Introduction
Plant production and productivity are strongly determined by seed germination, which is a critical step in the life cycle of higher plants (Cheng and Bradford 1999). Seed germination may be asynchronous and consequently plant performance may be far from satisfactory. There are currently several approaches to overcoming this problem, including priming. Seed priming is a pregermination technique that ensures rapid, uniform and synchronized germination and improves seedling vigour and growth under normal and adverse environmental conditions (Varier et al. 2010). The priming process involves the imbibition of seeds to allow pregermination metabolic activation, followed by dehydration prior to the reversible phase (avoiding radicle breakthrough) to avoid radicle emergence (Finch-Savage and Leubner-Metzger 2006). This technique is economical and environment-friendly. There are different priming methods for improving seed germination performance, such as hydropriming (imbibing seeds in water), osmopriming (imbibing in an osmotic solution), halopriming (imbibing in a saline solution), chemopriming (imbibing in chemical solutions), and hormopriming (imbibing with phytohormones). Hormopriming, the technique used in this study, is based on treatment with plant hormones such as auxins, abscisic acid, cytokinins, and gibberellins. This type of treatment results in a higher germination performance and the resulting plants can be more tolerant to abiotic stresses including water stress (Singh and Maheswari 2017). Several authors have shown that the positive effects of priming are associated with various physiological, biochemical, cellular, molecular and genetic changes such as mobilization of reserves, degradation of albumen, stimulation of osmolyte synthesis and activation of the cell cycle and some abiotic stress tolerance genes. The activation of antioxidant enzyme systems has been extensively studied in primed seeds (Varier et al. 2010, Boucelha et al. 2019a). The signalling roles of reactive oxygen species (ROS) in seed germination and dormancy have been well documented and it is assumed that ROS accumulation is beneficial for seed germination and seedling growth (see the review by Bailly et al. 2008 and Bailly, 2023). In a more precise way, Bailly et al. (2008) proposed the concept of the "oxidative window for germination", which restricts the occurrence of the onset of germination to a critical range of ROS level. Thus, ROS homeostasis regulates the beginning of germination, which is why activity of ROS-scavenging systems plays an important role. Furthermore, several authors also studied the involvement of ROS in the priming phenomenon (Boucelha et al. 2019a, Ren et al. 2023).
The role of ROS in seed dormancy control is due to their interaction with plant hormones that have central functions in seed dormancy and germination such as gibberellic acid (GA) (see review by Bailly et al. 2008). At low levels applied to the seed, GA stimulates germination by breaking dormancy through the activation of hydrolytic enzymes such as amylases, which promote the breakdown of reserves that provide energy for germination, resulting in rapid cell division and radicle elongation (Gubler et al. 1995). ROS have been reported to stimulate GA biosynthesis through a transcriptional regulation (Li et al. 2018) and GA treatment has been shown to induce ROS production (Cembrowska-Lech et al. 2015) by modifying the redox status of aleurone proteins, a process which might be related to ROS accumulation (Maya-Ampudia and Bernal-Lugo 2006).
Few studies have focused on the redox status of root radicles following treatment with GA, particularly applied as hormopriming (Ellouzi et al. 2023). Thus, the objective of this work was to study the effect of GA applied in two different ways, by seed imbibition in GA followed by rinsing (pretreatment with GA) and by hormopriming (GA treatment followed by redehydration) on the redox status of Trigonella foenum graecum L. radicles by investigating enzymatic and non-enzymatic antioxidant systems. Fenugreek (Trigonella foenum graecum) is an annual herb that belongs to the family Leguminosae cultivated worldwide, especially in southwest Asia, Middle East and Mediterranean. Due to its strong flavour and aroma fenugreek in one of those plants whose leaves and seeds are widely consumed as a spice in food preparations, and as an ingredient in traditional medicine. Its seeds are used for their carminative, tonic and aphrodisiac effects (Chopra et al. 1986) and it is assumed to have antidiabetic effect, hypocholesterolemic influence, antioxidant potency, digestive stimulant action, and hepatoprotective effect (Srinivasan 2006).
Plant material
Our study was carried out on fenugreek seeds (Trigonella foenum-graecum L.), variety Halba from southern Algeria. Homogeneous seeds were selected from the same lot and rinsed with 5% hypochlorite solution for disinfection.
Treatments, seed germination and measurement of seedlings radicle growth
For pretreatment seeds were treated by imbibition in distilled water or 0.1 mM GA3 for 8 hours and then rinsed before germination. Hormopriming and hydropriming were performed by seed soaking in 0.1 mM GA3 or in distilled water, respectively, for 8 hours and then rinsed and dehydrated under ventilation for 48 hours before germination. Control seeds were not treated in any way before germination. Treated and control seeds were germinated in Petri dishes (9 cm in diameter) on four layers of absorbent paper soaked in distilled water. Three Petri dishes, with 50 seeds each, were used per treatment. For the first 24 hours the germination experiment was performed in an oven at 25 ° C, after which the Petri dishes were moved to a 14 hours-photoperiod of natural daylight and room temperature (24 ± 1 °C) for the next two days. After three days radicles were sampled for ROS detection, biochemical analyses and measurement of activity of antioxidant enzymes.
The length of radicle obtained from treated and untreated seeds was measured by graph paper each day for three days and expressed in cm. The fresh weight of treated and untreated radicles was measured after the 3rd day and expressed in grams.
Cytochemical detection of ROS
Hydrogen peroxide (H2O2) in situ was detected by the method of Thordal-Christensen et al. (1997), using a cytochemical method with 3,3'-diaminobenzidine (DAB). A brown colour is visible at the site of reaction of DAB with hydrogen peroxide, due to polymerisation of the DAB molecule.
Superoxide anions (O2.-) in situ were detected by a cytochemical method using nitro blue tetrazolium (NBT) (Rao and Davis 1999). The superoxide radicals present in tissue reduce the NBT to a stable formazan of blue-indigo colour (Beyer and Fridovich 1987).
Biochemical analyses
Hydrogen peroxide (H2O2) was measured according to the method described by Alexieva et al. (2001) after reaction with 1 M potassium iodide (KI) in 100 mM potassium phosphate buffer (pH 7.0). The reaction was developed for 1 hour in darkness (yellowish colour) and absorbance measured at 390 nm. The amount of hydrogen peroxide per fresh weight (FW) was calculated using a standard curve prepared with known concentrations of H2O2 (0 to 10 nM) and expressed as nmol g-1 FW.
Lipid peroxidation was measured by quantification of the coloured malondialdehyde complex (MDA-TBA) detected at a wavelength of 532 nm, as described by Popham and Novacky (1991). The MDA content was expressed as µmol g-1 FW. This was calculated using the molar extinction coefficient of MDA (ε = 155 mM-1 cm-1).
Reduced glutathione (GSH) was measured according to Moron et al. (1979) by using 5,5’-dithiobis-2-nitrobenzoic acid (DTNB) (Ellman’s reaction) to give a yellow-coloured product that absorbs at 412 nm. The measurement was calculated from the molar extinction coefficient (ε = 13.3 mM-1 cm-1) and expressed as µmol g-1 FW.
The total non-enzymatic antioxidant capacity (TAC) was estimated by the phosphomolybdenum method (Prieto et al. 1999). TAC is expressed in mg equivalents of ascorbic acid per g of dry weight. A standard curve was constructed using 0-300 μg mL-1 ascorbic acid.
Activities of antioxidant enzymes
For extraction of catalase (CAT), superoxide dismutase (SOD) and ascorbate peroxidase (APX), 100 mg of radicles were cold ground in extraction buffer (0.1 M Tris-HCl, pH 8.1). The guaiacol peroxidase (GPOX) was extracted from 100 mg of radicles ground in 0.1 M potassium phosphate buffer (KH2PO4 / K2HPO4, pH 6.5) under cold conditions. The enzyme activity was measured in protein extracts by spectrophotometry. Total soluble proteins were determined by the Bradford method (Bradford 1976).
CAT activity was determined following the decomposition of H2O2 at 240 nm using the method described by Anderson et al. (1995). The activity was expressed as µmol of H2O2 degraded per minute per mg of protein. This activity was calculated using the molar extinction coefficient of H2O2 (ε = 36 mM-1 cm-1).
GPOX activity was determined according to the method of MacAdam et al. (1992), slightly modified by Boucelha et al. (2019b). The activity is measured by a colorimetric technique based on the increase in absorbance at 470 nm due to the polymerisation of guaiacol to tetraguaiacol (oxidation), which gives an orange colour in the presence of hydrogen peroxide. The activity was expressed in μmol of oxidised guaiacol per minute per mg of protein, using the molar extinction coefficient of tetraguaiacol (ε = 26.6 mM-1 cm-1).
APX activity was measured according to the method of Nakano and Asada (1981) by following the oxidation of ascorbate by hydrogen peroxide, which absorbs at a wavelength of 290 nm. The enzymatic activity is expressed as µmol of ascorbate oxidised per minute per mg of protein. This activity was calculated using the extinction coefficient of ascorbate (ε = 2.8 mM‑1 cm-1).
SOD activity was measured by method reported by Marklund and Marklund (1974) and slightly modified by Boucelha et al. (2019a). It is based on the competition between the oxidation reaction of pyrogallol by superoxide ions and the dismutation by SOD. The increase in absorbance at 420 nm was due to the auto-oxidation of pyrogallol. An enzymatic unit was defined as the quantity of enzyme capable of inhibiting 50% of the autooxidation of pyrogallol under the conditions of the assay. The activity of SOD was expressed in units per minute and per mg of protein.
Decomposition kinetic of GA3
To study the stability of GA3 in water used for imbibition, we studied the spontaneous decomposition kinetic of 0.1 mM GA3 by spectrophotometry at 254 mM, measuring the appearance of gibberellenic acid, a degradation product, according to the method of Pérez et al. (1996).
Statistical analysis
The ANOVA test was used to compare the results. The statistical significance between the results was assessed according to the Tukey post-hoc test and values were considered statistically significant at P < 0.05. The analysis of data was performed through STATISTICA 6.0 software (Stat Soft, Inc.). All values were expressed as mean and standard deviation.
Radicles growth
Fenugreek seed pregermination treatments significantly improved radicle length, following the same, almost linear, kinetics for all treatment types (Fig. 1A). However, this improvement was closely related to the type of treatment applied. In fact, we found that GA imbibition (without redehydration) showed the best growth, with an increase of about 66% on day 3 compared to the control batch. This was followed by the hydropriming (HP) with an increase of 49%, then the imbibition with water (W) and hormopriming (HO) with an increase of 36% and 20%, respectively, compared to the control. All treatments induced an increase in the fresh weight of fenugreek radicles (Fig. 1B). The greatest increases are recorded for imbibition with GA (107%) and hormopriming (95%). For the hydropriming, the increase was 75% while for imbibitions, it was only 23%.
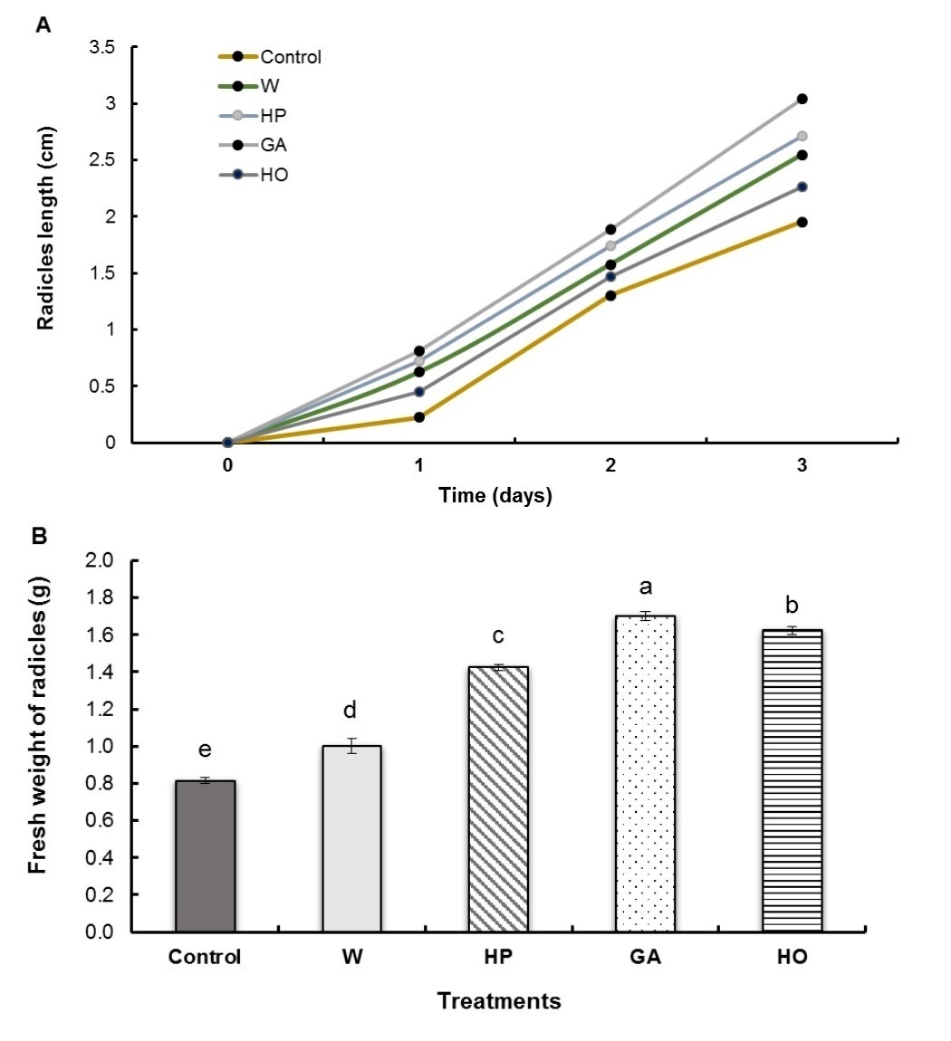
Fig. 1. Radicle length (A) and fresh weight (B) of fenugreek seedlings after different treatments of seeds. W − imbibition with water, HP − hydropriming, GA − imbibition with 0.1 mM gibberellic acid, HO − hormopriming with 0.1 mM gibberellic acid. Results are expressed as means (N = 3) and error bars represent standard errors. Different alphabetical letters indicate a significant difference (P < 0 .05) between means.
ROS production in situ
DAB assay revealed that the degree of H2O2 accumulation in tissue varied according to the type of treatment applied. Control seed radicles were characterized by the lowest production of H2O2 (Fig. 2A). A high accumulation of H2O2 was observed in the radicles of GA and hydropriming treated seeds, especially in the region of the root cap and elongation. In contrast, a low H2O2 accumulation was observed in the radicles resulting from hormone priming and water imbibition treatments.
Fenugreek radicles treated with NBT showed an accumulation of superoxide anions in the tissue (Fig. 2B). In the control radicles, the accumulation of superoxide anions was lower than in the radicles from treated seeds. Gibberellic acid imbibition showed the highest production of superoxide anions localized throughout the radicle. Hydropriming treatment also induced an accumulation of superoxide anions. However, in water imbibition and hormopriming treatments the presence of superoxide anions was only slightly visible.
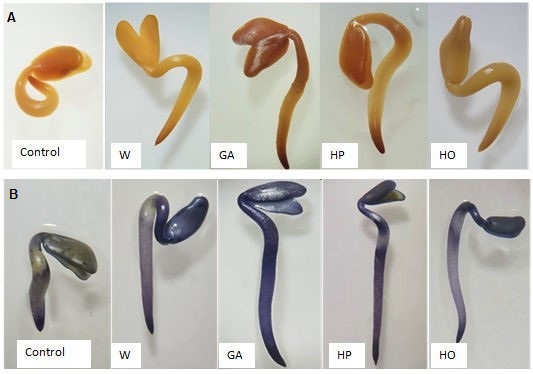
Fig. 2. Detection of reactive oxygen species in fenugreek radicles after different treatments of seeds: hydrogen peroxide (H2O2) after 24 hours of germination (A) and superoxide anion (O2.-) after 48 h of germination (B). W − imbibition with water, HP − hydropriming, GA − imbibition with 0.1 mM gibberellic acid, HO − hormopriming with 0.1 mM gibberellic acid.
Hydrogen peroxide and malondialdehyde content
Results indicate that the pregermination treatments of fenugreek seeds induced a significant increase in H2O2 concentration in the radicles except for water imbibition (Fig. 3A). Indeed, hydropriming and GA imbibition resulted in almost the same increase in H2O2 content (101% and 98%, respectively), as the control. An increase of 23% was observed in the case of the hormopriming treatment compared to the control.
Pregermination treatments of fenugreek seeds showed a significantly higher MDA content of control radicles than the radicles of treated seeds (Fig. 3B). Seed imbibition with gibberellic acid, favoured a decrease in MDA content for 44% compared to the control. This was followed by the hydropriming treatment (decrease of 24%), then the hormopriming treatment (decrease of 16 %) and finally the water imbibition treatment (decrease of 14%) compared to the control.
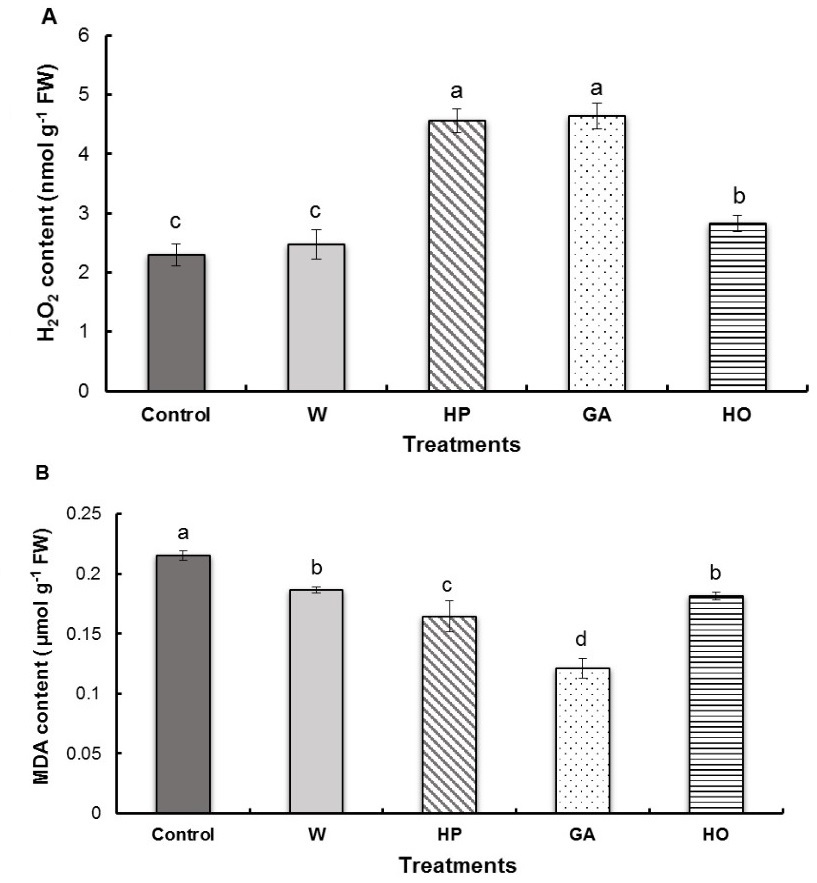
Fig. 3. Contents of hydrogen peroxide (H2O2) (A) and malondialdehyde (MDA) (B) in the radicles of fenugreek seedlings after different treatments of seeds. W − imbibition with water, HP − hydropriming, GA − imbibition with 0.1 mM gibberellic acid, HO − hormopriming with 0.1 mM gibberellic acid. Results are expressed as means (N = 3) and error bars represent standard errors. Different alphabetical letters indicate a significant difference (P < 0 .05) between means.
Activities of antioxidative enzymes
The pregermination treatment of fenugreek seeds induced a significant increase in antioxidant enzyme activities in some treatments (Fig. 4).
We observed significantly increased activity of catalase in seedlings from the gibberellic acid and hydropriming treated seeds, with increases of 88% and 33%, respectively, compared to the control. However, a less significant increase was observed in the water-imbibition (15%) and hormopriming treatment (12%) (Fig. 4A).
GPOX activity was significantly higher in fenugreek radicles pretreated with gibberellic acid, with an increase of 46% compared to the control. Hydropriming had a less prominent effect on the activation of this enzyme, with an increase of 16% over the control. In contrast, hormopriming and water-imbibition of the seeds had no effect on GPOX activity in the radicle (Fig. 4B).
The results of the APX activity showed that the pretreatments caused a very significant activation of this enzyme. Indeed, GA imbibition and hydropriming caused the significantly highest increase compared to the control (252% and 215% respectively), while in hormopriming and water-imbibition, the increase was of 188% and 148% respectively (Fig. 4C).
The antioxidant enzyme activity of SOD in the radicle revealed that GA imbibition and hormopriming induced respectively significant increases of 29% and 17% over the control. A less significant increase was observed in the activity of this enzyme for hormopriming while water-imbibition treatments did not show any significant change from the control (Fig. 4D).
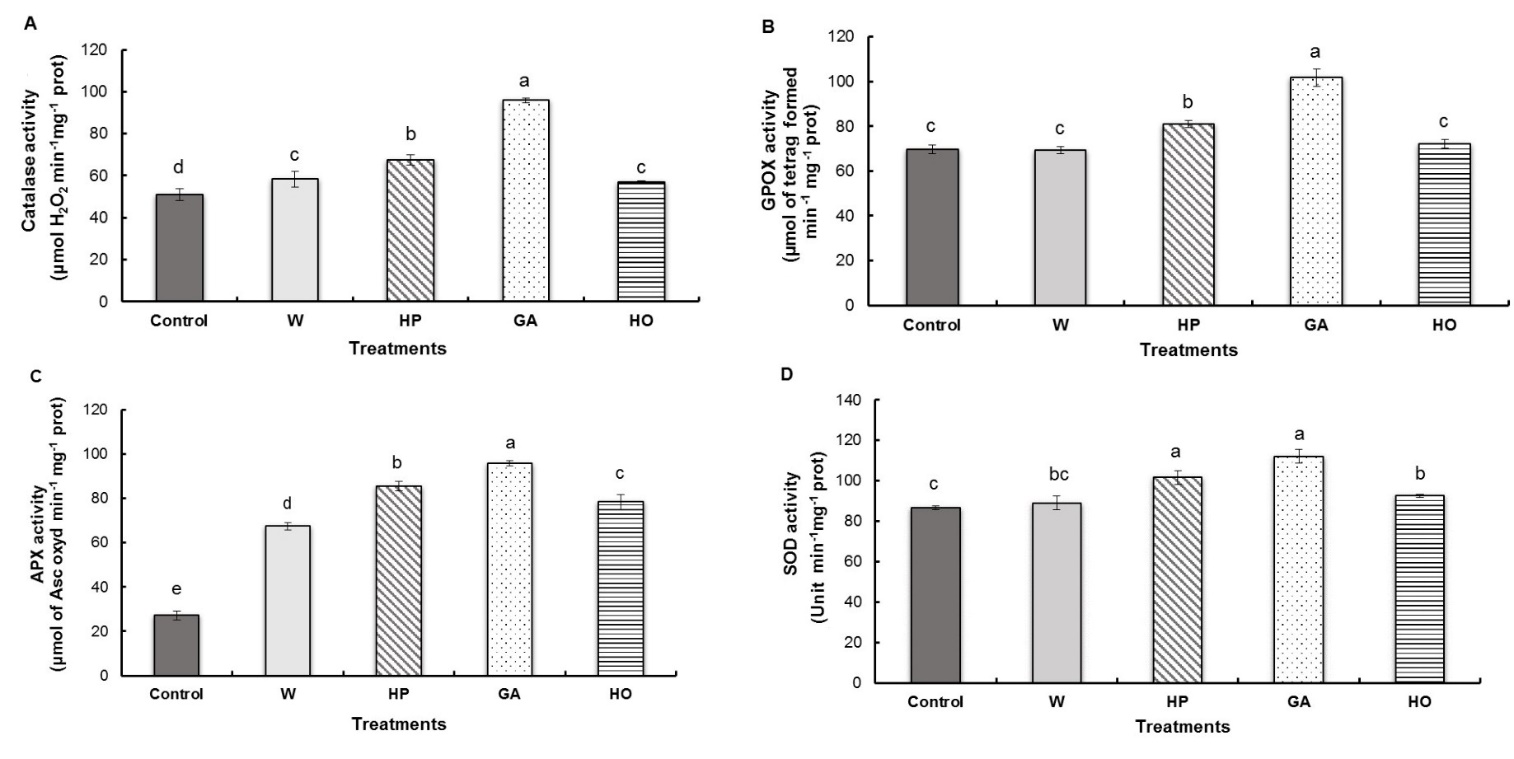
Fig. 4. Antioxidant activities of catalase (A), guaiacol peroxidase – GPOX (B), ascorbate peroxidase – APX (C) and superoxide dismutase – SOD (D) in fenugreek radicles after different treatments of seeds. W − imbibition with water, HP − hydropriming, GA − imbibition with 0.1 mM gibberellic acid, HO − hormopriming with 0.1 mM gibberellic acid. Results are expressed as means (N = 3) and error bars represent standard errors. Different alphabetical letters indicate a significant difference (P < 0 .05) between means.
Total antioxidant capacity and reduced glutathione content
All seed treatments significantly stimulated antioxidant capacity in the radicles of fenugreek seedlings; however, this increase was dependent on the type of treatment applied (Fig. 5A). Indeed, we observed that radicles soaked with gibberellic acid were characterized by the highest value in comparison to control (45%) followed by hydropriming (32%), hormopriming (22 %) and water-imbibition (10%) compared to the control.
Gibberellic acid and hydropriming treatments of fenugreek seeds caused a high increase in reduced glutathione content in the radicles (Fig. 5B) with percentages of 72% and 55% respectively compared to the control. However, we observed that hormopriming induced a weaker increase with 15% while water-imbibition treatment did not present any significant difference with the control.
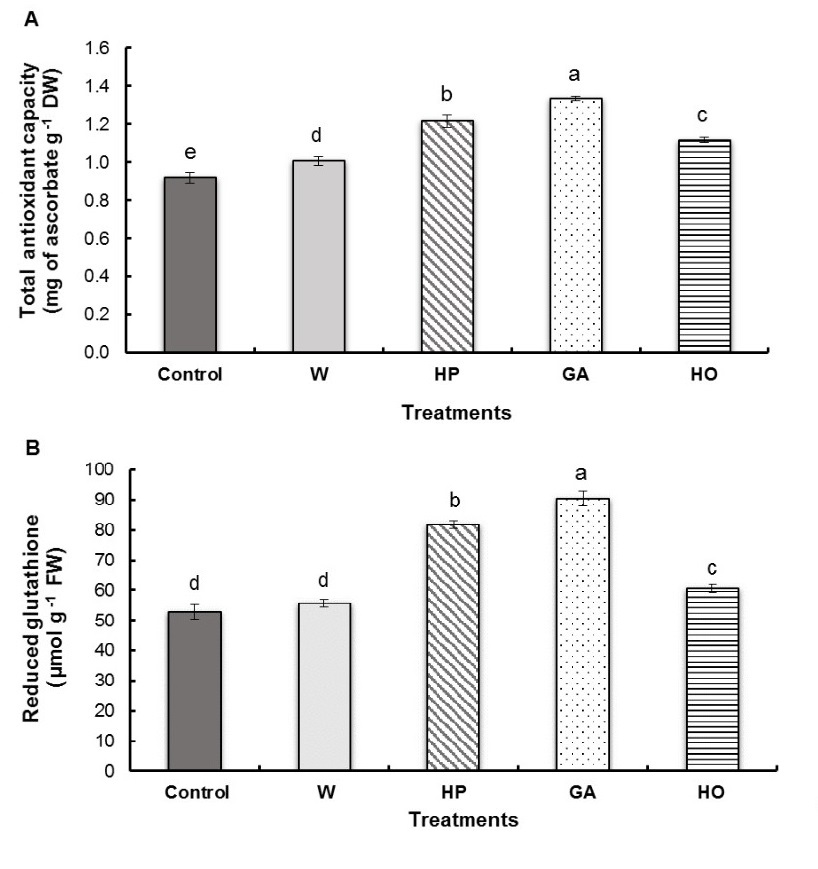
Fig. 5. Total antioxidant capacity (A) and reduced glutathione content (B) of fenugreek radicles after different treatments of seeds. W − imbibition with water, HP − hydropriming, GA − imbibition with 0.1 mM gibberellic acid, HO − hormopriming with 0.1 mM gibberellic acid. Results are expressed as means (N = 3) and error bars represent standard errors. Different alphabetical letters indicate a significant difference (P < 0 .05) between means.
Gibberellic degradation
In order to follow the stability of gibberellic acid in an aqueous medium, we followed its spontaneous degradation at 25 °C. From the curve obtained (Fig. 6), we were able to deduce that the gibberellic acid solution decomposes spontaneously into gibberellinic acid (inactive form).
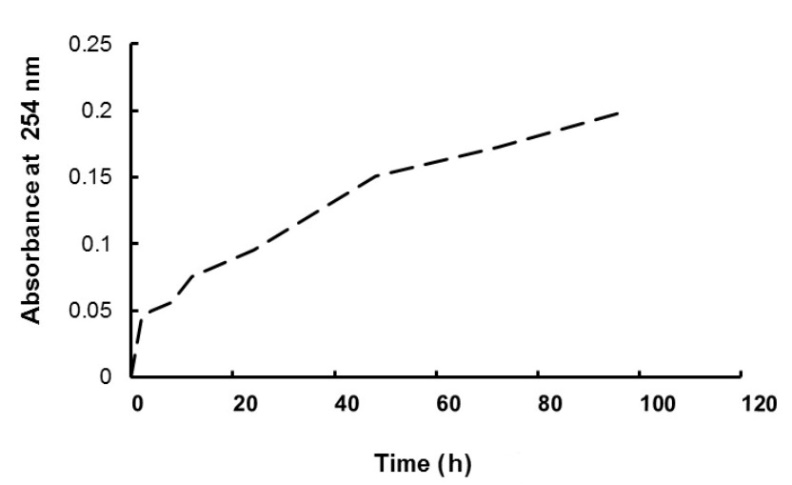
Fig. 6. Kinetics of gibberellic acid degradation at 25 °C represented by gibberellinic acid appearance.
Discussion
In this study, we found that pregermination treatments of fenugreek seeds, particularly imbibition with gibberellic acid, induced biochemical changes in the radicles derived from these fenugreek seeds and consequently better seed germination and seedling growth.
A significant improvement in germination performance was observed in Trigonella foenum-graecum L. seeds that had undergone pregermination treatments, particularly in the gibberellic acid and hydropriming treatments, followed by the hormopriming and water imbibition.
Hydropriming is known to improve seed germination performance and has been reported for rice (Hussain et al. 2015), sunflower (Hussain et al. 2006), wheat (Ahmadi et al. 2007), maize (Janmohammadi et al. 2008), bean (Ghassemi-Golezani et al. 2010), lentil (Saglam et al. 2010) and black-eyed bean (Boucelha et al. 2019a). These authors have shown that priming was an effective method for improving germination performance, resulting in uniform and homogeneous cultures. Several authors have suggested that this improvement is related to changes at the seed level, such as accelerated water uptake (Gelormini 1995), increased respiration intensity Corbineau et al. (2000), nucleic acid synthesis, strong degradation of reserves (Varier et al. 2010), activation of antioxidant enzyme activities (Amooaghaie and Vaviland 2011) and strong protein synthesis under genetic control (Varier et al. 2010). All these phenomena could be the consequence of a "memorization by the embryo" of events that occurred during the redehydration imposed during priming. It has been shown that a plant can store information when exposed to stress events and can use this memory to aid responses when these events reoccur (see review by Kinoshita and Seki, 2014). It has been demonstrated that epigenetic mechanisms are essential for stress memory and adaptation in plants (Chen and Arora 2013). Gibberellic acid is known to play a role in stimulating seed germination and is involved in many physiological and biochemical processes in plants (Mirheidari et al. 2022). Moreover, gibberellic acid is widely used in the laboratory and greenhouse to trigger the germination of some seeds that would otherwise remain dormant (Riley 1987). Thus, several works have reported the use of gibberellic acid as an exogenous treatment to enhance germination (Seandhalaksmi et al. 2022). Based on the work of Ogawa et al. (2003), exogenous GA enters the seed and is added to endogenous GA. Indeed, these authors showed that GA-deficient Arabidopsis thaliana seeds could not germinate without the addition of exogenous GA. This improvement has been linked to the synthesis and activation of amylases (Vieira et al. 2002) and lipases (Jridi et al. 2004), allowing accelerated germination with faster division cells (Li et al. 2018). Thus, seeds treated with gibberellic acid showed significant cell elongation compared to the other treatments. This cell expansion would also be due to the GA activation of aquaporins, membrane channels involved in water transport, which increases cell turgidity (Ogawa et al. 2003).
The treatments applied to fenugreek seeds stimulated the production of ROS in embryonic tissues, more precisely in the meristematic zone. This ROS formation, as evidenced by cytochemical tests and increased H2O2 content, was more pronounced in the gibberellic acid and hydropriming treatments. These effects are in line with the concept of the "oxidative window" proposed by Bailly et al. (2008) and confirmed in the case of hydropriming by Boucelha et al. (2019a). According to Bailly et al. (2008), for seed germination it is necessary that ROS content be within a range that allows signalling, while lower or higher amounts of ROS would lead to inability to germinate. Published data have shown that ROS are key players in several physiological processes in seeds such as seed dormancy control due to their interaction with plant hormones like gibberellic acid (Finkelstein et al. 2008) and the perception and transduction of environmental conditions during imbibition (Bailly 2019). In dry seeds, i.e at low moisture content, ROS accumulation would probably result mainly from non-enzymatic reactions. In this physiological state, glucose and amino groups derived from amino acid and nucleic acids are condensed to form Amadori and Maillard products, which are major sources for ROS production (Sun and Leopold 1995) and lipid peroxidation (McDonald 1999). However, during imbibition, the reactivation of metabolism causes an enhanced accumulation of ROS, generally resulting from electron leakage within the mitochondrial electron transport chain (Kranner et al. 2010).
Bailly et al. (2008) proposed a mechanism for the control of dormancy and germination through a dialogue between ROS and hormone. Indeed, according to these authors, there is an interaction between ROS and the gibberellic acid signalling pathway during germination. Under imbibition conditions, NADPH oxidase and β-oxidation increase ROS levels, which in turn repress the DELLA protein responsible for the negative regulation of GA synthesis. This induces the synthesis of gibberellic acid, which then triggers the activation of GA-inducible transcription factors GAMYB which in turn induces the transcription of α-amylase in the aleurone layers of many seeds (Gubler et al. 1995, Kaneko et al. 2002).
Membrane lipid peroxidation, which causes damage to cell membranes, is a good indicator of the presence of reactive forms of oxygen and thus allows assessment of cellular oxidative stress intensity. Oracz et al. (2007) supported the hypothesis of an inverse correlation between MDA content and seed dormancy since they observed that the MDA content increased during dormancy alleviation. Our results showed a more pronounced decrease in MDA content in the gibberellic acid and hydropriming treatments compared to the control as well as hormopriming and water imbibition where the decrease was less prominent. A reduction in lipid peroxide content in hydroprimed seeds has already been reported by El-Araby and Hegazi (2004) for tomato and Sharma et al. (2014) for okra seeds. This decrease was also observed in gibberellic acid-treated seeds, as shown in the work of Li et al. (2013) and Ahmad (2010). The reduction in MDA accumulation in primed seeds could be explained by improved membrane repair during the priming process and induction of antioxidant enzymes (Nawaz et al. 2013). For all treatments in this study, the decrease of MDA content correlated with the increase of the different antioxidative enzymes activities as well as increased level of glutathione, but did not correlate with the level of ROS. Therefore, the MDA content could be the result of the enhanced antioxidative defence which prevents oxidative damage despite higher level of ROS. Thus, cells maintain ROS homeostasis during germination (Li et al. 2013).
Fenugreek seed treatment stimulated the activation of antioxidant enzymes such as superoxide dismutase, catalase, ascorbate peroxidase and guaiacol peroxidase at the radicle level, with the most significant effect in the gibberellic acid pretreatment. These results are in agreement with those of different authors who showed that treatment of seeds with gibberellic acid leads to an increase in enzymatic antioxidant activity (Li et al. 2013). The increase in antioxidant enzymes activities was also observed in fenugreek radicles after hydropriming treatment although less prominently. Several studies showing that hydroprimed seeds of several crop species are characterised by very high antioxidant activities (Varier et al. 2010, Boucelha et al. 2019a, Melzi Ou Mezzi et al. 2021). Thus, several works have linked germination improvement to increased antioxidant enzymatic activities, which allow the elimination of free radicals, and, then, the restoration of the homeostasis of the redox status (Varier et al. 2010, Boucelha et al. 2019a, Boucelha et al. 2021).
Total antioxidant capacity corresponds to the presence of natural antioxidants capable of preventing oxidative damage (Priando et al. 1999). These non-enzymatic antioxidants include glutathione, ascorbic acid (vitamin C), tocopherols (vitamin E), carotenoids and phenolic compounds including flavonoids (Asada 2006). TAC levels reflect the reduced state of these molecules. Our results showed that radicles from seeds soaked in gibberellic acid had the highest total non-enzymatic antioxidant activity, which is in agreement with lower MDA content. Few studies have measured non-enzymatic antioxidant activities after pregermination treatments. Boucelha et al. (2019a) showed no significant changes in hydroprimed Vigna unguiculta seeds, while the results of Melzi Ou Mezzi et al. (2021) suggested that hydropriming stimulates total antioxidant activities in fenugreek radicles.
Imbibition in gibberellic solution (without redehydration) and hydromopriming induced separately increases in almost all parameters (radicles growth, H2O2 content, catalase, GPOX, APX and SOD activities, TAC, GSH content). However, hormopriming in which seeds were dehydrated after treatment with gibberellic acid resulted in values more like those obtained for seeds imbibed in water. This could mean that some of the exogenous gibberellic acid that entered the seed during imbibition may be degraded to gibberellenic acid during drying. Pérez et al. (1996) reported that gibberellic acid loses its biological activity in aqueous alkaline solutions over time by degradation to gibberellenic acid via an isomeric form iso-GA (degradation intermediate), both of which are inactive and do not induce amylase activity in barley endosperm. On the basis of these observations and our results, we suggest that drying the seeds for 48 h caused a partial degradation of GA to inactive forms, which was unable to exert a beneficial effect on the seeds.
Conclusion
Pretreatment of seeds with gibberellic acid and hydropriming resulted in improved germination performance and balanced redox status of fenugreek (Trigonella foenum graecum L.) seeds. These physiological and biochemical changes at the radicle level would be the result of the activation of certain cell signalling pathways leading to a change in gene expression that needs to be further elucidated.