Introduction
Polyphenols are secondary plant metabolites that can be classified into different groups like phenolic acids, flavonoids, stilbenes and lignans. Flavonoids can be divided into flavonols, flavones, isoflavones, flavanones, anthocyanidins and flavanols. These differences between polyphenol groups are made on the basis of different number of phenol rings and structural elements which they contain (Bravo, 1998;Manach et al., 2004). Many studies have found that polyphenols showed numerous positive bioactivities (Camouse et al., 2005;Kampa et al., 2007;Pandey et al., 2009;Scalbert et al., 2005). Furthermore, polyphenols showed that they can interact with macromolecules like proteins, carbohydrates and lipids (Jakobek, 2015;Le Bourvellec, 2012). It has been shown that these interactions can affect the bioavailability of polyphenols (Palafox-Carlos et al., 2011). The interest in the interactions between polyphenols and dietary fiber like β-glucan has increased recently. β-glucan is a natural dietary fiber, which belongs to polysaccharides, and it can be found in cereals, mushrooms, seaweed and yeast (Laroche et al., 2007). It showed many positive activities like lowering the cholesterol, it was found to be very successful in the diabetes treatment, antioxidant activity and others (Chen et al., 2008;Kofuji et al., 2012;Queenan et al., 2007). Interactions can be interpreted by studying the adsorption processes at a constant temperature and their linear adsorption models, which are called adsorption isotherms. Overall, from the parameters of linear adsorption models, information about the adsorption processes (interactions) could be obtained, like whether the adsorption is physical or chemical, are interactions favored, or if the adsorption is happening in a single layer or in multiple layers on the adsorbent surface. Also, the adsorption capacity can be determined from the experimental data (Foo et al., 2010). The aim of this work was to obtain the information about interactions between polyphenols and β-glucan from barley through parameters of linear adsorption models like the Freundlich's, Langmuir's, Dubinin-Radushkevich's, Tempkin's and Hill's. The unadsorbed polyphenols in model solutions were measured with the spectrophotometric methods like the Folin-Ciocalteu method for gallic acid, and the pH differential method for cyanidin-3-galactoside and cyanidin-3-glucoside.
Materials and methods
Chemicals
The methanol (HPLC grade) was purchased from J. T. Baker (the Netherlands). Gallic acid monohydrate (398225 ≥ 98%) and β-D-glucan from barley (G6513 ≥ 95%) were purchased from Sigma-Aldrich (St. Louis, MO, USA), while cyanidin-3-O-galactoside chloride (ideain chloride - 0923 S ≥ 97%) and cyanidin-3-O-glucoside chloride (kuromanin chloride - 0915 S ≥ 96) were purchased from Extrasynthese (Genay, France). Sodium carbonate and potassium chloride were purchased from Gram-mol (Zagreb, Croatia). Folin-Ciocalteu reagents, sodium acetate trihydrate, sodium phosphate dodecahydrate and sodium dihydrogen phosphate dehydrate, which were used for phosphate buffer preparation, were purchased from Kemika (Zagreb, Croatia).
Standards preparation
The stock solutions of polyphenol standards were prepared in the concentration of 1000 mg/L for gallic acid, 485 mg/L for cyanidin-3-galactoside and 480 mg/L for cyanidin-3-glucoside in methanol. Polyphenol concentrations in model solutions were monitored by the calibration curves obtained by preparing different concentrations of standards from the stock solution. The dillutions for the gallic acid were 1, 10, 50 100, 200, 500, and 1000 mg/L, for the cyanidin-3-galactoside and the cyanidin-3-glucoside 1, 10, 50 100, and 200 mg/L. The calibration curves were constructed by the Folin-Ciocaletu method for gallic acid and the pH diferential method for cyanidin-3-galactoside and cyanidin-3-glucoside. Prior to measurement, stock solutions were stored in a freezer at -18 °C. β-glucan stock solution was prepared in the concentration of 190 mg/L in distilled water. The prepared stock solution of β-glucan was heated for 15 min at 80 °C and stored in a refrigerator at 4 °C.
Spectrophotometric method for total polyphenols (Folin-Ciocalteu method)
Total polyphenols were determined by the Folin-Ciocaletu method (Singleton et al., 1999). 20 μL of polyphenol standard, 1580 μL of distilled water, 100 μL of the Folin - Ciocalteu reagent and 300 μL of Na2CO3 (200 g/L) were added into a glass tube. The solution was mixed in the vortex, incubated at 40 °C for 30 min in the water bath, and the absorbance was measured at 765 nm against the blank solution (which contained 20 µL of distilled water instead of the prepared solution) with a UV-Vis spectrophotometer (Selecta, UV 2005, Barcelona, Spain). Gallic acid solutions were measured by applying the same procedure, the calibration curve was constructed and used for the calculation of a gallic acid concentration after the adsorption process (in mg/L).
Spectrophotometric method for anthocyanins (pH differential method)
Cyanidin-3-galactoside and cyanidin-3-glucoside were determined by the spectrophotometric pH differential method (Tonutare et al., 2014). For this method sodium acetate trihydrate buffer (0.4 M, pH 4.5) and potassium chloride buffer (0.025 M, pH 1.0) were used. 500 µL of anthocyanin standard and 1500 µL of buffer solutions pH 1.0 were added into a first glass cuvette. 500 µL of anthocyanin standard and 1500 µL of buffer solutions pH 4.5 were added into a second glass cuvette. The solutions in two glass cuvettes were incubated for 15 min at a dark place. The absorbance was measured at 510 nm and at 700 nm with a UV-Vis spectrophotometer against the blank solution (which contained 500 µL of distilled water instead of the prepared solution). The absorbance was calculated (Eq. 1):
where:
A = absorbance of standard,
A510 = absorbance at 510 nm and
A700 = absorbance at 700 nm.
Anthocyanin calibration curves were constructed by plotting the measured absorbance against the anthocyanin concentrations. They were used for the calculation of anthocyanin concentration after the adsorption process (in mg/L).
The study of adsorption between polyphenols and β-glucan
Adsorption of polyphenols onto β-glucan was monitored in model solutions which were added in plastic tubes in total volume of 500 µL. Model solutions contained β-glucan, polyphenol and a phosphate buffer (0.13 M, pH 5.5) (Wu et al., 2011) with the final concentration of β-glucan 5 mg/L, gallic acid 50, 100, 150, and 400 mg/L, cyanidin-3-galactoside and cyanidin-3-glucoside 25, 50, 100, and 150 mg/L, and the rest of the volume was phosphate buffer. The adsorption was carried out for 16 h at 25 °C for all model solutions. After 16 h, the model solutions were centrifuged (Eppendorf minispin centrifuge, Hamburg, Germany) through a polyethersulfon membrane (Sartorius, Vivaspin 500, 10000 MWCO, 100 - 500 µL). The adsorbed polyphenols retained on the membrane, while the unadsorbed polyphenols passed through the membrane. The unabsorbed polyphenols were determined by the Folin-Ciocalteu method and the method for anthocyanins.
The adsorption capacity was calculated (Eq. 2):
where:
qe = polyphenols adsorption capacity onto β-glucan (mg/g),
γ1 = initial concentration of polyphenols in model solution (mg/L),
γ2 = equilibrium concentration of polyphenols in model solution (mg/L),
V1 = volume of model solution (L),
γ3 = β-glucan concentration in model solution (g/L) and
V2 = volume of β-glucan in model solution (L).
Furthermore, isotherm parameters of Freundlich's Langmuir's, Dubinin-Radushkevich's, Tempkin's and Hill's models were calculated according toTable 1 (Foo et al., 2010;Marsal et al., 2012;Babaeivelni et al., 2013;Soto et al., 2011).
Statistical analysis
For the data analysis MS Excel was used. Calibration curves were constructed by two replicate samples of each standard concentration, each measured twice (n=4). The concentration of polyphenols in model solutions was measured twice (n=2). In order to obtain linear equations of different adsorption models and corresponding determination coefficient R2, linear regression function was used.
Results and discussion
The adsorption isotherms are used for obtaining the information about interactions between polyphenols and different adsorbents (Gao et al., 2012;Gao et al., 2013;Huang, et al., 2007;Marsal et al., 2012;Timothy et al., 2014). There are only a few papers that describe adsorption isotherms of polyphenol adsorption onto the β-glucan (Gao et al., 2012;Wu et al., 2011). The effects of the molecular structure of polyphenols with β-glucan were also investigated (Wang et al., 2013). In this work, we studied the adsorption process between polyphenolic compounds and -glucan, as a natural dietary fiber, through the linear adsorption models. Molecular structures of polyphenols like gallic acid, cyanidin-3-galactoside and cyanidin-3-glucoside, which have been used in this study, are presented inFig. 1. Gallic acid is a simple molecule which belongs to the phenolic acid subgroup. On the other hand, cyanidin-3-galactoside and cyanidin-3-glucoside are anthocyanins which belongs to the group of flavonoids. They are glycosylated at a position 3 with a glucose at cyanidin-3-glucoside molecule and with a galactose at cyanidin-3-galactoside molecule (Bravo, 1998;Manach et al., 2004).Fig. 2,3 and4 represent linear adsorption models of Freundlich's, Langmuir's, Dubinin-Radushkevich's, Tempkin's and Hill's isotherms for gallic acid, cyanidin-3-galactoside, and cyanidin-3-glucoside adsorption onto β-glucan. The linear equation and the corresponding determination coefficient R2 are associated to each model. Due to the determination coefficient, it can be seen that Freundlich's model fits the best for gallic acid (R2 is 0.9966), followed by the Hill's, Tempkin's and Dubinin-Radushkevich's model (Fig. 2). For cyanidin-3-galactoside Langmuir's model (R2 is 0.9741) fits the best, followed by the Freundlich's, Tempkin's, Hill's and Dubinin-Radushkevich's model (Fig. 3). The Freundlich's model fits the best for cyanidin-3-glucoside (R2 is 0.8624), followed by the Hill's, Tempkin's, Langmuir's and Dubinin-Radushkevich's models (Fig. 4).
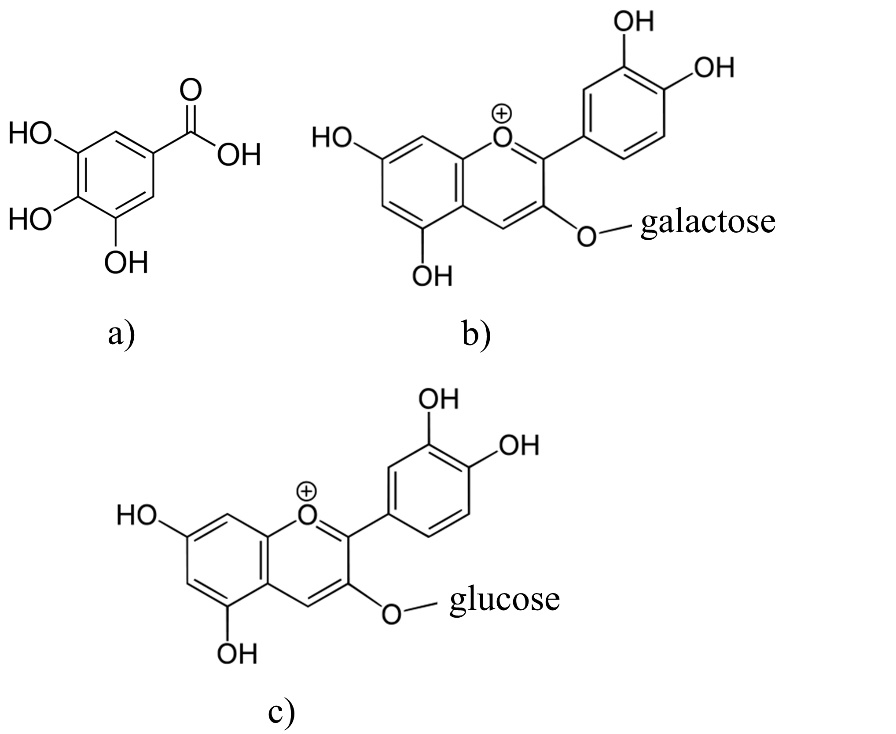
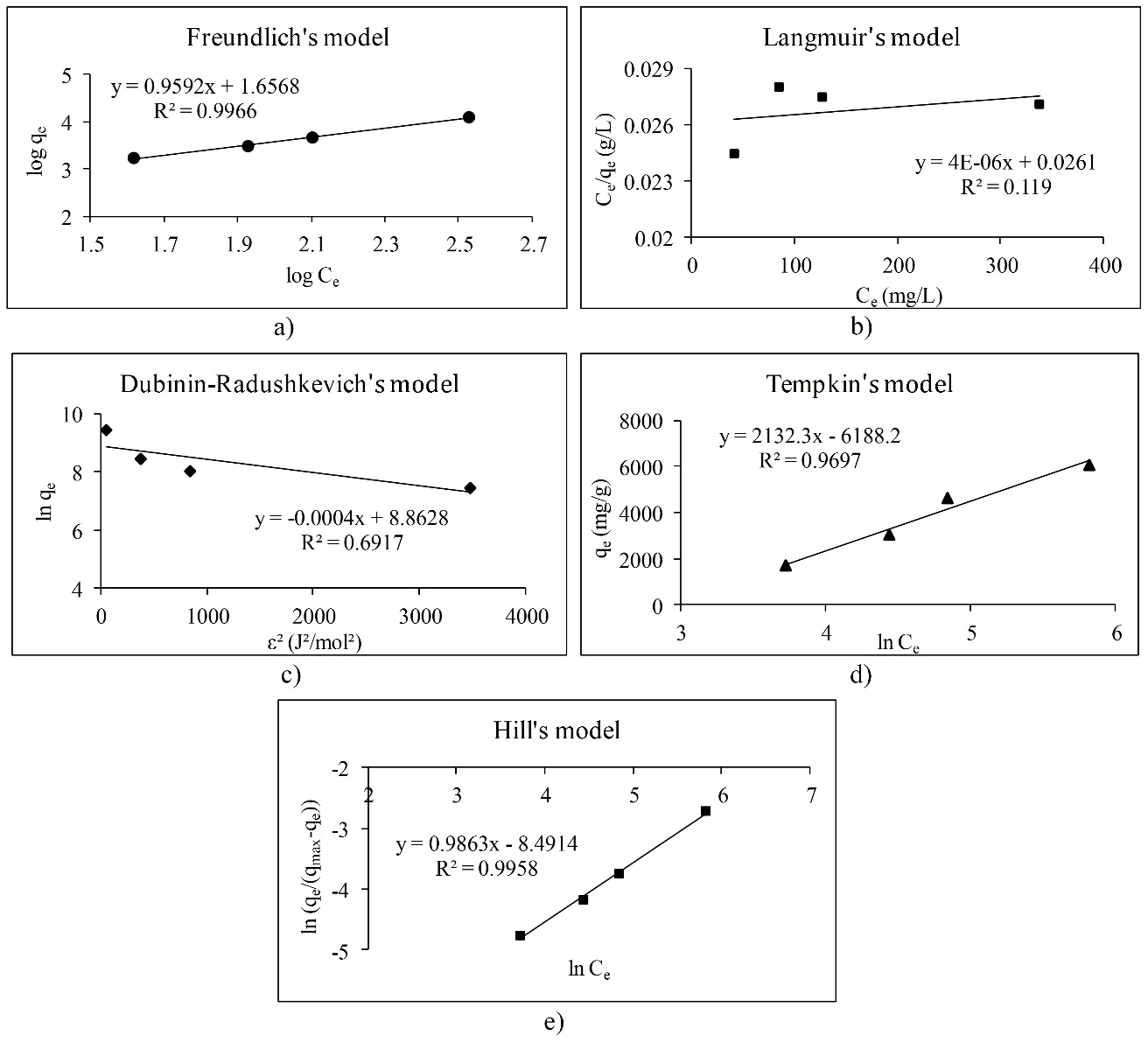
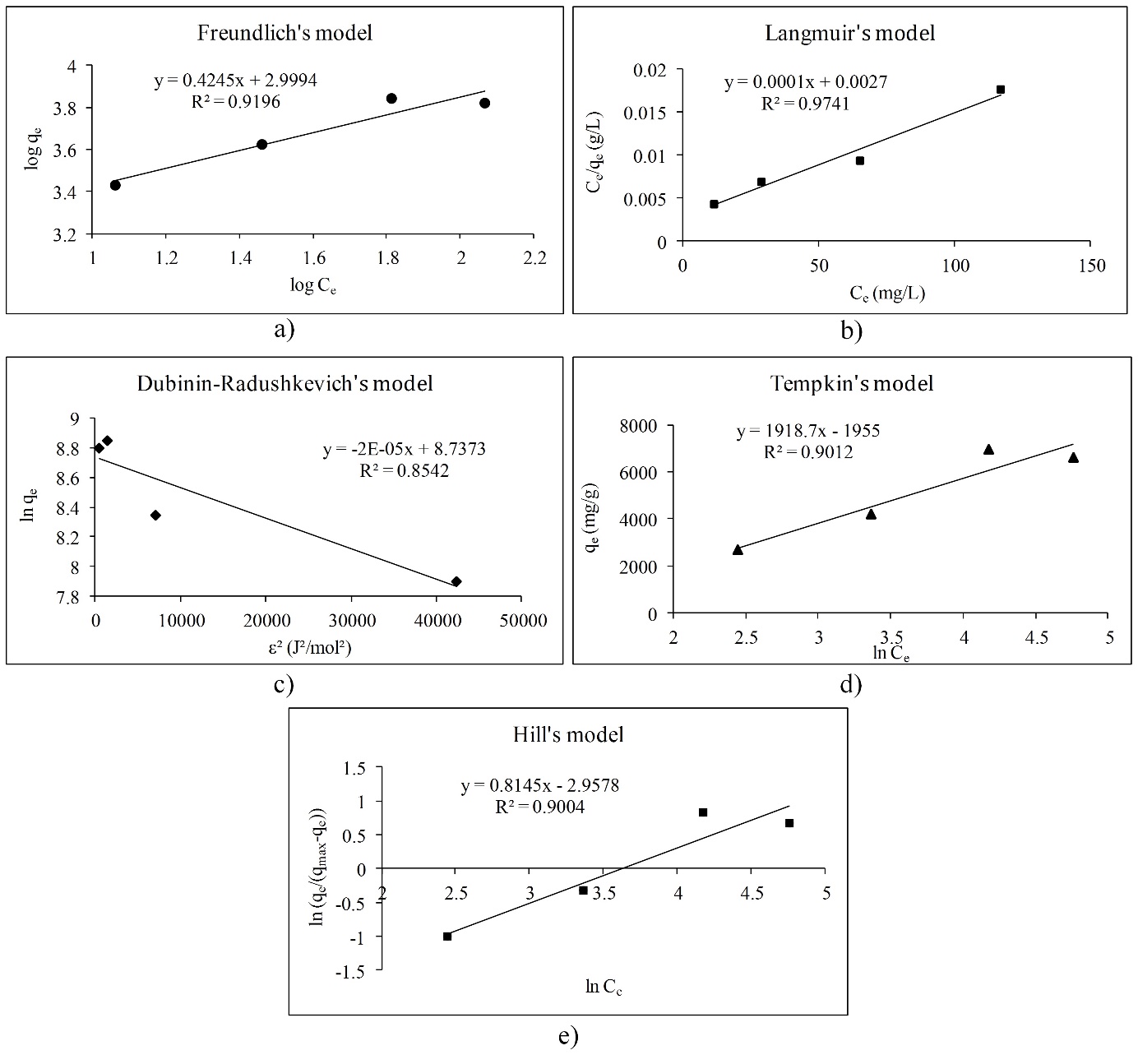
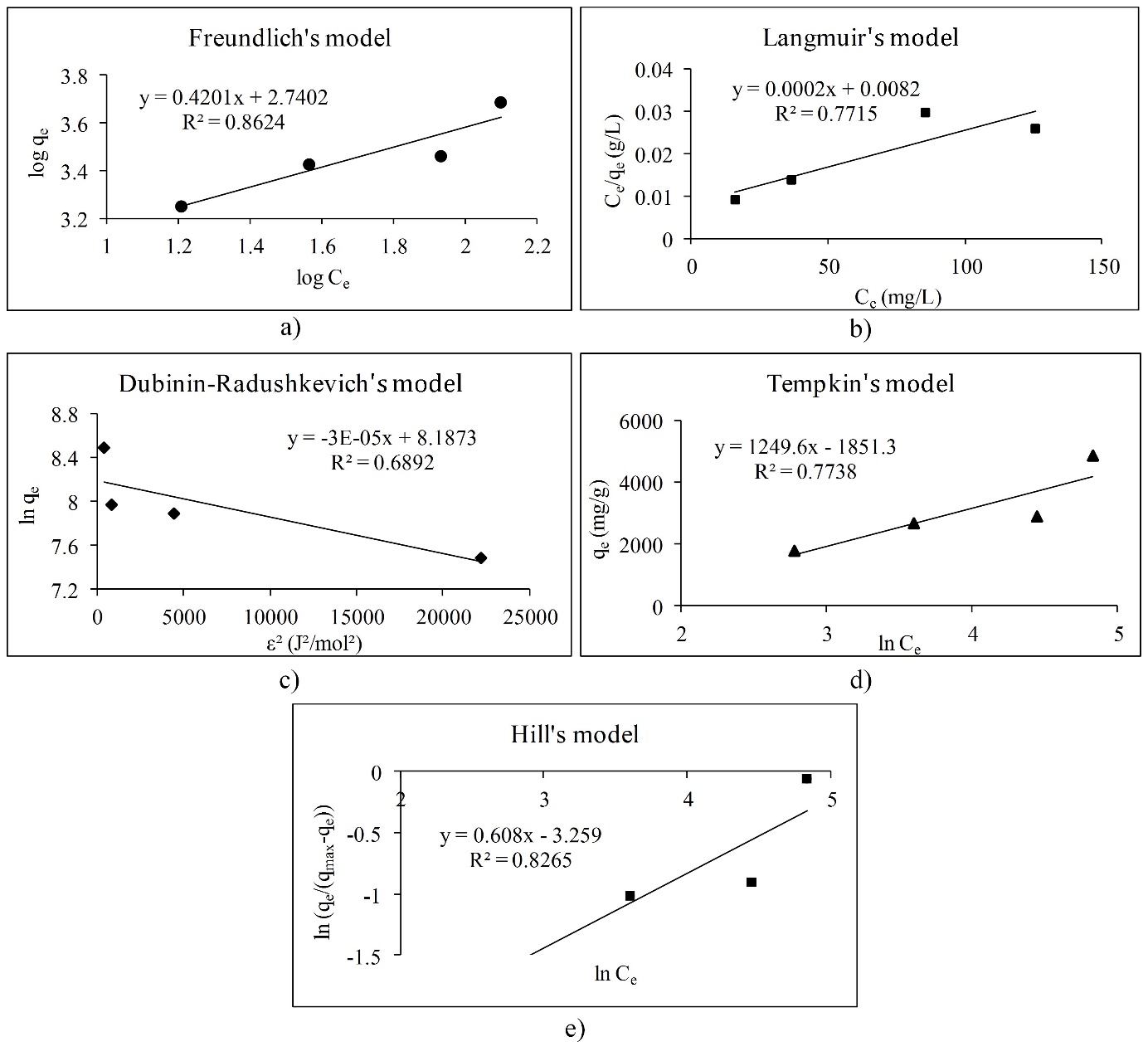
Overall, the adsorption of gallic acid and cyanidin-3-glucoside could be non-ideal, reversible and multilayer due to the Freundlich's model. Due to the slope (1/n) of linear equations for gallic acid and cyanidin-3-glucoside, surface heterogeneity is greater for gallic acid than for cyanidin-3-glucoside because of the slope of 0.9592 (Foo et al., 2010;Marsal et al., 2012). Cyanidin-3-galactoside adsorption onto β-glucan could be a monolayer adsorption where all sites possess equal affinity for the adsorbate (Foo et al., 2010).Table 2 represents adsorption isotherm parameters of Freundlich's and Langmuir's models. The parameters of the Freundlich's model indicate that the adsorption of all polyphenols onto β-glucan is favored because n is higher than 1 for all polyphenols, especially for anthocyanins. The Freundlich's constant Kf presents the value of the adsorption capacity qe at an equilibrium concentration Ce of 1 mg/L (Marsal et al., 2012). Due to that, the highest adsorption capacity for equilibrium concentration of 1 mg/L showed cyanidin-3-galactoside, followed by cyanidin-3-glucoside and gallic acid. From the Langmuir's model it can be seen that gallic acid showed the highest maximum theoretical adsorption capacity (qm), followed by cyanidin-3-galactoside and cyanidin-3-glucoside.Table 3 presents the adsorption isotherm parameters of the Dubinin-Radushkevich's, Tempkin's and Hill's models. Adsorption mean free energy E indicates that all polyphenols are physically attached to β-glucan because mean free energy is less than 8 kJ/mol (Marsal et al., 2012). Earlier studies (Quiros-Sauceda et al., 2014;Bordenave et al., 2014) also found that polyphenols can interact with polysaccharides in general by the hydrogen bonds between hydroxyl groups of polyphenols and an oxygen atom of the glycosidic linkages of polysaccharides, then with hydrophobic interactions. Furthermore, dietary fibers in particular, and polyphenols can interact by hydrogen bonds, covalent interactions or physicochemical entrapment during the gastrointestinal digestion (Quiros-Sauceda et al., 2014;Bordenave et al., 2014). This agrees with our study. The theoretical saturation capacity qm (Table 3) is higher for gallic acid than for cyanidin-3-galactoside and cyanidin-3-glucoside. The Tempkin's model also showed that adsorption of all polyphenols onto β-glucan is physical. Due to the Tempkin isotherm equilibrium constant A, which presents Tempkin's adsorption potential, cyanidin-3-galactoside showed the highest potential, followed by cyanidin-3-glucoside and gallic acid. Furthermore, Hill cooperativity coefficient of the binding interaction (nH) is lower than 1, which indicates that all polyphenols showed negative cooperativity in binding (Ringot et al., 2007).
aIndicates the heterogeneity of adsorbent surface
bIndicates the intensity of adsorption
cIndicates the value of adsorption capacity for equilibrium concentration of 1 mg/L
dConstant related to the free energy of adsorption
eMaximum adsorption capacity
aAdsorption mean free energy-indicates the physical or chemical adsorption
bTheoretical adsorption capacity
cIndicates Tempkin adsorption potential
dTempkin isotherm constant-indicates the physical or chemical adsorption
eIndicates the positive, negative or noncooperative binding interactions
fHill constant
Conclusions
The results of linear adsorption parameters showed that adsorption between all polyphenols was favored, especially for cyanidin-3-glucoside, followed by cyanidin-3-galactoside and gallic acid. All polyphenols were physically bonded onto β-glucan, where they showed negative cooperativity in binding. Gallic acid showed the highest theoretical adsorption capacity, followed by cyanidin-3-galactoside and cyanidin-3-glucoside. Gallic acid and cyanidin-3-glucoside showed that adsorption onto β-glucan was multilayer, non-ideal and reversible, while cyanidin-3-galactoside showed the monolayer adsorption. This study showed that information about interactions between polyphenols and dietary fibers like β-glucan can be obtained through parameters of linear adsorption models. Also, this information about polyphenol-polysaccharide interactions could help in understanding the bioavailability of polyphenols associated with dietary fibers.