Introduction
The oral absorption of a drug is affected by various physiological factors in the gastrointestinal tract [1]. The physiological conditions in the fed state are markedly different from those in the fasted state. For example, bile micelle concentration is significantly increased in the fed state. In addition, food components can interact with drug molecules. It is still challenging to predict the food effects on oral drug absorption from in vitro data [2-4]. In the mechanistic oral absorption models, the free (unbound) fraction in the intestinal fluid (fu) is one of the key parameters that determine the effective intestinal permeability of a drug (Peff) [5]. High solubility/ low permeability drugs tend to show a negative food effect. In these cases, the rate and extent of fraction dose absorbed Fa is limited by epithelial membrane permeation (Fa rate-limiting step (FaRLS): permeability-limited (PL) by the epithelial membrane (PL-E)) [2,6]. Recently, the bile micelle and food binding of drugs were reported to be able to elucidate the negative food effect for hydrophilic tertiary and quaternary amines [5,7]. Some zwitterionic antihistamine drugs are also known to show negative food effects in humans. However, it has not been clear whether a zwitterionic drug can also bind to bile micelles and/or food components.
The purpose of the present study was to investigate whether zwitterionic antihistamine drugs can bind to bile micelles and food components. Bilastine (BIL), cetirizine (CET), fexofenadine (FEX), and olopatadine (OLO) were employed as model drugs (Figure 1). The physicochemical properties of these drugs are shown inTable 1. These drugs are zwitterionic and show moderate lipophilicity at pH 6.5. The fed/fasted AUC ratios of BIL, CET, FEX, and OLO are 0.60 to 0.7 [8-10], 0.92 [11], 0.76 to 0.85 [12,13], and 0.84 [14], respectively. In this study, dynamic dialysis was used to measure the fu values in the simulated intestinal fluids consisting of bile micelles and food homogenates.
Drug | MW | pKa a | Isoelectric point | log Doct pH 6.5b | Dose, mg | Food effect, %c |
---|---|---|---|---|---|---|
Bilastine | 464 | 4.0 (A) 8.8 (B) | 6.40 | 0.39 ± 0.01 | 20 | 0.60 – 0.70 |
Cetirizine | 389 | 2.12 (B) 2.90 (A) 7.98 (B) | 5.44 | 1.46 ± 0.01 | 10 | 0.92 |
Fexofenadine | 502 | 4.20 (A) 7.84 (B) | 6.02 | 0.51 ± 0.01 | 120 | 0.76 – 0.85 |
Olopatadine | 337 | 4.18 (A) 9.79 (B) | 6.99 | 0.34 ± 0.01 | 10 | 0.84 |
The permeation flux (J) is expressed by the total drug concentration dissolved in the GI fluid (Cdissolv = unbound + bound) and the effective (apparent) permeation coefficient (Peff) as inEquation (1) [2]:
In the case of PL-E, according to the free fraction theory, only unbound molecules can permeate the epithelial membrane,Equation (2),
where Pep is the epithelial membrane permeation coefficient defined based on the unbound drug concentration, and fu is the unbound (free) fraction. Bile micelle and food binding can reduce the concentration of unbound drug molecules (= fu Cdissolv) at the epithelial membrane surface. In the case when the oral absorption of a drug is permeability-limited, Fa can be calculated as inEquation (3)
where A = 1.4 × 104 s/cm (Peff in cm/s). The Fa ratio (= AUC ratio) in the fasted/fed states approximately becomes the fu ratio (fu,fed/fu,fasted) for Fa < 0.7 cases [2].
Experimental
Material
Fexofenadine hydrochloride, bilastine, olopatadine hydrochloride, and cetirizine dihydrochloride were purchased from Tokyo Chemical Industry Co., Ltd (Tokyo, Japan). Sodium chloride (NaCl), sodium dihydrogen phosphate dihydrate (NaH2PO4 2H2O), 8N NaOH, 1-octanol, taurocholic acid (TC), and oleic acid (OA) were purchased from FUJIFILM Wako Pure Chemical Corporation (Osaka, Japan). Egg yolk lecithin (EL) was purchased from Kewpie Corporation (Tokyo, Japan). Glyceryl mono-oleate (GM) was purchased from Nippon Surfactant Industries Co., Ltd (Tokyo, Japan). A cellulose dialysis membrane (Φ 15.9×25 mm×15 m) was purchased from As-One Corporation (Osaka, Japan).
The FDA breakfast ingredients, and other food were purchased from the local market (bacon (Itoham Foods Inc, Japan), toast (Choujuku bread, Pasco Shikishima Corporation, Japan), egg (Akadama, miwa keien Co., Ltd, Japan), hash browns (Hoshino potato, Heinz Japan Ltd, Japan), whole milk (Oishii Megumilk Snow Brand Milk, MEGMILK SNOW BRAND Co., Ltd, Japan), butter (Hokkaido-butter, MEGMILK SNOW BRAND Co., Ltd, Japan).
Methods
Processing of FDA Breakfast
The FDA breakfast was comprised of one strip of bacon, half a slice of toast, one fried egg, 55 g of hash browns, 30 g of butter, and 100 mL of whole milk. After cooking, the FDA breakfast was homogenized for 15 s by a food processor. The FDA breakfast homogenate (BFH) was divided into small aliquots and stored in a freezer (-30 °C). It was thawed under running water before use.
Measurement of the unbound fraction by dynamic dialysis
Dynamic dialysis was performed using a side-by-side chamber (SANPLATEC Co., Ltd (Osaka, Japan)) with a cellulose dialysis membrane (molecular weight cut-off: 3500). The membrane area was 2.0 cm2. The fluid volume was 1.5 mL for both the donor and acceptor sides. The fasted and fed state simulated intestinal fluids (FaSSIF and FeSSIF, respectively) with or without BFH were used as test media (Table 2). Each drug was dissolved in the test media and added to the donor side (0.04 mM (BIL), 0.2 mM (CTZ), 0.5 mM (FEX), and 0.5 mM (OLO)). The blank FaSSIF was added to the acceptor side. After incubation for 1 h at 37 °C, the drug concentration in the acceptor side was measured by HPLC (Shimazu Prominence LC-20 series and Agilent Technologies 1200 Series, column: ZORBAX Eclipse Plus (C18 2.1×50 mm, 3.5 μm) (Agilent Technologies), flow rate: 0.6 mL min-1, mobile phase: 0.1 % trifluoroacetic acid-acetonitrile/0.1 % trifluoroacetic acid-water (25 % (BIL), 35 % (CTZ), 35 % (FEX), and 28 % (OLO)), detection: UV (BIL: 280 nm, CET: 230 nm, FEX: 225 nm, and OLO: 300 nm), column temperature: 40 °C, and injection volume of 10 μL).
Test medium | Content, mM | BFH, %c | |||
---|---|---|---|---|---|
TC | EL | GM | OA | ||
FaSSIF | 3 | 0.75 | 0 | 0 | 0 |
FeSSIF | 15 | 3.75 | 0 | 0 | 0 |
×2FeSSIF | 30 | 7.50 | 0 | 0 | 0 |
FeSSIFv2 | 10 | 2.0 | 5 | 0.8 | 0 |
BFH b | 0 | 0 | 0 | 0 | 50 |
FeSSIF+BFH | 15 | 3.75 | 0 | 0 | 50 |
Permeation was calculated as the ratio of the concentration in the acceptor side at 1 h and the theoretical equilibrium concentration (0.02 mM (BIL), 0.1 mM (CTZ), 0.25 mM (FEX), and 0.25 mM (OLO)) (×100 to convert to %). The unbound fraction (fu) was calculated as the ratio of permeation in each medium and the test medium of Blank FaSSIF.
The octanol-water distribution coefficient measurement
The octanol-water distribution coefficient (log Doct) values of model drugs were measured by the shake-flask method. A model drug was dissolved in blank FaSSIF pre-saturated with 1-octanol (BIL: 0.1 mM, CTZ: 1 mM, FEX: 1 mM, and OLO: 1 mM). The drug solution (2.5 mL or 5.5 mL) was added to 0.5 mL of 1-octanol pre-saturated with water. The samples were shaken by a shaker for 90 minutes at room temperature (25 ± 2 °C). The drug concentration in the aqueous phase was measured by a UV absorbance (BIL: 280 nm, CET: 230 nm, FEX: 225 nm, and OLO: 300 nm, UV-1850, Shimadzu Corporation, Kyoto, Japan). The log Doct value was calculated byEquation 4:
where Caq,ini and Caq are the initial and equilibrium drug concentrations in the aqueous phase, and Vaq and Voct are the volume of aqueous and octanol phases, respectively.
Results and discussion
The fu values of all drugs decreased in the presence of bile micelles and BFH (Figure 2,Table 3). This result suggests that the reduction of fu by bile micelle binding in the fed state is likely to be one of the reasons for the negative food effect on the oral absorption of these drugs. The addition of BFH also decreased the fu values of model drugs. Therefore, direct food binding could also be one of the reasons for the negative food effect.
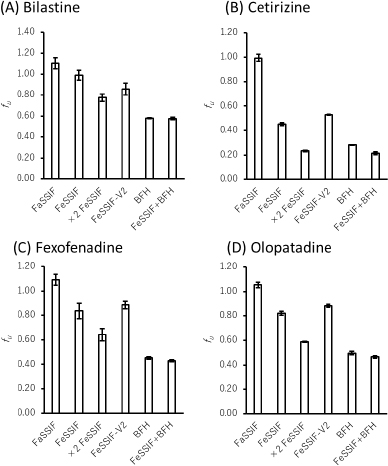
Drug | Test medium | Permeation at 1 h, % | fu | fu ratio b |
---|---|---|---|---|
Bilastine | Blank FaSSIF | 15.6 ± 0.5 | ||
FaSSIF | 17.3 ± 0.8 | 1.10 ± 0.05 | ||
FeSSIF | 15.5 ± 0.7 | 0.99 ± 0.05 | 0.90 ± 0.04 | |
×2 FeSSIF | 12.1 ± 0.6 | 0.78 ± 0.04 | 0.70 ± 0.03 | |
FeSSIF-V2 | 13.4 ± 0.8 | 0.86 ± 0.06 | 0.78 ± 0.05 | |
BFH | 9.0 ± 0.1 | 0.58 ± 0.00 | 0.52 ± 0.00 | |
FeSSIF+BFH | 9.0 ± 0.1 | 0.58 ± 0.01 | 0.52 ± 0.01 | |
Cetirizine | Blank FaSSIF | 16.4 ± 0.6 | ||
FaSSIF | 16.3 ± 0.5 | 0.99 ± 0.03 | ||
FeSSIF | 7.4 ± 0.2 | 0.45 ± 0.01 | 0.46 ± 0.01 | |
×2 FeSSIF | 3.8 ± 0.1 | 0.23 ± 0.01 | 0.23 ± 0.01 | |
FeSSIF-V2 | 8.7 ± 0.1 | 0.53 ± 0.00 | 0.53 ± 0.00 | |
BFH | 4.6 ± 0.0 | 0.28 ± 0.00 | 0.29 ± 0.00 | |
FeSSIF+BFH | 3.5 ± 0.2 | 0.21 ± 0.01 | 0.22 ± 0.01 | |
Fexofenadine | Blank FaSSIF | 12.0 ± 0.7 | ||
FaSSIF | 13.1 ± 0.5 | 1.09 ± 0.04 | ||
FeSSIF | 10.0 ± 0.7 | 0.84 ± 0.06 | 0.76 ± 0.06 | |
×2 FeSSIF | 7.7 ± 0.6 | 0.64 ± 0.05 | 0.59 ± 0.05 | |
FeSSIF-V2 | 10.6 ± 0.4 | 0.88 ± 0.03 | 0.81 ± 0.03 | |
BFH | 5.4 ± 0.1 | 0.45 ± 0.01 | 0.41 ± 0.01 | |
FeSSIF+BFH | 5.1 ± 0.1 | 0.43 ± 0.01 | 0.39 ± 0.01 | |
Olopatadine | Blank FaSSIF | 20.8 ± 0.7 | ||
FaSSIF | 21.9 ± 0.5 | 1.05 ± 0.02 | ||
FeSSIF | 17.1 ± 0.3 | 0.82 ± 0.02 | 0.78 ± 0.02 | |
×2 FeSSIF | 12.2 ± 0.1 | 0.59 ± 0.01 | 0.56 ± 0.01 | |
FeSSIF-V2 | 18.4 ± 0.3 | 0.88 ± 0.01 | 0.84 ± 0.01 | |
BFH | 10.3 ± 0.3 | 0.50 ± 0.02 | 0.47 ± 0.01 | |
FeSSIF+BFH | 9.7 ± 0.2 | 0.46 ± 0.01 | 0.44 ± 0.01 |
The fu ratios of FeSSIF/FaSSIF of FEX and OLO were in good agreement with the extent of the food effect in humans. However, the food effect was underestimated for BIL and overestimated for CET. In the presence of BFH, the fu ratio of FeSSIF/FaSSIF of BIL was in good agreement with the food effect. Even though the fu ratio of CET is lower than that of the other drugs, the clinical negative food effect on the oral absorption of CET is less significant. This would be due to the higher lipophilicity and faster passive permeability of CET. In such case, the extent of Fa becomes less sensitive to a decrease in fu and Peff (Eq. 3). The bioavailability in humans of CET is > 70 % [11], which is higher than that of FEX (33 %) [16] and BIL (61 %) [8].
The charge species distribution of the model drugs is shown inFigure 3. Despite being zwitterion at pH 6.5, the antihistamine drugs were bound to bile micelles. The bile micelle binding of CET was stronger than that of others, as expected from its higher log Doct (CET > FEX ≈ BIL ≈ OLO). CET is a triprotic compound [17,18]. However, the first pKa (N1 of the piperidine group) is 2.12 and is not protonated at pH 6.5. Therefore, CET mainly exists as a zwitterion at pH 6.5.
The log Doct values of BIL (0.39) and OLO (0.34) are close to that of propranolol (PRO) (log Doct = 0.4) [5]. In addition, the amine groups of these drugs are > 99 % charged at pH 6.5. However, the bile micelle binding of propranolol is reported to be about 3-fold stronger than those of BIL and OLO. In addition, even though the log Doct values of the zwitterions are greater than that of quaternary ammonium compounds (QAC) (log Doct ≪ 0), the fu values of the zwitterionic drugs are comparable with that of QACs [7]. The bile micelles in FaSSIF and FeSSIF are negatively charged by the -SO3- group in taurocholic acid. Therefore, the carboxyl anion (-COO-) in the zwitterionic drugs was suggested to reduce the bile micelle binding, like in the cases of liposome binding [18,19]. In a buffer solution at the isoelectric pH point, a zwitterion drug exists as an equilibrium between a zwitterion molecule and an un-ionized molecule [20]. The isoelectric pH points of the model drugs are near pH 6.5 (5.4 to 7.0) (Table 1). From the difference between the pKa values of carboxylic acid and amine (> 3.6), they are estimated to exist as the zwitterion more than 99.8 % [21]. Therefore, the zwitterionic molecules may bind bile micelles. A more detailed mechanism of the charge effect on the bile micelle binding of drugs is under investigation.
Conclusions
In conclusion, the zwitterionic antihistamine drugs were found to bind to bile micelles and foods, suggesting that it would be the reason for the negative food effect. The lipophilicity and charge state were suggested to affect the bile micelle binding. Dynamic dialysis would be a good tool to measure bile micelle and food binding.