Introduction
Climate changes and human activities such as deforestation, urbanization, industrial activities and agriculture often lead to the extinction of plant species that are unable to survive or reproduce in a degraded ecosystem. Particularly, species with narrow geographical ranges are at higher risk of extinction (Newbold et al. 2018). Among threatened species, a critical factor driving extinction is the failure to replenish populations with new individuals. Therefore, pinpointing the reasons for species' inability to regenerate is paramount for the protection and restoration of endangered species. Seed characteristics are directly related to the conservation and population growth of threatened plants (Cochrane et al. 2014). Successful preservation and the establishment of ex situ conservation of endangered plants primarily depends on successful germination and seedling establishment (Güleryüz et al. 2021). Understanding the specific requirements for successful germination is imperative for effectively conserving these plants (Cochrane et al. 2002).
Seed dormancy, which delays germination and inhibits seedling development, is an evolutionary adjustment that helps seed persistence through adverse conditions ensuring germination occurs when environmental situations become more favourable for seedling establishment (Kildisheva et al. 2020). However, during the dormancy period, seeds are often lost due to animal consumption, attack by microorganisms and environmental stresses, limiting the ability of plants to expand their population (Chen et al. 2022). Comprehending the seed dormancy type enables environmentalists to improve targeted strategies to break dormancy and stimulate germination. In accordance with the modified version of the classification of seed dormancy proposed by Baskin and Baskin (2004), seed dormancy can be categorized into five classes: morphological dormancy (MD), physiological dormancy (PD), morphophysiological dormancy (MPD), physical dormancy (PY) and combinational dormancy (PY + PD) (Baskin and Baskin 2004). Recently, Baskin and Baskin (2021) presented an expanded system for primary seed dormancy classification, which includes a greater diversity of subclasses, levels and types (Baskin and Baskin 2021). The Convolvulaceae, which includes two subfamilies: Convolvuloideae and Hombertoideae, is known for seeds with physical dormancy. Physical dormancy occurs when seeds are unable to germinate due to physical barriers, often caused by a water-impermeable seed coat (Jayasuriya et al. 2009). Previous studies have identified physical dormancy in seeds of Calystegia sepium (L.) R. Br., Calystegia soldanella (L.) Roem. & Schult. and Convolvulus arvensis L. from the tribe Convolvuleae within the subfamily Convolvuloideae (Jayasuriya et al. 2008a). In view of these findings, as well as of the presence of a hard seed coat in C. persicus, it is likely that physical dormancy is present in the seeds of this species. Depending on the type of dormancy, some treatments such as seed coat scarification, cold and warm stratification and different chemical treatments can overcome dormancy barriers (Fernández and Tapias 2022).
Convolvulus persicus L. (Convolvulaceae) is an endemic endangered perennial species that grows in the coastal regions of the Caspian Sea and the Black Sea. It is threatened due to the restricted ranges and destruction of its environment by human actions and natural conditions. Plants inhabiting coastal dune ecosystems are exposed to harsh natural conditions, including soil salinity, salt spray, nutrient deficiency, sand burial, sea waves and flooding, strong winds and high light intensity (Hesp 1991). The plant produces ovate-elliptical and short petioled leaves and white, funnel-shaped and bisexual flowers (Strat and Holobiuc 2018). Previous studies have demonstrated that C. persicus produces few or no seedlings in its natural habitat on the southern coast of Caspian Sea in Iran and the Danube delta shore in Romania. In fact, C. persicus reproduces primarily through asexual reproduction via rhizome buds (Strat and Holobiuc 2018, Asheqian et al. 2021). Field surveys conducted on the Iranian coast of the Caspian Sea revealed a significantly low seed production percentage in C. persicus compared to its flower production percentage. Additionally, seed germination percentage across three populations of C. persicus along the southern coastline of the Caspian Sea was found to be poor (< 10%) (Asheqian et al. 2021). Collectively, a large number of unfertilized flowers, low seed production rate, low seed germination percentage, harsh coastal habitat conditions and human actions increase the extinction risk of C. persicus. Consequently, this species is categorized as critically endangered across various regions including Iran, Azerbaijan, Romania, Bulgaria, and Turkey (Holobiuc et al. 2015, Sayadi and Mehrabian 2017, Strat and Holobiuc 2018, Asheqian et al. 2021).
The primary objective of this study was to elucidate the dormancy status and germination requirements of C. persicus, thereby offering valuable insights for conservation and collection management efforts. To our knowledge, this research is the initial attempt to investigate the dormancy type and enhance the seed germination rate of C. persicus seeds. We hypothesized that the thick coat of C. persicus seeds is impermeable, which results in physical dormancy. Thus, application of various treatments that can lead to seed coat permeability, ultimately can lead to seed dormancy release. We put forward the hypothesis that germination capacity depends on seed traits, which are influenced by the climate conditions of plant communities. Therefore, we expected that seeds from different populations would exhibit varying seed qualities and germination percentage. To assess our hypothesis, our study focused on four key aspects: (1) evaluation of seed characteristics; (2) assessment if seed coat permeability; (3) investigation of the effects of temperature on fresh seed germination and (4) application of different dormancy release methods including scarification, sulphuric acid, cold and warm stratification and gibberellic acid.
Materials and methods
Seed collection
Seeds were obtained from two different localities (Goharbaran Sari, 36˚50.054' N, 53˚12.9681' E and Naftchal Babolsar, 36˚44.0311' N, 52˚47.6602' E) situated in the Mazandaran province of Iran in July 2022. Climatic factors for these areas are presented in Tab. 1, sourced from the Mazandaran meteorology general office. The collected seeds were stored in dark glass bottles at 5 °C for a duration of 6 months before utilization.
Tab. 1. Climatic factors of two different localities, Babolsar and Sari (average values for the 2020-2022 period) situated in the Mazandaran province of Iran.
Determination of seed characteristics
Seed dimensions including width, length and thickness were determined by a Vernier calliper with an accuracy of 0.02 mm. Ten randomly selected seeds were weighed from each population using an electronic balance. Tetrazolium test was utilized for assessment seed viability by analysing the intensity of coloration in stain embryos. Colourless 2,3,5-triphenyl-2H-tetrazolium chloride (TTC) can penetrate intact membranes and change into red coloured formazan in living cells, a process catalysed by dehydrogenase enzymes concerned with aerobic respiration. In seeds, cells that are metabolically active will exhibit a pink or red staining pattern (Kittock and Law 1968). For tetrazolium testing, seeds were imbibed for 24 h in distilled water. Subsequently they were transferred to 1% (w/v) TTC solution and kept for 24 h at room temperature. Red colouring and uniformity of staining were used to determine seed viability as shown in Fig. 1 (ISTA 2003).
Germination test
To evaluate the germination potential of fresh seeds (within 14 days of seed collection), a preliminary germination test was performed for each population. Seeds were sterilized with sodium hypochlorite solution (1%) for 3 minutes and subsequently rinsed three times with sterilized water. Four replicates of 10 sterilized seeds were situated in 8 cm Petri plates on double filter paper and moistened with 3 mL of distilled water. Seeds were incubated at different temperatures: 10, 15, 20, 25, 30 and 40 °C for 4 weeks.
Water imbibition test
Seed imbibition mechanically scarified seeds and control seeds was compared to assess seed coat permeability. Each set contained three replicates of ten seeds. Seeds in each replicate were initially weighed and were placed onto moistened filter paper in Petri plates and kept under identical conditions. The seeds were removed, dried gently with a paper towel and reweighed after 2, 4, 6, 8, 10, 12, 24, 48, and 72 h. Percent increase of fresh mass was calculated using the equation:
%Wr = [(Wf–Wi)/Wi] ×100,
where Wr is percent increase in mass, Wi is initial fresh weight prior to imbibition and Wf is fresh mass following a specifc period of imbibition (Zhang et al. 2015).
Dormancy-breaking treatment
To disrupt the seed coat and break the seed dormancy, various physical and chemical treatments were applied (Kildisheva et al. 2020). For each treatment four replicates of ten seeds were considered.
Primary treatments:
- Scarification: seeds were mechanically scarified by sandpaper (N150) in two separated treatments for 3 and 5 minutes.
- Sulfuric acid (H2SO4): seeds were soaked in 90% H2SO4 in two separate treatments for 5 and 15 minutes.
- Cold stratification: seeds were incubated at 4 ℃ in two separate treatments for 4 and 8 weeks.
- Warm stratification: seeds were incubated at a fixed temperature of 28 ℃ in two separated treatments for 4 and 8 weeks.
- Gibberellic acid (GA3): seeds were soaked in 300 and 600 mg L-1 GA3 for 48 h.
Combined treatments:
- Mechanical scarification (5 min) + 25% H2SO4 (15 min) + cold stratification (4 weeks).
- Mechanical scarification (5 min) + 25% H2SO4 (15 min) + warm stratification (4 weeks).
- GA3 (300 and 600 mg L-1) + cold stratification (4 weeks).
- GA3 (300 and 600 mg L-1) + warm stratification (4 weeks).
Control included sterilized and untreated seeds. Both control and treated seeds were placed in a growth chamber under controlled conditions (22-24 ℃, 14 h light/10 h dark cycle).
Germination was monitored daily and the test concluded after 30 days. Germination was defined as radicle protrusion (> 2 mm). Percentage of germination, rate of germination and seed vigour were calculated using the following formulae:
where GP is germination percentage; N is the total number of seeds and n is the number of germinated seeds.
where GR is germination rate; ni is the number of germinated seeds after i days and Ti is the number of days from the beginning of the test.
where SV is seed vigour, LS is the mean of seedling length and GP is the germination percentage (Abdul-Baki and Anderson 1973).
Statistical analysis
Statistical analyses were conducted using the SPSS version 25.0 (IBM Corporation, Armonk, NY, USA). The normality of the variable distribution was assessed using the Kolmogorov-Smirnov test. To compare seed qualities between the two collection sites (Sari and Babolsar), an independent-sample t-test was employed. A one-way analysis of variance (ANOVA), followed by Duncan’s multiple range test, was performed to test the differences of seed germination percentage at various temperatures, water uptake between control and scarified seeds of both sites. Furthermore, a one-way ANOVA was performed to determine whether various dormancy-breaking treatments significantly increased the final germination percentage (GP, %), germination rate (GR) and seed vigour (SV) relative to control seeds. Statistical significance was set at P < 0.05. All data were expressed as the means ± standard error (SE) of the four independent replicates except for the imbibition test which was conducted with three replicates.
Results
Seed characteristics
The seeds of C. persicus are enclosed within capsules that split open upon maturity. These seeds typically appear large, black and sometimes dark brown (Figs. 1A-D).
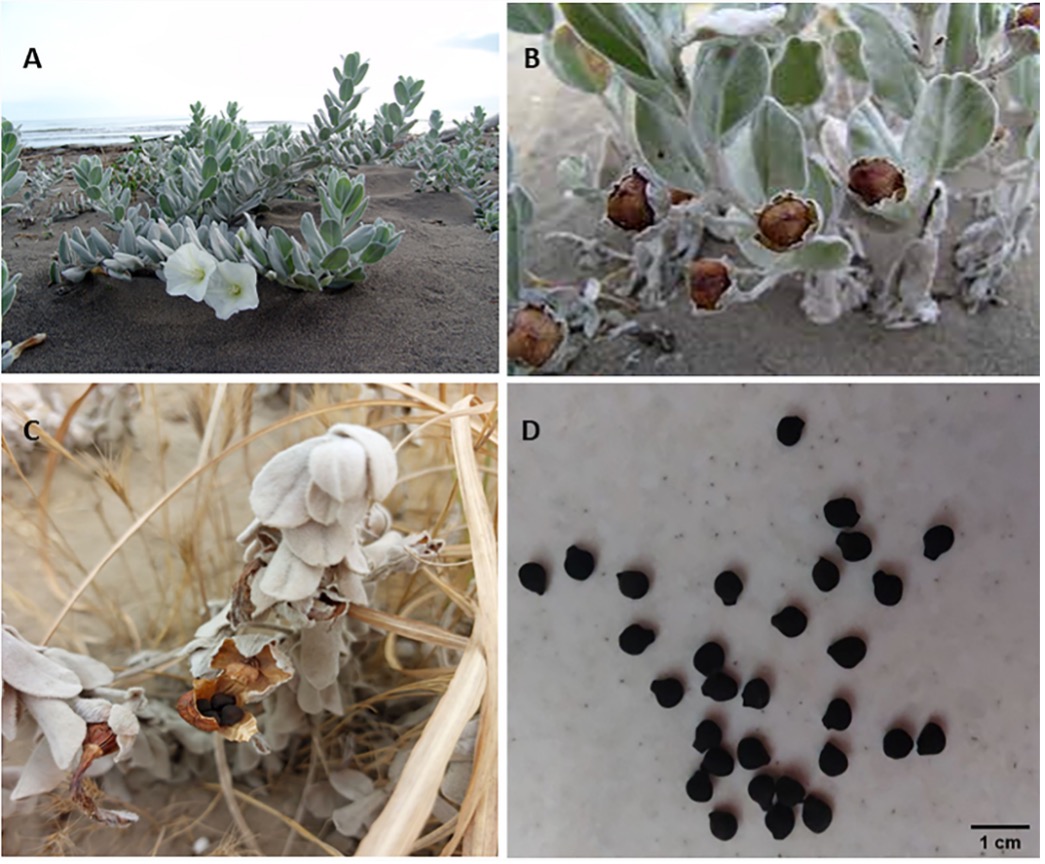
Fig. 1. Convolvulus persicus in the Mazandaran province of Iran: mature plants (A), fruits (B), capsule dehiscence (C) and seeds (D).
The length, width, thickness and weight of C. persicus seeds obtained from Sari were significantly higher than those from Babolsar (P < 0.05, Tab. 2). The viability of C. persicus seeds collected from two sites did not differ significantly (Tab. 2).
Tab. 2. Seed characteristics of Convolvulus persicus from two different localities in the Mazandaran province of Iran. Data represents the mean ± standard error (n = 4). Student's t-test was used to determine the significance between means (*) at P < 0.05, n.s. - not significant.
Germination test
Statistical analysis did not reveal significant differences in germination percentage at 15, 20, 25, 30 °C (P = 0.08). Seeds incubated at low and high temperatures (10 and 40 °C) did not germinate. In general, the germination percentage at different temperatures in both populations did not exceed 10% (Fig. 2).

Fig. 2. Effect of temperature on seed germination of Convolvulus persicusfrom two localities in the Mazandaran province of Iran. Data represent the mean ± standard error (n =4). Different letters indicate significant differences at P < 0.05 (one-way ANOVA with Duncan’s post hoc test).
Water imbibition test
Significant differences were observed in water absorption rates between early and late hours of imbibition in intact and mechanically scarified seeds within both populations (P < 0.05, Fig. 3). Scarified seeds demonstrated a higher and faster rate of water absorption compared to the non-scarified seeds in both the Babolsar and Sari populations. Mechanical scarification resulted in a significant increase in water uptake compared to the control after 72 h. By the end of the 72-h observation period, scarified seeds showed water uptake of 62 ± 2.13% for Babolsar and 75 ± 2.66% for Sari, whereas non-scarified seeds showed an increase of only 24 ± 1.37% for Babolsar and 28 ± 1.74% for Sari (P < 0.05, Fig. 3).
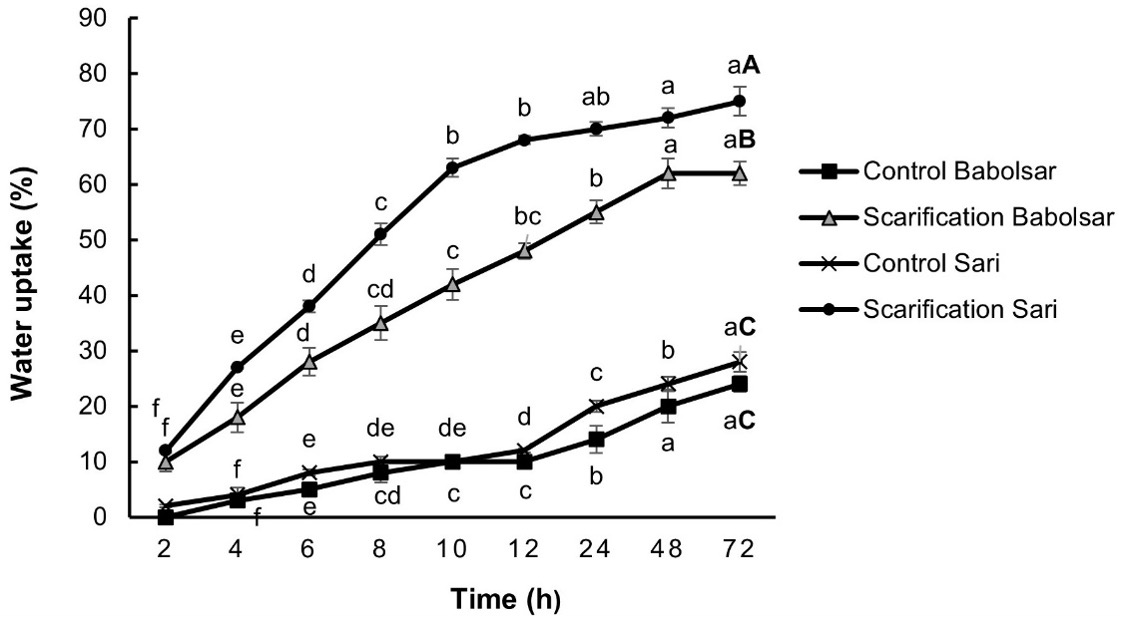
Fig. 3. Water uptake for control and scarified seeds of Convolvulus persicus from two localities in the Mazandaran province of Iran. Data represent the mean ± standard error (n =3). Small letters indicate comparison of water uptake (%) in each time within a group and capital letters represent comparison water uptake (%) among groups after 72 hours at P < 0.05 (one-way ANOVA with Duncan’s post hoc test).
Dormancy-breaking treatments
Considering primary treatments ANOVA analysis showed that mechanical scarification for 3 min had no significant effect on germination percentage when compared to the control in both Babolsar and Sari populations (5% and 7.5%, respectively, P > 0.05). But application of mechanical scarification for 5 min exhibited a statistically significant impact on germination percentage of both Babolsar and Sari populations (27% and 22%, respectively, P < 0.05).
In seeds pretreated with 90% H2SO4 (for 5 and 15 min) the germination percentage did not significantly differ from that of the control and this result was identical in both populations (P > 0.05, Fig. 4).
The results showed that the germination percentage in seeds under GA3 treatments was less or equal to control regardless of the concentration used and GA3 did not improve seed germination percentage in either population, Babolsar or Sari (P > 0.05, Fig. 4(.
In contrast, cold and warm stratification significantly (P < 0.05) increased germination percentage in both populations, with warm stratification showing higher efficacy than cold stratification. In Babolsar, germination percentage significantly increased under cold stratification from 5% to 20 ± 4% and 17.5 ± 4.7%, for 4 and 8 weeks, respectively. While under warm stratification it increased to 25% for both 4- and 8-weeks duration. The results of the cold and warm stratification treatments on seeds from Sari revealed that the germination percentage was enhanced from 10% to 20% and 22% by 4- and 8-week cold stratification, respectively and improved to 35% and 27% under 4- and 8-week warm stratification, respectively. Seeds of C. persicus collected from Sari had the highest germination percentage (35 ± 2.8%) under 4-week warm stratification (P < 0.05, Fig. 4).
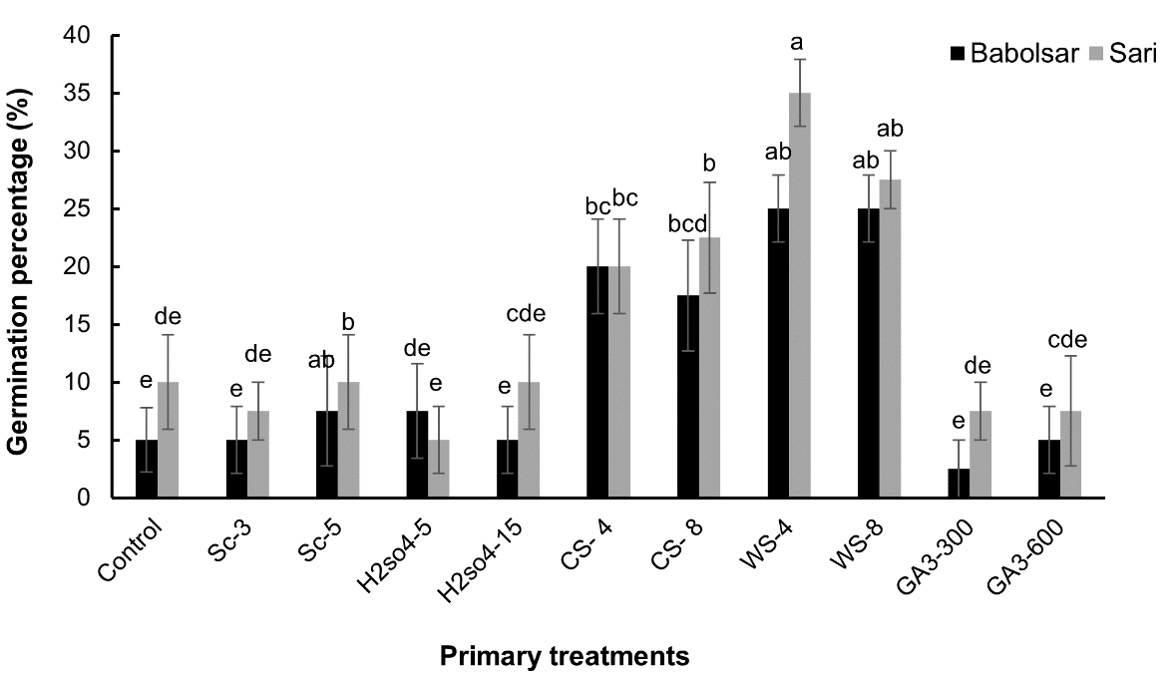
Fig. 4. The seed germination percentage of Convolvulus persicus under different physical and chemical treatments in two populations from Mazandaran province of Iran. Data represent the mean ± standard error (n = 4). Mean values with different letters differ significantly at P < 0.05 according to one-way ANOVA with Duncan’s post hoc test. Sc − mechanical scarification (3 and 5 min), H2SO4 − application of sulfuric acid (5 and 15 min), CS − cold stratification (4 °C, 4 and 8 weeks), WS − warm stratification (28 °C, 4 and 8 weeks), GA3 − application of gibberellic acid (300 and 600 mg L-1).
Following the assessment of scarification for 5 min and cold and warm stratification on seed dormancy release of C. persicus, combined treatments were administered involving scarification along with cold and warm stratification for 4 weeks. The result of one-way ANOVA revealed that mechanical scarification in combination with H2SO4 and cold stratification greatly improved the germination of C. persicus seeds by 71 ± 3.8% and 84 ± 3.1% (P < 0.05) in both populations, Babolsar and Sari, respectively. In addition, the mechanical scarification in combination with H2SO4 and warm stratification led to a substantial rise in germination percentage for Babolsar and Sari seeds, reaching 71 ± 3.1% and 81 ± 3.6%, respectively. However, no statistically significant difference was found in the germination percentage between the two mentioned treatments (P > 0.05, Fig. 5A).
The seed germination percentage of C. persicus under GA3 in combination with cold stratification treatment increased significantly (P < 0.05) by 34 ± 5.9% and 40 ± 3.1% for 300 mg L-1 GA3 and 43 ± 3.6% and 37 ± 8.8% for 600 mg L-1 GA3, for Babolsar and Sari, respectively (Fig. 5A). Exposure to GA3 in combination with warm stratification treatment also significantly (P < 0.05) improved the seed germination of C. persicus in both populations (Fig. 5A) in which the germination percentage of Babolsar treated seeds were 40 ± 5.9% and 37 ± 7.21% (for 300 and 600 mg L-1 GA3, respectively) and for Sari treated seeds were 40 ± 5.9% and 50 ± 5.1%, (for 300 and 600 mg L-1 GA3, respectively). However, the germination percentage of C. persicus seeds was unaffected by concentration of GA3 and was not different (P > 0.05) for two concentrations (300 and 600 mg L-1) of GA3, Fig. 5A).
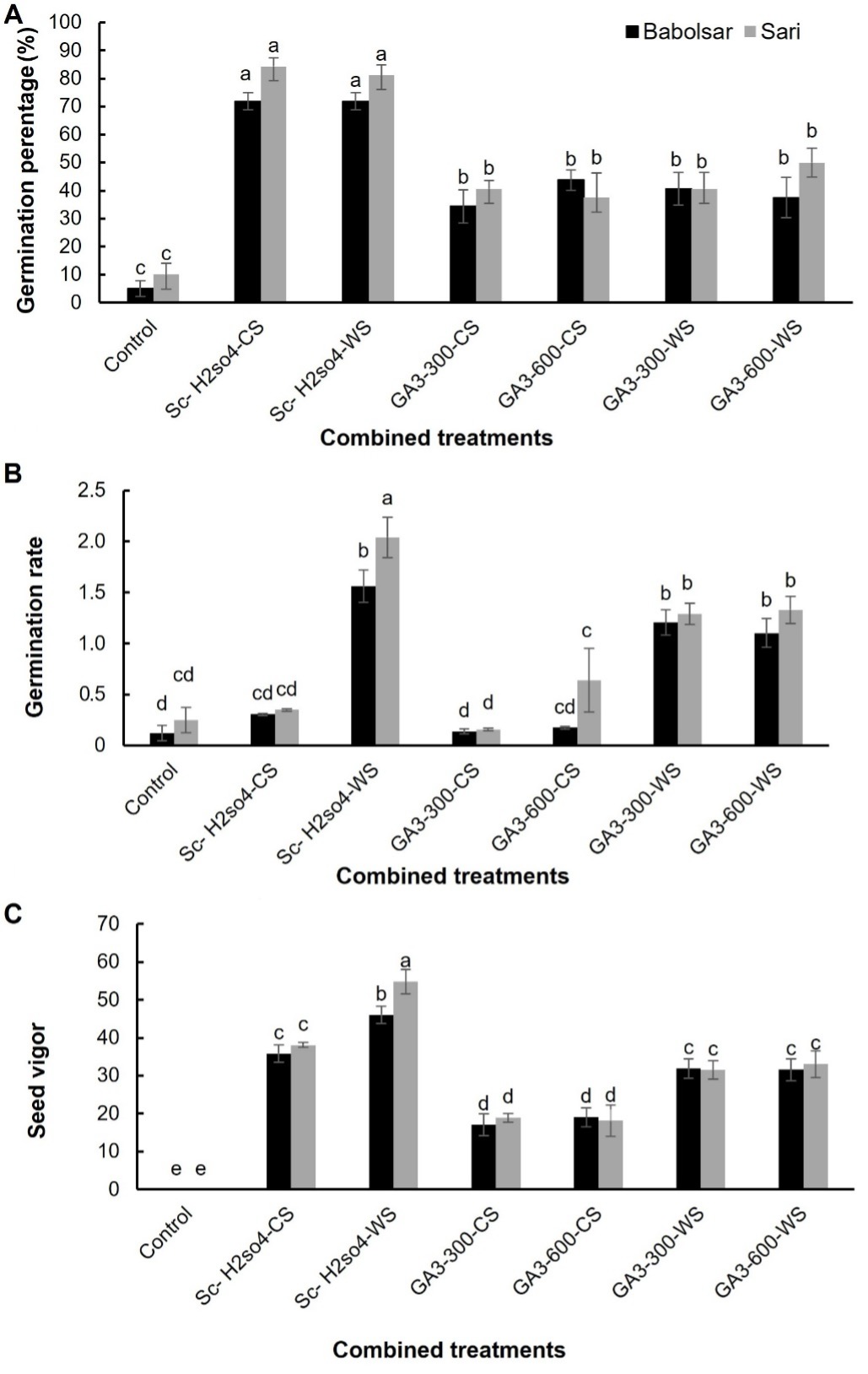
Fig. 5. Effects of combined physical and chemical treatments on seed germination percentage (A), germination rate (B) and seed vigour (C) of Convolvulus persicus in two populations from Mazandaran province of Iran. Data represent the mean ± standard error (n = 4). Mean values with different letters differ significantly at P < 0.05 according to one-way ANOVA with Duncan’s post hoc test. Sc-H2SO4-CS − mechanical scarification in combination with sulphuric acid and cold stratification, Sc-H2SO4-WS − mechanical scarification + sulphuric acid + warm stratification, GA3-300-CS - gibberellic acid (300 mg L-1) + cold stratification, GA3-600-CS - GA3 (600 mg L-1) + cold stratification, GA3-300-WS - GA3 (300 mg L-1) + warm stratification, GA3-600-WS - GA3 (600 mg L-1) + warm stratification.
The highest germination rates were observed in seeds treated with mechanical scarification in combination with H2SO4 and warm stratification in the Sari population (2 ± 0.19, P < 0.05). However, seeds under the same combination of treatments in the Babolsar population exhibited a lower germination rate than the Sari population (1.5 ± 0.15, P < 0.05). The seed germination rates under combined treatment of scarification in combination with H2SO4 and cold stratification were not significantly different (P > 0.05) from the control in either population. Germination rate for seeds treated with GA3 (both 300 and 600 mg L-1) under cold stratification also did not significantly differ (P > 0.05) from the corresponding control in either population. On the other hand, in seeds treated with GA3 (both 300 and 600 mg L-1) under warm stratification, the seed germination rate was significantly (P < 0.05) enhanced in both populations (Fig. 5B).
Our experimental findings underscored the loss of vigour in control seeds, leading to their inability to thrive as young seedlings. Control seeds displayed a limited capacity for germination, as evidenced by the emergence of the radicle exceeding 2 mm in length. However, no subsequent stages of seedling development such as radicle elongation, hypocotyl arch emergence, appearance of folded cotyledons, and full emergence of open cotyledons were observed. Consequently, these control seeds did not progress to seedling maturity and displayed a seed vigour value of zero due to stunted radicle and hypocotyl growth. The seeds under primary treatments exhibited a low germination percentage and rate with a considerable portion failing to advance to the stage of mature seedlings. Consequently, we abstained from assessing their germination rate and seed vigour. In contrast, all seeds treated with combined treatments exhibited progressive growth through all stages of seedling development until reaching maturity (Fig. 6). Among the treated seeds, the highest seed vigour was found in the seeds treated with scarification in combination with H2SO4 and warm stratification, especially in the Sari population (54 ± 3.2, P < 0.05) while combined treatment with cold stratification gave significantly lower values (Fig. 5C). Seeds treated with GA3 (both 300 and 600 mg L-1) under cold stratification showed the lowest seed vigour (ranging from 17 to 19) compared to other treatments (P < 0.05, Fig. 5C).
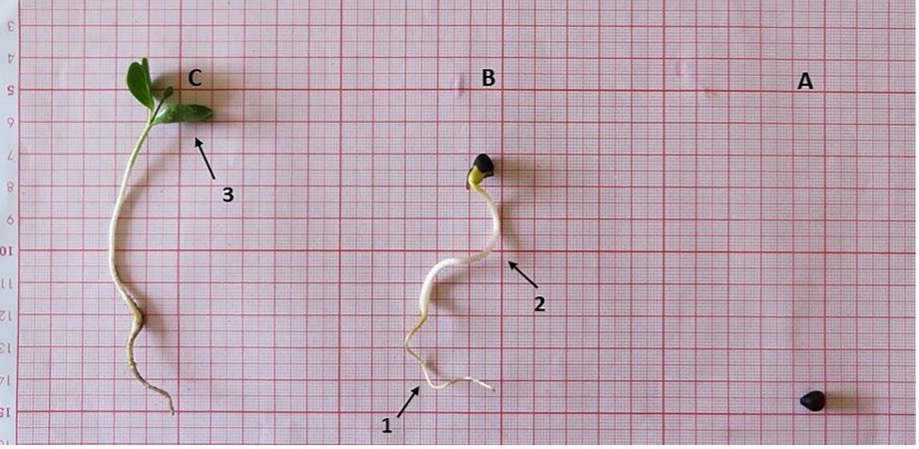
Fig. 6. Seed germination of Convolvulus persicus in the Mazandaran province of Iran: swollen seed (A), germinated seed is developing into mature seedling; 1- radicle and 2- hypocotyl (B) and mature seedling; 3- cotyledon leaves (C).
Discussion
Worldwide, 50-90% of wild plants generate seeds that show some dormancy after maturity. The characteristics of seed dormancy depend on genetics, ecological conditions, growth pattern and geographical distribution (Baskin and Baskin 2014). The interruption of seed dormancy and improvement in germination percentage and rates are influenced by a combination of environmental conditions such as light, temperature, moisture content and hormonal balance. With an understanding the specific requirements of different plant species and implementing appropriate techniques such as scarification, stratification, light exposure, temperature control, and moisture management, seed dormancy can be interrupted and germination rates can be improved (Nautiyal et al. 2023). Our investigation was prompted by the Asheqian et al. (2021) report on poor germination of C. persicus seeds, which motivated us to explore the dormancy status and germination of this endangered and endemic species on the northern coasts of Iran. For reporting of dormancy type and dormancy release treatments, some information should be provided, such as seed qualities, imbibition testing and germination status (Kildisheva et al. 2020).
Our study of C. persicus seed characteristics showed that the seeds collected from Sari were greater in size and weight than the seeds collected from Babolsar. Our previous investigation into C. persicus revealed that the species exhibits more vegetative and reproductive growth in the Sari site than in Babolsar (Asheqian et al. 2021). This disparity can be attributed to the climatic factors present in Sari, such as higher temperatures and increased sunshine hours as shown in Tab. 1. Consequently, the larger seeds observed in Sari may be directly correlated to the prevailing climatic conditions in this particular location. The viability of Sari and Babolsar seeds did not significantly different and both of them were above 75%. In general, seed characteristics is ascertained by genetic potential and environmental factors, especially the nutrition of the mother plant throughout seed formation. It is known that, the seed size and viability have a meaningful impact on germination, seedling growth and establishment (Li et al. 2017). The size of a seed plays a vital role in the early phases of seedling growth, as larger seeds provide more nutrients for development. Additionally, the speed at which seeds germinate may be influenced by their size, with larger seeds typically holding more water. Also, the vitality of a seed is crucial for successful germination and the creation of normal seedlings. A strong germination rate is essential for the successful establishment and seedlings growth, impacting their capacity to contend and thrive in their environment (Tumpa et al. 2021). According to the viability test in our investigation, the regeneration of C. persicus does not seem to be limited by seed viability.
The next critical step in identifying seed dormancy is to assess seed germination across varying temperature conditions (Baskin and Baskin 1998). Seeds are typically considered nondormant if they exhibit a high germination percentage (over 75%). On the contrary, if seeds have low or no germination at diverse temperature levels, they are probably dormant (Erickson et al. 2016). Our investigation revealed that the germination percentage of C. persicus seed under alternating temperatures in both populations was notably low, not surpassing 10%. This observation strongly suggests the presence of dormancy in C. persicus seeds. To further explore this dormancy, we conducted imbibition testing to assess the water permeability of the seed coat. Seeds with impermeable coats are often physically dormant (Silveira 2013). The imbibition essay displayed that the percentage and speed of water absorption in the scarified seeds in both Sari and Babolsar populations were higher than in non-scarified seeds. This finding confirms that the C. persicus seed coat is impermeable. Several studies have reported a water-impermeable coat in Convolvulaceae that causes physical seed dormancy (Jayasuriya et al. 2008b, Baskin and Baskin 2014, Kildisheva et al. 2020). Thus, our findings are aligned with existing literature and underscore the importance of understanding seed coat properties in dormancy mechanisms.
After mechanical or chemical scarification, non-deep physically dormant seeds will immediately and extensively germinate (Nautiyal et al. 2023). However, our results indicate that seed dormancy and germination were not affected efficiently either by mechanical scarification treatments for 3 minutes, nor by chemical scarification using H2SO4 for 5 and 15 minutes. Although, scarification for 5 minutes demonstrated some efficacy in enhancing germination percentage, the observed increase did not surpass 30%. This suggests that there is still a problem for seed germination. When scarified seeds absorb water adequately, but fail to show remarkable enhancement in germination percentage, the presence of physiological dormancy is suggested (Kildisheva et al. 2018a). Interestingly, the application of gibberellic acid treatment in the absence of scarification, at 300 and 600 mg L-1, did not result in any significant outcome on seed dormancy alleviation or enhancement of germination percentage in C. persicus seeds. In contrast, both cold and warm stratification for 4 and 8 weeks notably increased germination percentage in both the Babolsar and the Sari populations. But the germination ability of seeds in warm stratification conditions was higher than that in cold stratification conditions after 4 and 8 weeks. This shows that cold and warm stratification treatments can release physical dormancy. Stratification enhances the embryo's growth capacity and then enables it to surpass the physical barrier to its development (Chen et al. 2022).
Building upon the progress made in overcoming the dormancy of C. persicus seeds and enhancing germination through cold and warm stratification methods, we decided to explore combined treatments with cold and warm stratification to further enhance the germination percentage. Previous studies have highlighted the efficacy of combined treatments in facilitating seed germination in various species. For instance, the combination of scarification and stratification treatments notably enhanced the germination of Rubus seeds. Especially, H2SO4 scarification with cold stratification was impressive for Rubus parviflorus Weston, Rubus phoenicolasius Maximand and Rubus takesimensis Nakai (Choi et al. 2016). Similarly, scarification with H2SO4 followed by warm stratification for 4-weeks was found to be highly effective in improving seed germination percentage for Rosa multibracteata Hemsl & E.H.Wilson, Rosa hugonis Hemsl. and Rosa filipes Rehder & E.H.Wilson (Zhou and Bao 2011). Our study demonstrates that utilizing a combination of mechanical scarification, H2SO4 and either cold or warm stratification considerably enhances the seed germination percentage of C. persicus. Although no statistically meaningful difference was found in the germination percentage between C. persicus seeds subjected to the combined treatment of mechanical scarification + H2SO4 and either cold or warm stratification, in both populations the results of germination rate and vigour index suggest that the combined treatment of mechanical scarification + H2SO4 and warm stratification may be more favourable to the breaking of seed dormancy and enhancing potential seedling survival than cold stratification. Mechanical scarification and H2SO4 treatment can simulate natural processes that enhance seed germination in situ. Factors such as waves, tidal movements, the burial and exhumation of seeds by shifting sands particularly in dune systems and biotic interactions such as ingestion by waterbirds can mimic the effects of natural abrasion on seed coats. The application of H2SO4 mimics natural processes such as microbial degradation or physical abrasion that occur in the seed's natural habitat, which can lead to the breaking of dormancy. Warm stratification can mimic natural warming of soils in spring, aligning with environmental cues for dormancy break and germination. The success of warm stratification over cold stratification may also reflect an adaptation to the specific climate and soil conditions of the area where the seeds originate (Baskin et al. 2002). Seeds from warmer climates or those that experience significant soil temperature fluctuations such as coastal ecosystems may be more responsive to warm stratification treatments.
Gibberellic acid is a highly effective hormone that stimulates germination and has been proposed as a solution for the problem of seed dormancy in various species. By activating the amylase enzyme, gibberellic acid facilitates the transfer of essential materials from storage sites to growth sites, ultimately enabling dormant seeds to germinate. Conversely, without gibberellin, the breakdown and transfer of materials do not occur, resulting in germination failure (Bewley and Black 1994). The effectiveness of gibberellic acid as a growth promoter is further enhanced when combined with cold or warm stratification treatments. Exogenous GA3 application and cold stratification have proven to be particularly effective methods for releasing of physiological dormancy (Baskin and Baskin 2007). Recent research by Chen et al. (2022) demonstrated that the combined treatment of gibberellic acid and warm stratification successfully broke dormancy in Cinnamomum migao H. W. Li seeds and significantly increased their germination rate. Conforming to these findings, our own results also highlight the role of combining gibberellic acid treatment with both cold and warm stratification for overcoming dormancy and improving germination in C. persicus seeds. However, it is worth noting that compared to the combined treatments of mechanical scarification and H2SO4 during cold and warm stratification, the effect of the combined treatments of gibberellic acid and either cold or warm stratification was relatively low in terms of removing dormancy and increasing the seed germination percentage of C. persicus. Our findings provide evidence that dormancy in C. persicus seeds can be a combination of physical and physiological types, but the final answer to this issue requires more detailed research. To ensure successful germination, it is crucial to address both types of dormancies. Additionally, when working with rare plants, the quantity of available seeds is often limited. As a result, researchers typically rely on a single germination treatment (Crawford et al. 2007). Therefore, it becomes necessary to provide the most effective protocol for maximizing germination percentage. By doing so, seed banks can considerably enhance their efficiency and play a more significant role in conserving biodiversity (Godefroid et al. 2009).
Conclusion
Convolvulus persicus seeds exhibit a kind of combination of physical and physiological dormancy. Through comprehensive investigation, we have identified a highly effective method for overcoming dormancy in C. persicus seeds: a combined treatment involving mechanical scarification, H2SO4 application, and warm stratification. This particular treatment significantly enhances both germination percentage and rate, along with seed vigour. Importantly, our findings indicate that warm stratification serves as more favourable approach for breaking seed dormancy and promoting C. persicus seedling survival compared to cold stratification. Furthermore, while occasional variations in seed traits were observed between the Sari and Babolsar populations, the overall impact of population type on germination capacity was deemed insignificant. This underscores the robustness and adaptability of C. persicus seeds across different geographical locations. Overall, our study provides valuable insights into the dormancy mechanisms of C. persicus seeds and offers practical recommendations for enhancing germination and seedling survival in this species.