohorn 1994). Larger needles in females are commonly believed to be the result of a need for greater assimilation area, required to support the reproductive efforts of females (Nowak-Dyjeta et al. 2017), as it is known that more resources are required to successfully mature fruit/cone than to produce pollen (Ashman 2005). In addition, Garbarino et al. (2015) proposed that growth in females could be favored by their occupation of more productive microhabitats, as opposed to not so fastidious male individuals. This could be further emphasized by improved soil properties under the female individuals, due to input of organic matter from the littered cones, as they are two to three times richer in nitrogen and potassium than needles and twigs (Gauquelin et al. 2002). Moreover, as wind-pollinated species, male trees of junipers are likely to be investing more resources into reproduction, due to the higher quantity and quality of reproductive material needed (Popp and Reinartz 1988, Gauquelin et al. 2002), therefore leaving fewer nutrients and less energy for foliar growth and ultimately resulting in smaller leaves (Harris and Pannell 2008). However, sexual dimorphism in this study was not detected uniformly in all of the populations. On the one hand, populations Le Beaucet (P1) and Pula (P6) displayed a significant number of gender-based differences in needle morphology, while other populations showed rather few differences. Diverse results between populations could be based on variable reproductive investment intensity of individuals of dioecious species, depending on many factors, like environmental and habitat conditions (Montesinos et al. 2011), plant size (Shibata and Kudo 2016), resource availability and pollination success (Rodríguez-García et al. 2018) and flowering and fruiting abundance (Montesinos et al. 2012). Therefore, secondary sex differences in dioecious plants are associated with trade-offs between the cost of reproduction and other plant functions, like growth, metabolism processes and defense (Maldonado-López et al. 2014, Liu et al. 2021).
In addition to significant phenotypic variability between both species and sexes, populations of the researched species demonstrated high levels of interpopulation diversity. All of the studied within-taxa populations differed significantly, according to all of the measured needle traits except NA, and three of the seven cone traits (NCS, SL, SW). Interpopulation diversity, however, is not uniform in the two taxa. Populations of J. oxycedrus differ significantly from each other according to all of the studied morphological traits, while differentiation in J. deltoides populations is present only according to two of the studied traits. This is likely the result of diminished gene flow or, alternatively, significant adaptations to specific climatic and edaphic conditions of each microhabitat (Kawecki and Ebert 2004). The lower levels of diversity noted in J. deltoides populations could be the result of their location, in the marginal, northernmost part of the species’ distribution (Vilar et al. 2016). In such conditions, lower levels of variability are to be expected (Brus et al. 2011) due to additional selective pressures during which various events, such as the bottleneck effect, might have arisen (Abeli et al 2014), therefore impacting the allelic richness and subsequent variability of phenotype. Higher variability in morphological traits detected among J. oxycedrus populations could be explained by a higher level of overall genetic diversity of Juniperus species in the western Mediterranean than in the eastern (Boratyński et al. 2014), combined with processes like local adaptation (Savolainen et al. 2013) and phenotypic plasticity (Radersma et al. 2020). Although the results indicate higher variability in J. oxycedrus in relation to J. deltoides, no uniform trend was observed at the intrapopulation level, since some populations within both taxa were more or less variable, differing also between needle and cone traits.
Taxonomical complexity of junipers in these regions is further complicated by various other Juniperus taxa within the Juniperus (syn. Oxycedrus Spach) section that are widely distributed across Mediterranean region. According to Klimko et al. (2007) there are four subspecies within the J. oxycedrus: subsp. oxycedrus, subsp. badia (H.Gay) Debeaux; subsp. transtagana Franco and subsp. macrocarpa (Sm.) Ball. Nowadays, however, the latter two subspecies are recognized at species level, J. navicularis Gand and J. macrocarpa Sm., while former two subspecies had their status changed to varieties (WFO 2023). Furthermore, on the north-eastern coast of Adriatic Sea, sporadic reports of J. macrocarpa have been made (Topić and Šegulja 2000). However, none of these reports were confirmed at a later date during the research of Brus et al. (2016) and Roma-Marzio et al. (2017). As a result, there is a lack of conclusive proof of J. macrocarpa presence as far north as the north-eastern Adriatic coast. On the other hand, only the typical variety of J. oxycedrus has been reported in France (Boratyński et al. 2014, Cano Ortiz et al. 2021), with the exception of reports from Corsica. Its taxonomical congruence is, therefore, under certain terms.
Conclusions
The differences between the J. oxycedrus and J. deltoides have been confirmed in this research, with J. oxycedrus having larger needles and cones, although less variable, than those of J. deltoides. Furthermore, sexual dimorphism in needle morphology was found in both species, but more so in J. oxycedrus. In general, female individuals demonstrated higher trait values than males in both species. In addition to significant phenotypic variability between both species and sexes, populations of the researched species demonstrated high levels of interpopulation variability, especially in J. oxycedrus, while intrapopulation variability showed no uniform pattern between the two species.
The results of this research support earlier delimitations of the two taxa, in addition to opening new questions about the possible influence of gender imbalance on the morphological diversity and variability within and between the different taxa. Additional research into genetic variability could be used to further discern the specifics of the intra- and interpopulation variability.