Introduction
Abiotic stressors, which include temperature extremes, water availability, salinity, heavy metals, and UV radiation, can have detrimental effects on plants and result in significant crop yield losses (Saharan et al. 2022). Heavy metals such as arsenic, cadmium, lead, and mercury are toxic to plants and animals even at low concentrations and can accumulate in the environment from human activities such as mining, industrial discharge, and use of pesticides and fertilizers (Alengebawy et al. 2021, Singhal et al. 2023). Cadmium (Cd) is a nonessential, heavy metal element and a widespread environmental contaminant that is persistent, non-biodegradable, bio-accumulative and highly toxic even at low concentrations (Hussain et al. 2019, Mahawar and Shekhawat 2023). Cd toxicity, can affect many physiological and morphological processes in plants, including plant growth, respiration, water and nutrient uptake, and root growth (Chen et al. 2011, Liu et al. 2012, Sheirdil et al. 2012). Cd causes adverse effects on germination, seedling vigour index, and plant growth (Sheirdil et al. 2012, He et al. 2014). Plants exposed to Cd have damaged photosynthetic apparatus and inhibited chlorophyll biosynthesis, which negatively impacts growth and biomass, leaf chlorophyll fluorescence, and photosynthetic parameters. (Ci et al. 2010, Xue et al. 2014, Yang et al. 2015, Mahawar et al. 2021). Cd contamination in agricultural soils limits crop production, which is a major concern given the increasing demand for food worldwide.
Soybean ( Glycine max (L.) Merr.) is the most significant legume crop in the world, as 20% of the seed is oil and 40% is high-quality protein (Ferguson and Gresshoff 2009). It plays a significant role in the global oilseed agriculture landscape because of its high productivity, profitability, and essential role in preserving soil fertility. The increase in Cd concentration significantly inhibited seed germination, root and shoot growth in soybean plants (Sheirdil et al. 2012). Therefore, it is crucial to explore the approaches to alleviating heavy metal toxicity in soybean. Seed priming has proven to be an effective strategy for increasing seed vigour and germination, as well as seedling growth and field establishment in unfavourable conditions of heavy metal toxicity (Prajapati et al. 2020). It appears that seed priming is a helpful method for reducing cadmium toxicity in plants (Singhal et al. 2023). In the field of seed technology, physical techniques have proven to be much superior to conventional protocols of osmo, chemical, hydro and hormonal priming (Bilalis et al. 2012). One of the most researched physical pre-sowing seed treatments in agriculture is based on the use of magnetic field (MF) for the alleviation of heavy metal toxicity (cadmium, arsenic and mercury) in plants (Chen et al. 2011, Fatima et al. 2020, Prajapati et al. 2023).
Magnetopriming (MP) has a significant effect on a number of levels, including morpho-structural elements (seed germination, plant growth, and yield) and modifications in the expression of genes linked to nitrogen metabolism and seed germination in non-stress and abiotic stress conditions (Kataria et al. 2020, Sarraf et al. 2020, Raipuria et al. 2021). According to some theories, MF could attract iron particles, growth hormones, and carbohydrates (Xue et al. 2014). It may also have an impact on transcription-related factors and gene expression processes (Stutte et al. 2006, Kataria et al. 2020). Additionally, it might immediately trigger the production of calcium ions (Ca2+) in seed embryos (Kataria et al. 2023). The germination of seeds and the physiological growth of tomato plants are significantly impacted by neodymium earth magnets by the stimulation of seed germination and speed of germination by 50% and production of larger leaf areas and more root hairs in the growing plantlets (Abhary and Akhkha 2023). It was earlier reported that MP mitigates the adverse effect of cadmium stress in mung beans by reducing the level of malondialdehyde, nitric oxide, hydrogen peroxide and superoxide radical content and increased the growth and photosynthetic performance in mung bean plants (Chen et al. 2011). However, the mitigating effect of MP during seed germination has not been reported for heavy metal toxicity especially for cadmium in soybean seedlings. Hence the objective of the present study is to examine the impact of MP on seed germination, seedling growth and biochemical parameters in soybean under cadmium toxicity and to reveal if MP could enhance tolerance of soybean towards Cd toxicity during early seedling stage.
Materials and methods
Plant material
Breeder seeds of soybean ( Glycine max [L.]) var. JS-9560 (commonly grown variety of soybean in the Malwa region, Indore, Madhya Pradesh, India), were obtained from the ICAR-Indian Institute of Soybean Research, Khandwa Road, Indore, M.P., India.
Magnetic field treatment
To pre-treat the seeds with a static magnetic field (SMF), we used an electromagnet (AETec), fabricated by the Academy of Embedded Technology in Delhi, India. The pole components measured 16 cm in length and 9 cm in diameter while the coil consisted of 3000 turns and a resistance of 16 Ohms. Nearly 100 dry seeds of soybean at a time were placed in a cylindrical sample holder made of thin transparent plastic between the two poles of the electromagnet for magnetopriming, while maintaining the temperature at 25 ± 1 °C. The distance between the two poles was 5cm. The SMF was developed using a direct current (DC) power supply (80 V/10 A) with a continuously changing output. Based on previous studies, soybean seeds were treated with 200 mT of SMF for an hour (Kataria et al. 2020). The SMF strength was obtained by adjusting the current and voltage by power supply and the obtained SMF strength was measured using a digital gauss meter (AETech model DGM-102) with a probe consisting of an indium arsenide crystal encapsulated by a 5×4×1 mm non-magnetic sheet. The local geomagnetic field was less than 10 mT in the north to south direction. With the exception of the SMF treatment (200 mT for 1 hour) for primed seeds, all other conditions were the same for unprimed and magnetoprimed seeds. Every experiment was carried out concurrently with unprimed seeds (which were used as controls) stored at room temperature (25 ± 1 °C) and kept away from the magnetic field (less than 5 mT).
Treatment to induce cadmium toxicity
To conduct the germination experiment, both magnetoprimed and unprimed soybean seeds were first surface-sterilized with 0.01% HgCl2 for 2 minutes, followed by washing three times with distilled water. The fifteen seeds were then allowed to germinate on wet Whatman filter paper in each Petri plate (15 cm diameter) in three replications with 10 mL of different concentrations of CdCl2 (25, 50, 100, 200, and 300 μM) while 10 mL of distilled water was used as a control (0 μM). The seeds were kept for germination in darkness at 25 ± 1 °C for 5 days in the incubator. Germination percentage was calculated based on the number of normal seedlings, as the ratio of the number of germinated seeds to the total number of seeds on the third day of imbibition in different concentrations of CdCl2.
Early growth characteristics of seedlings
To estimate the effect of magnetopriming on soybean seedling growth, ten seedlings were randomly selected from each treatment (magnetoprimed and unprimed seeds germinated under different concentrations of CdCl2) in biological triplicates (n = 3). The length of shoot and root, along with the length of the whole seedling, were measured for each selected seedling. Fresh weight was also measured. For dry weight determination, the seedlings were dried in an oven at 80 °C for 72 h and weighed. The vigour index was calculated using the formulae proposed by Abdul-Baki and Anderson (1973):
Vigour index I = germination % × seedling length (root + shoot) cm
Vigour index II = germination % × seedling dry weight (root + shoot) g
Vigour index I is the multiple of the percentage of germination and seedling length, and Vigour index II is the multiple of percentage of germination and seedling dry weight.
Enzyme activities during germination
Total amylase and protease activities were measured in the soybean seedlings derived from unprimed and magnetoprimed seeds after 5 days (120 h) of imbibition under different CdCl2 concentrations. After 5 days of imbibition, the fresh seedlings with root, shoot and cotyledons were taken for analysis of enzymes and biochemical parameters. Each experiment was repeated in three biological replicates with three technical replicates.
The assay of total amylase activity was performed using the method of Sawhney et al. (1970). The seedling homogenate (100 mg) was mixed with 5 mL of 80% chilled acetone and centrifuged at 15 000 rpm for 10 min at 4 °C. The resulting pellet was dissolved in 10 mL of 0.2 M phosphate buffer (pH 6.4) and centrifuged again at 15 000 rpm for 20 min at 4 °C. The amylase activity in the obtained supernatant was then determined by the addition of 2 ml of phosphate buffer (pH 6.4), 1 mL of starch (1%), and incubation for 30 min at room temperature. Subsequently, 1 mL of 0.1 M HCl and 0.1 mL of 0.1 M potassium iodide were added to the reaction mixture and the absorbance was measured at 660 nm using a spectrophotometer. The total amylase activity was expressed as mg starch hydrolysed g-1 fresh weight of seedlings h-1.
The assay of protease activity was carried out according the method of Kunitz (1947) modified by Kataria et al. (2023). The 1.0 g seedlings were crushed in 0.2 M phosphate buffer (pH 7.4) and centrifuged at 13 800 rpm at 4 °C for 30 minutes. To 0.5 mL of supernatant, 0.5 mL of casein (1%) prepared in 0.2 M carbonate buffer (pH 9.2) was added and the mixture was incubated for 10 minutes at 37 °C. The reaction was terminated by the addition of 1 mL of 10% trichloroacetic acid (TCA) and centrifugation at 13,800 rpm for 10 minutes at 4 °C. After centrifugation, 2.5 mL of carbonate buffer (0.44 M pH 9.5) was added and the development of a blue colour was observed upon addition of 0.5 mL Folin’s reagent followed by incubation for 30 minutes at room temperature. The protein content was measured at 660 nm against carbonate buffer (0.44 M, pH 9.5) and Folin’s reagent taken as a blank and the protease activity was represented as mg protein hydrolysed g-1 fresh weight of seedlings.
Determination of biochemical parameters
Thiobarbituric acid reactive substances (TBARS) were used to measure the level of malondialdehyde (MDA), according to the method described by Heath and Packer (1968). Soybean seedlings (100 mg) were homogenized in 1 mL of 0.1% (w/v) TCA, and the resulting homogenate was centrifuged at 12 000 rpm for 15 minutes at 4 °C. The supernatant was used for the TBARS assay. Specifically, 0.5 mL of the supernatant was mixed with 1 mL of 0.5% (w/v) 2-thiobarbituric acid (TBA) prepared in 20% TCA and incubated in a boiling water bath for 30 minutes. The reaction was stopped by placing the tube in an ice bath, and the mixture was then centrifuged at 12 000 rpm for 5 minutes. The absorbance of the supernatant was measured at 532 nm, and the value for the non-specific absorbance read at 600 nm was subtracted. TBA (0.5%) in TCA (20%) was taken as blank. The amount of MDA was calculated using an extinction coefficient (ε = 155 mM cm) and expressed as µmol MDA g-1 fresh weight of seedlings.
The proline content was calculated using the method of Bates et al. (1973). Using a mortar and pestle, 500 mg of soybean seedlings were homogenised in 10 mL of 3% (w/v) aqueous sulphosalicylic acid; the homogenate was then centrifuged for 5 minutes at 4°C at 10 000 rpm. The proline was estimated using the supernatant. Two mL each of glacial acetic acid, acid ninhydrin reagent, and supernatant were combined. The mixture was boiled at 100οC for 1 h. The reaction was terminated by cooling in an ice bath and 4 mL of toluene was added. Following thorough mixing, the toluene-containing chromophore was isolated, and the red colour generated absorbance was measured at 520 nm using toluene as the blank. The proline content was calculated through the standard curve prepared in the range of 5-25 μg proline and expressed as μg proline g-1 fresh weight of the seedlings.
The estimation of hydrogen peroxide (H2O2) was carried out by the method described by Mukherjee and Choudhuri (1983), which involves the formation of a titanium-hydroperoxide complex. First, 0.5 g of soybean seedlings were crushed in chilled acetone (5 mL) and filtered using Whatman No.1 filter paper. The filtrate (5 mL) was mixed with titanium reagent (2 mL; containing 5% (w/v) titanium oxide and potassium sulphate digested in concentrated sulphuric acid) and ammonium hydroxide solution (2.5 mL) to precipitate the titanium-hydroperoxide complex. The resulting precipitate was centrifuged at 13,800 rpm for 15 min at 4 °C, and the pellet was resuspended in concentrated sulphuric acid (2 M) and centrifuged again. The absorbance of the supernatant was then measured at 415 nm against sulphuric acid (2 M) using a Shimadzu Spectrophotometer (UV-1800) and the amount of H2O2 was calculated and expressed as μmol g-1 fresh weight.
Tolerance index (TI)
Tolerance index (in terms of root length) of seedlings from unprimed and magnetoprimed seeds grown under different concentrations of CdCl2 was determined with the following formula given by Iqbal and Rahmati (1992):
Tolerance index (T.I.) = mean root length in metal solution / mean root length in control × 100
Statistical analysis
Three replications and fully randomised designs were used to arrange the samples. The mean ± standard error (S.E.) of the three biological replicates (n = 3) was used to show the data. Ten seedlings were taken for each replication in order to measure the many aspects of seedling growth, including length of seedlings, root and shoot, fresh and dry weight of seedling, and vigour indices. The mean of three seedlings in each replica serves as the data for the biochemical analysis. The data were analysed by Student’s t-test and significant differences between non-stressed and Cd-stressed soybean seedlings that emerged from unprimed seeds are marked as following*P < 0.05,**P < 0.01,***P < 0.001. Statistically significant differences between magnetoprimed and unprimed seedlings grown in non-stress as well as Cd-stress conditions are marked as#P < 0.05,##P < 0.01,###P < 0.001.
Results
Effect of MP on seed germination and seedling development under cadmium treatments
Germination percentage decreased with increasing CdCl2 concentration in magnetoprimed and unprimed seeds of soybean. A remarkable decrease of 50% was observed at 100 μM CdCl2 and 72% was observed at 200 and 300 μM CdCl2 in unprimed seeds (Fig. 1a). Magnetopriming of seeds enhanced percentage germination at all the concentrations of CdCl2 used as compared to respective unprimed seeds. Maximum enhancement in percentage germination by MP (41%, 66% and 33%) was obtained at 100, 200 and 300 μM CdCl2, respectively (Fig. 1a).
Root, shoot and seedling lengths were reduced with increasing CdCl2 concentration in magnetoprimed and unprimed seeds of soybean (Figs. 1b, c, d). The decrease in root length was more prominent than the decrease in shoot length. Maximum decreases of 75% and 93% were observed in root length of seedlings from unprimed seeds at 100 and 200 μM CdCl2 and root growth was completely inhibited at 300 μΜ CdCl2 (Fig. 1b). Magnetoprimed soybean seeds demonstrated higher shoot and root length at all the concentrations of CdCl2 used as compared to corresponding controls (Fig. 1b, Fig. 1c). Magnetopriming caused increases of 18, 19 and 24% in shoot length of seedlings at 50, 100 and 200 μM CdCl2, respectively (Fig. 1B). Similarly, significant promotion in root length by 55, 113 and 240% at 50, 100 and 200 μM CdCl2 respectively was obtained in seedlings from magnetoprimed seeds as compared to the seedlings of unprimed seeds (Fig. 1c).
There was substantial decrease in length of seedlings observed at a higher concentration (300 μΜ) of CdCl2 (Fig.1d). The CdCl2 at 100, 200 and 300 μΜ concentrations caused significant inhibition of seedling length by 51%, 73% and 80% respectively. On the other hand, a maximum increase of 31% was observed at 100 μM CdCl2 followed by a 59% increase at 200 μM CdCl2 in magnetoprimed seeds (Fig. 1d).
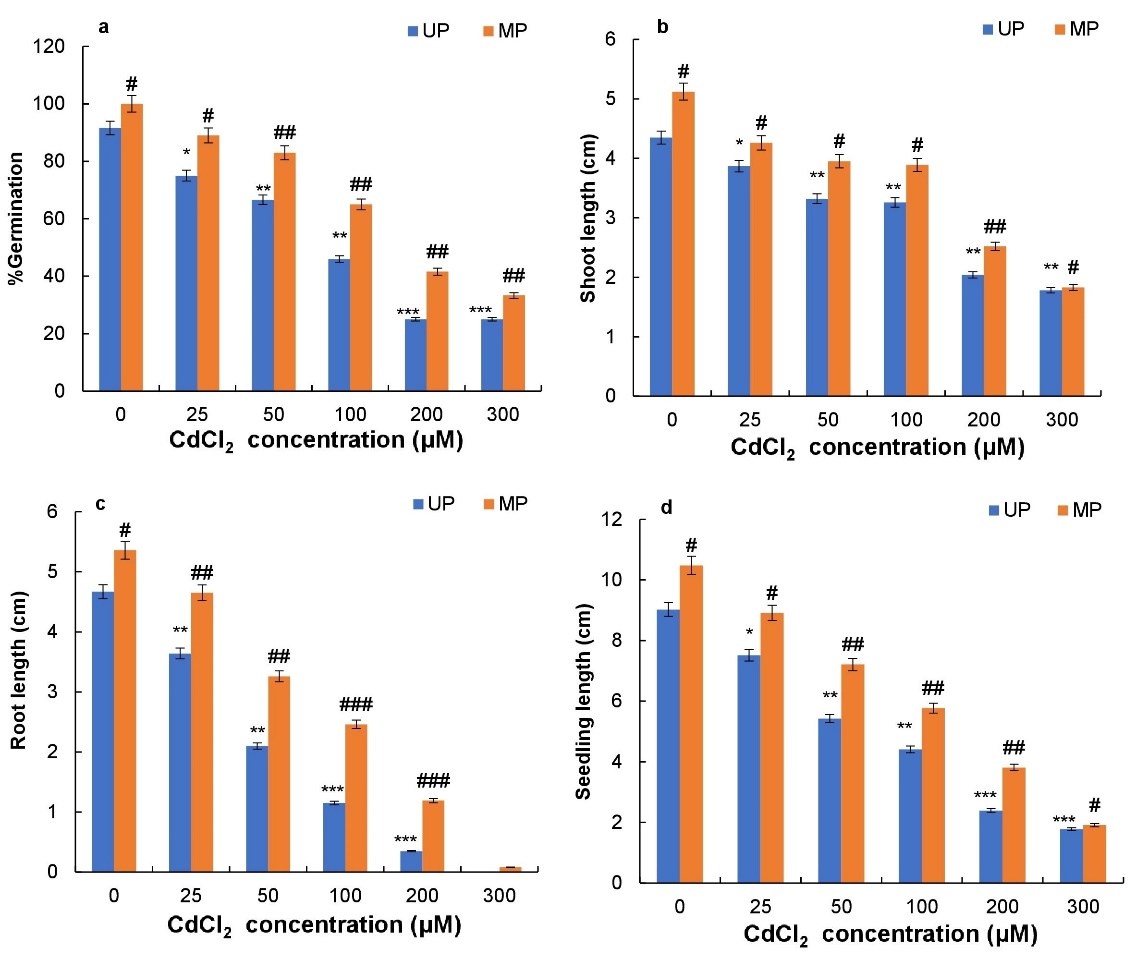
Fig. 1. Effect of magnetopriming on percentage germination (a), shoot length (b), root length (c) and seedling length (d) of soybean seedlings under Cd toxicity. The vertical lines on the bar indicate ± S.E. for mean of triplicates (n = 3). Significant differences between non-stressed and Cd-stressed soybean seedlings that emerged from unprimed seeds are marked as follows:*P < 0.05,**P < 0.01,***P < 0.001. Statistically significant differences between magnetoprimed (MP) and unprimed (UP) seedlings grown in non-stress as well as Cd-stress conditions are marked as#P < 0.05,##P < 0.01;###P < 0.001.
Effect of MP on fresh weight and dry weight of soybean seedling under cadmium treatments
Fresh and dry weight of unprimed and primed seeds gradually decreased with increasing CdCl2 concentration (Figs. 2a, b). CdCl2 treatments caused reduction in fresh weight by 26%, 35% and 44% respectively at 100, 200 and 300 μΜ CdCl2 concentration as compared to the controls (Fig. 2a). Further, there was significant increase in fresh and dry weight of seedling observed in primed seeds as compared to unprimed ones at all the concentrations of Cd used. Maximum of 24, 37, 42 and 21% promotion in fresh weight of seedlings was found in magnetoprimed as compared to unprimed seed respectively at 50, 100, 200 and 300 μΜ CdCl2 (Fig. 2a). Similar results were observed for dry weight of seedlings (Fig. 2b).
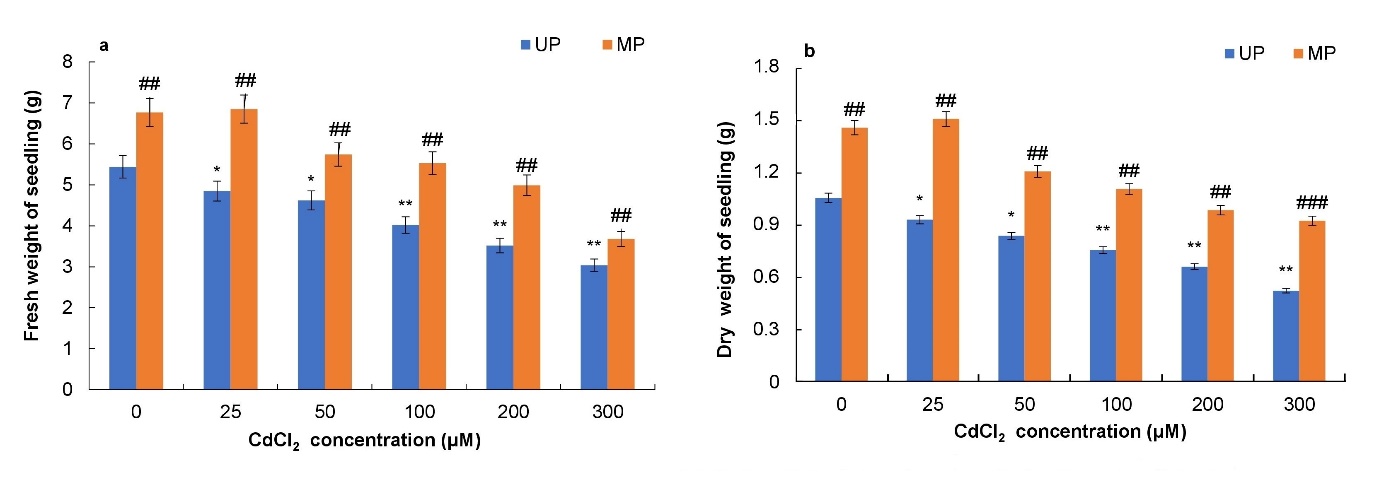
Fig. 2. Effect of magnetopriming on fresh weight (a) and dry weight (b) of soybean seedlings under Cd toxicity. The vertical lines on the bar indicate ± S.E. for mean of triplicates (n = 3). Significant differences between non-stressed and Cd-stressed soybean seedlings that emerged from unprimed seeds are marked as follows:*P < 0.05,**P < 0.01. Statistically significant differences between magnetoprimed (MP) and unprimed (UP) seedlings grown in non-stress as well as Cd-stress conditions are marked as##P < 0.01.
Effect of MP on vigour indices of soybean seedling under cadmium treatments
Treatment of soybean seeds with different concentrations of CdCl2 reduced the seed vigour indices gradually from 0 to 100 μΜ CdCl2, thereafter significant decrease was observed at 200 and 300 μΜ in both primed and unprimed seeds (Figs. 3a, b). Vigour index-I was found decreased prominently by 75%, 92% and 94% respectively at 100, 200 and 300 μΜ CdCl2 concentration in unprimed seeds compared to their controls (Fig. 3a). Similarly, vigour index-II decreased severely at 200 and 300μΜ CdCl2 concentrations by 83% and 87% respectively in seeds compared to the control (Fig. 3b). However, magnetoprimed seeds showed higher vigour indices at all CdCl2 levels as compared to unprimed seeds. There was prominent increase in vigour index-I at 50, 100 and 200 μΜ CdCl2 concentration (84%, 165% and 43% respectively) in magnetoprimed seeds as compared to unprimed seeds (Fig. 3a). Similarly, vigour index-II increased significantly at 100, 200 and 300 μΜ CdCl2 concentration by 106, 147 and 135% respectively in magnetoprimed seeds as compared to unprimed seeds (Fig. 3b).
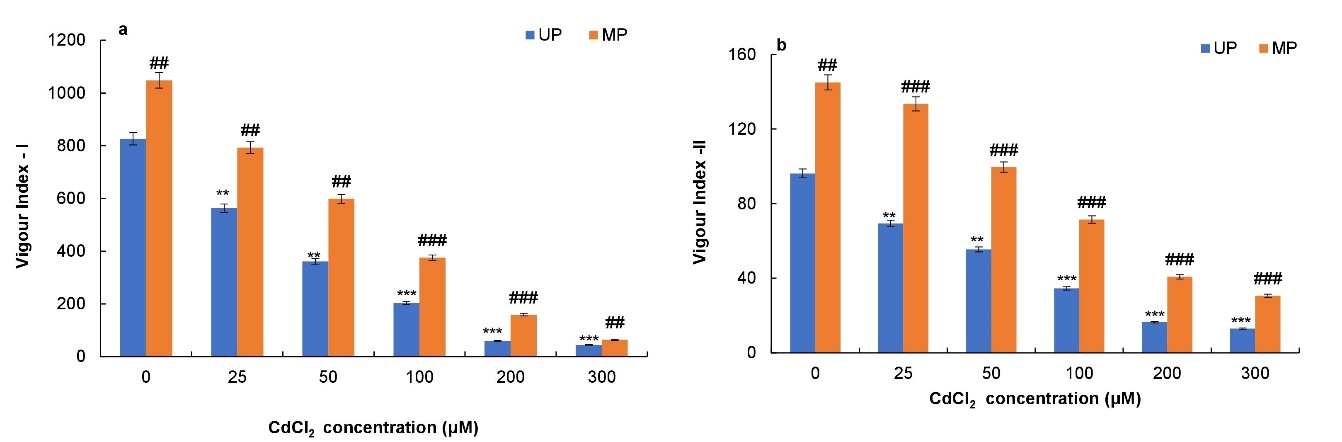
Fig. 3. Effect of magnetopriming on vigour index-I (a) and vigour index-II (b) of soybean seedlings under Cd toxicity. The vertical lines on the bar indicate ± S.E. for mean of triplicates (n = 3). Significant differences between non-stressed and Cd-stressed soybean seedlings that emerged from unprimed seeds are marked as follows:**P < 0.01,***P < 0.001. Statistically significant differences between magnetoprimed (MP) and unprimed (UP) seedlings grown in non-stress as well as Cd-stress conditions are marked as##P < 0.01,###P < 0.001.
Effect of MP on total amylase and protease activities in soybean seedlings under cadmium treatments
When soybean seeds were stressed with CdCl2, total amylase and protease activities decreased slightly in the seedlings from unprimed seeds as compared to control seedlings. However, primed seeds showed increased amylase activity by 49%, 51%, 64% and 70% at 0, 100, 200 and 300 μM CdCl2 concentrations, respectively (Fig. 4a). Further, magnetoprimed seeds showed increased protease activity by 30, 44, 54 and 53% at 0, 100, 200 and 300 μM CdCl2 concentrations, respectively (Fig. 4b).
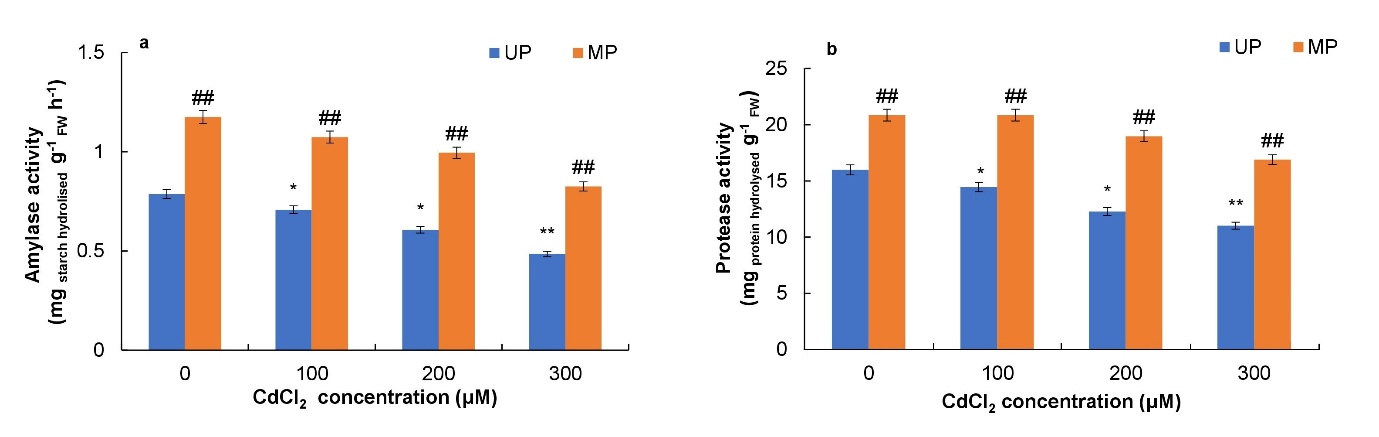
Fig. 4. Effect of magnetopriming on amount of starch hydrolysed (a) and protein hydrolysed (b) in soybean seedlings under Cd toxicity. The vertical lines on the bar indicate ± S.E. for mean of triplicates (n = 3). Significant differences between non-stressed and Cd-stressed soybean seedlings that emerged from unprimed seeds are marked as follows:*P < 0.05,**P < 0.01. Statistically significant differences between magnetoprimed (MP) and unprimed (UP) seedlings grown in non-stress as well as Cd-stress conditions are marked as##P < 0.001. FW – fresh weight.
Effect of MP on proline, MDA and H2O2 content in soybean seedlings under cadmium treatments
MDA content was found to rise gradually with increasing concentration of CdCl2 in unprimed as well as primed seedlings. However, the level of MDA was higher in seedlings emerged from unprimed seeds at all the concentrations of CdCl2 used (Fig. 5a). MDA content was decreased in the seedlings from SMF-primed seeds as compared to the seedlings from unprimed seeds at all the concentrations of CdCl2 (0 to 300 μM) (Fig. 5a). A remarkable decrease in MDA content (by 37%) was observed at 300 μM CdCl2 concentration in the seedlings from primed seeds as compared to the seedlings of unprimed seeds.
Proline content was considerably increased in seedlings of unprimed seeds at all the concentrations of CdCl2 (Fig. 5b). The enhancement in proline content was 39, 92 and 100% in seedlings of unprimed seeds at 100, 200 and 300 μM CdCl2 concentrations as compared to the control seedlings. Magnetopriming of seeds lowered the proline quantity at all concentrations of CdCl2. There was 23% decrease in proline content at 200 μM and 300 μM CdCl2 concentration in primed seeds (Fig. 5b).
The cadmium treatments increased the H2O2 content by 26% (200 μM CdCl2) and 35% (300 μM CdCl2) in the seedlings of unprimed seeds as compared to their controls (Fig.5c). Magnetopriming also caused a further increase in H2O2 content in soybean seedlings in comparison to unprimed seeds at all the cadmium chloride concentrations.
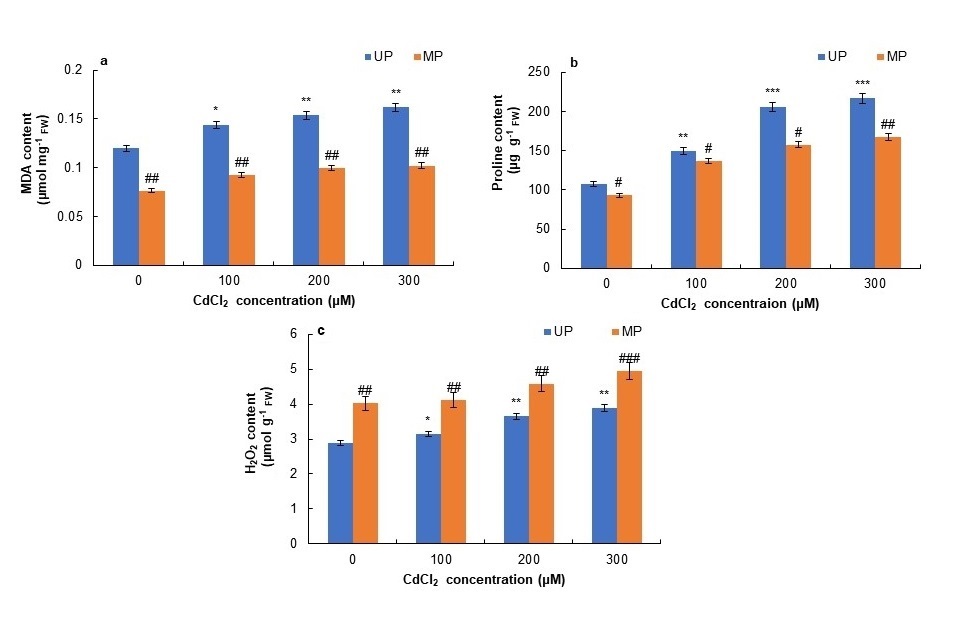
Fig. 5. Effect of magnetopriming on malondialdehyde - MDA (a), proline (b) and H2O2 (c) content of soybean seedlings under Cd toxicity. The vertical lines on the bar indicate ± SE for mean of triplicates (n = 3). Significant differences between non-stressed and Cd-stressed soybean seedlings that emerged from unprimed seeds are marked as follows:*P < 0.05,**P < 0.01,***P < 0.001. Statistically significant differences between magnetoprimed (MP) and unprimed (UP) seedlings grown in non-stress as well as Cd-stress conditions are marked as#P < 0.05,##P < 0.01. FW – fresh weight.
Effect of MP on tolerance index (TI) of soybean seedlings under cadmium treatments
TI was 100% for control seedlings and it decreased with an increase in CdCl2 toxicity (Fig. 6). The TI after treatment with 25, 50, 100, 200 and 300 μM CdCl2 was 78, 45, 25, 8 and 0%, respectively, for soybean seedlings obtained from unprimed seeds (Fig. 6). Whereas seeds pre-treated with MP showed higher values of TI at all the levels of CdCl2 i.e. 87, 61, 46, 22 and 2% TI compared to values from unprimed seeds at 25, 50, 100, 200 and 300 μM CdCl2, respectively.
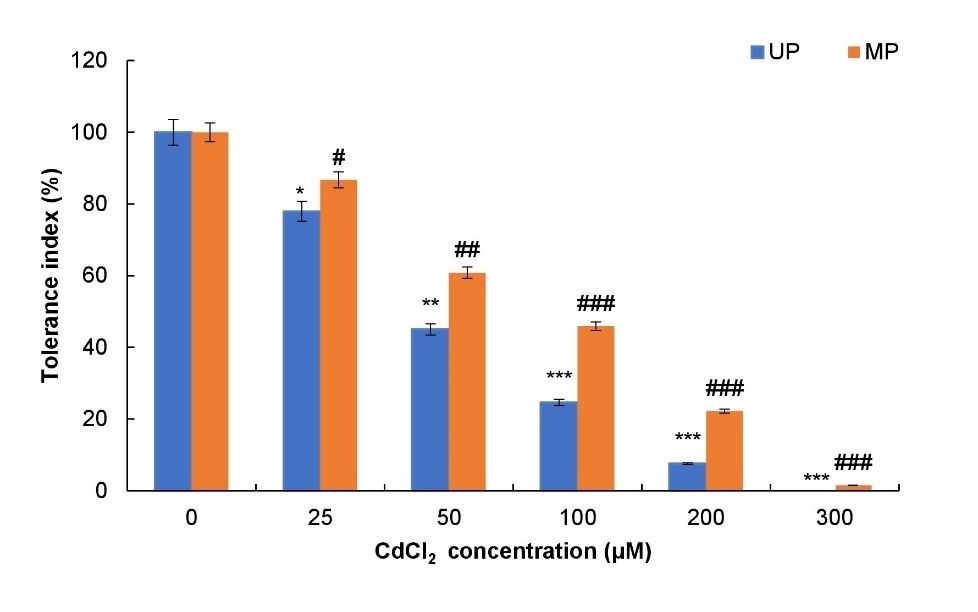
Fig. 6. Effect of magnetopriming on tolerance index of soybean seedlings in terms of root length under Cd toxicity. The vertical lines on the bar indicate ± SE for mean of triplicates (n = 3). Significant differences between non-stressed and Cd-stressed soybean seedlings that emerged from unprimed seeds are marked as follows:*P < 0.05,**P < 0.01,***P < 0.001. Statistically significant differences between magnetoprimed (MP) and unprimed (UP) seedlings grown in non-stress as well as Cd-stress conditions are marked as#P < 0.05,##P < 0.01,###P < 0.001.
Discussion
Amongst heavy metals, Cd is a toxic environmental contaminant, which has detrimental effect on plants, animals and humans. It is often found in industrial and agricultural waste and can contaminate soil, water, and food (Yin et al. 2021). The germinating seed serves as the first point of interaction between the developing plant and its surrounding environment, making it particularly susceptible to the toxic effects of Cd (Stefanello 2019, Mahawar et al. 2021). Cd significantly inhibited the seed germination of various plant species such as swiss chard, lettuce, wheat, basil, chia, beans and spinach (Ahmad et al. 2012, Bautista et al. 2013, El Rasafi et al. 2016, Gharebaghi et al. 2017, Stefanello 2019).
The results of the present investigation also showed that, in seedlings derived from unprimed seeds, Cd treatments significantly inhibit seed germination and early seedling growth parameters (root, shoot, and seedling length, fresh and dry weight of seedlings, and vigour indices); a dose-response inhibition was noted for every parameter examined. Even in the presence of cadmium, however, MP of soybean seeds with SMF at 200 mT for an hour before germination improved the seeds' early development characteristics. The length of both shoot and root is critical for efficient material exchange and nutrient acquisition (Bewley and Black 1994, Stefanello 2019).The inhibition was more pronounced for root length in the seedlings from unprimed seeds grown under Cd treatments. At a higher concentration of Cd (300 μM CdCl2), the root growth was completely inhibited. Interestingly, MP with SMF enhanced root length to a greater extent than the shoot length. In the present study, positive effects of MP were observed in soybean seedlings under Cd toxicity, such as increased seed germination percentage, root, shoot, and seedling length, as well as enhanced fresh/dry weight and vigour indices of seedlings. The inhibitory effects of Cd on shoot and root growth lead to stunted seedlings as Cd toxicity disrupts the water and nutrient uptake and transport (Liu et al. 2012, Sheirdil et al. 2012, Stefanello 2019).The increase in root length induced by MP can contribute to enhanced water and nutrient uptake and provide necessary resources for seedlings to cope with the toxic effects of Cd. However, the exposure of seeds to MF has earlier been shown to increase their capacity to absorb moisture and their nutrient content in soybean (Kavi 1977, Radhakrishnan and Kumari 2012). These effects on moisture absorption and physiological activity can contribute to improved germination and seedling vigour. There have also been reports on the effects of very low-frequency alternating magnetic fields on cell membrane ionic permeability (Khizhenkov et al. 2001, Thomas et al. 2013). Studies by Kataria et al. (2019, 2020, 2021, 2023) have confirmed that applying MP with SMF-pretreatment to the seeds can have a positive effect on seed germination and seedling vigour under salt stress. Our results are consistent with the findings of several earlier studies, including those on rice, wheat, maize, soybean and barley seeds, which have also revealed that MP can improve seed germination percentage, seedling growth and vigour index (Carbonell et al. 2000, Florez et al. 2007, Martinez et al. 2009, Shine et al. 2012, Kataria et al. 2015, Ercan et al. 2022). The tolerance index represents the ability of seedlings to withstand and survive under CdCl2 toxicity, decreased with increasing concentrations of CdCl2 in both unprimed and magnetoprimed seedlings. However, the seedlings from magnetoprimed seeds exhibited a higher tolerance index than unprimed seed at all the concentrations of Cd. This suggests that MP helped to mitigate the inhibitory effects of cadmium on seedling growth and increased their tolerance to Cd toxicity. Similarly, Kataria et al. (2020, 2022) have reported that the salt tolerance index of soybean seedlings was increased by MP due to the higher nitric oxide production by SMF pre-treatment, which helps to maintain the balance of abscisic acid (ABA) and gibberellic acid (GA) for higher seed germination and seedling growth under salt stress conditions.
Furthermore, high concentrations of Cd have negative effects on hydrolyzing enzymes including acid phosphatases (ACPs) and α-amylases, they prevent reserved carbohydrates from being hydrolyzed and from moving from the endosperm to the growing embryonic axis. As a result, the embryo that is germinating becomes starved (Kuriakose and Prasad, 2008). Amylase is an enzyme that breaks down the stored carbohydrate reserves of a seed during germination. Also, it has been shown that Cd's interactions with proteolytic enzymes limit store protein catabolism, which results in the slowing of seedling growth (Gianazza et al., 2007). The magnetoprimed soybean seeds maintained a higher total amylase and protease activity than unprimed seeds under both non-stress and in cadmium stressed conditions. This suggests that the MP may have influenced the biochemical mechanisms involved in seed germination and growth, including amylase and protease activity. Our finding is consistent with the results of previous studies. For instance, Vashisth and Nagarajan (2010) reported that magnetoprimed wheat and sunflower seeds had significantly higher amylase activity than their controls. Previous studies have reported higher α-amylase and protease activity in magnetoprimed soybean and maize seedlings under various stress conditions such as salt, mercury toxicity and UV-B (Kataria et al. 2017, 2019, 2020, 2023, Raipuria et al. 2021, Prajapati et al. 2023). The higher protease activity in magnetoprimed seeds could contribute to the breakdown of stored proteins into amino acids (Rajendra et al. 2005) that can be utilized for energy and growth during seedling development. The results suggest that MP can enhance the seedling's ability to cope with stress conditions by increasing total amylase and protease activities, which might improve the crop tolerance under Cd toxicity. Theoretical studies have shown that a magnetic field may enhance the density of ions passing through the cellular membrane of a seed, so affecting the osmotic pressure in a way that favours water entering into the cell (Reina et al. 2001). As previously indicated, seeds exposed to either a static or an oscillating magnetic field had higher levels of enzyme activity, particularly α-amylase (Kataria et al. 2017, 2020). The enzymes α-amylase which help the seed use its stored starch energy, are essential for seed germination. Therefore, a larger amount of solvent would be supplied to the seed's germination enzymes more quickly by the magnetic field's osmotic pressure action (Diehl 2022).
Interestingly, MP was found to lower the levels of MDA and oxidative stress marker (proline) and enhanced the H2O2 content in soybean seedlings in the presence of Cd toxicity. This suggests that MP may play a protective role against oxidative damage caused by CdCl2 stress in plants. Similar findings were reported by Chen et al. (2011), where MF treatment was found to reduce the toxic effects of Cd on mung bean by increasing the rate of photosynthesis, nitric oxide concentration, and nitric oxide synthase activity, and reducing the lipid peroxidation. Chen et al. (2017) found the alleviation of the adverse effect of Cd and lead (Pb) through MP due to enhanced lipid peroxidation and antioxidant defence system in wheat seedlings. According to Prajapati et al. (2023), MP reduced lipid peroxidation at 200 mT (1 h) while increased the H2O2 content in soybean seedlings grown under mercury toxicity. This implies that MP alters membrane stability and H2O2 content to regulate antioxidant activity. Fatima et al. (2020) also observed that MP of soybean seeds mitigated the arsenic toxicity in terms of growth, photosynthesis, and water uptake through increase in the thickness of the midrib of leaves.
Several studies have reported changes in proline content in plants under different stress conditions and magnetic treatments. Banerjee and Roychoudhury (2015) stated that proline serves as an osmoprotectant in plants cultivated in a range of stressful conditions. For instance, cadmium toxicity caused a significant increase in proline accumulation in the shoots of Brassica juncea, Triticum aestivum, and Vigna radiata, indicating that proline plays an important role in the response to cadmium stress (Alia and Saradhi 1991, Sharmila et al. 2017). Mercury treatments raised the proline content in Melissa officinalis (Safari et al. 2019) and Eichhornia crassipes (Malar et al. 2015), which is consistent with our findings. However, magnetic treatment with SMF has been found to decrease the proline content in soybean seedlings under mercury toxicity (Prajapati et al. 2023). Chickpea plants (Hozayn et al. 2022), S alvia (Khosrojerdi et al. 2023) and orange seedlings (Mahmoud et al. 2019) treated with magnetic water also showed decreased amount of proline under saline soils. Okba et al. (2022) reported a decrease in proline content with magnetic water treatment, suggesting a positive role of magnetic water in reducing the detrimental effects of water deficit stress. These authors suggested that proline is especially important during stressful situations because it helps in regulation of the osmotic pressure of the cytosol and the vacuole with that of the external environment, which improves the uptake of water and nutrients.
Plants have the capability to produce ROS in response to stress, which can have both positive and negative effects on their growth and survival (Hasanuzzaman et al. 2020). H2O2 production was enhanced markedly by Cd toxicity in a number of plant species (Ahmad et al. 2011, Mahawar et al. 2021). However, Shine et al. (2012) have shown that priming with SMF can increase the content of ROS which can serve as key signalling agents, influencing critical events in seed life, including seed germination by inducing ABA catabolism and gibberellic acid (GA) biosynthesis. Magnetoprimed seeds showed a decrease in ABA concentration and a rise in GA content in soybean under salinity suggesting that MP actively contributes to seed germination and the release of dormancy (Kataria et al. 2021). Thus the production of ROS such as H2O2 induced by MP of seeds represents one of the mechanisms through which seed invigoration and enhanced seedling growth can be achieved under nonstress as well as under abiotic stress conditions (Kataria et al. 2017, 2021, 2023, Raipuria et al. 2021). According to Barba‐Espin et al. (2010), during the germination of pea seeds, H2O2 plays a direct role as a signalling molecule within the phytohormone network, causing the induction of proteins linked to plant signalling and development processes. Therefore, H2O2 functions as a central node that harmonizes and integrates phytohormone interactions to improve magnetoprimed soybean seed germination and vigour under Cd toxicity. Therefore, MP with SMF could be a promising technique for improving germination of seeds and seedling growth by alleviating cadmium stress through improving the tolerance of soybean seedlings. But further investigation is required to completely comprehend the processes behind the advantageous effects of-MP in the presence of heavy metal toxicity.
Conclusion
In conclusion, the present study revealed that the presence of Cd had a detrimental effect on seed germination and seedling growth, and this effect was found to be concentration dependent. Total amylase and protease activity declined under Cd toxicity, suggesting that it might be the result of disruptions in the reserve mobilisation process from cotyledons to the developing embryonic axis. The tolerance index of seedlings emerged from magnetoprimed seeds indicated that MP mitigates the toxic effects induced by Cd and enhances the tolerance in soybean seedlings by promoting root length, seedling vigour, total amylase and protease activity, H2O2 and reducing MDA and proline content as compared to the seedlings from unprimed seeds under Cd toxicity. Overall, the study suggested the possibility that use of a magnetic field might mitigate the harmful effects of Cd toxicity in soybeans. Further research in this area could explore the underlying mechanisms and optimize the magnetic field parameters for improved crop productivity and environmental sustainability.